1 Introduction
To understand pedogenesis, studies classically rely on the interpretation of the distribution of the major and minor elements and of the mineralogical phases that constitute the different soil horizons. Since these elements are localized in a wide variety of mineral phases, their mobilization is not specific to a given pedogenetic process, hence the difficulties encountered to infer and quantify the role of these different processes on pedogenesis. In addition, minor elements can originate from both natural and anthropogenic sources, which render their use as tracers of pedogenetic processes less straightforward. Rare earth elements (REE), that is, the 14 natural elements of the lanthanide series (La to Lu), have a close, but however, distinct geochemical behavior in natural systems, according to the individual REE or the group of REE under consideration [24]. Due to their properties, REE have already been extensively used as tracers of genesis and of origin or of various geochemical processes in several disciplines like hydrology [31,38], geochemistry [26] or geology [5,33]. The results of such studies let us suppose that the physical and chemical behaviors of these elements could be rather specific to a given pedogenetic process and that REE could be efficiently combined with the classical study of major elements and mineral phases to trace pedogenetic processes. However, whereas REE in soils mainly arise from parent materials by opposition to anthropogenic sources [25], up to now, the potential of REE as tracers of pedogenetic processes remains almost unexploited and the few works existing in soil science mainly deal with the geochemical fate of REE, but rarely with their pedological behavior (see, however, [1,9,12,18,19]). In addition, considering their wide ranges of concentrations and their close behavior in natural systems, REE concentrations in the studied material are normalized with respect to a chosen reference material to be compared [24] and in the case of pedological studies, the geological bedrock is usually chosen as reference for the normalization of all horizons (e.g., [1,12,18,19]). Since soil horizons result from the action of several pedogenetic processes, this way of normalization does not allow one to differentiate the impact of the successive processes on REE mobilization.
This study presents an innovative methodology to use REE normalizations, which aims at separating the signal of a pedogenetic process from the others in order to discern their respective impact on REE mobilization and fractionation. Since pedological evolution results from successive transformation fronts of different pedogenetic processes [7,22,32], the differentiation of pedological features are mainly expressed behind the transformation front of the process creating them and before the following front, that is, more or less in a given horizon. Thus, normalizing the REE concentrations of the pedological feature to the REE concentrations of the material from which it developed should allow one to differentiate the impact of the pedogenetic process involved. This approach is only possible if we have previously determined:
- • which pedological feature is the most representative of the action of each pedogenetic process;
- • which horizon is the most representative of each pedogenetic process;
- • what is the material from which the pedological feature developed.
In the present study, this methodology has been applied to a solum that displays [3]:
- • a pedogenesis sufficiently expressed, so that REE fractionations are strongly pronounced, hence an old solum;
- • high REE concentrations to facilitate the analyses;
- • a well-known succession of pedogenetic processes.
2 Material and methods
2.1 Pedological context
The chosen solum, at the northeastern margin of Morvan Mountain (Bourgogne, France), consists in the nontruncated end-member of a soil catena. It developed in the clay material resulting from the autochthonous decarbonatation of Sinemurian and Lotharingian aged-limestones that consist in hard and compact crystalline calcite. These limestones display high REE contents following mineralization events during the Lias epoch [3]. The solum then underwent successive pedogenetic processes, namely succession of oxidative and reductive conditions and eluviation [3]. It is composed of six horizons A, E, Bgd, BP, Bc and C (Fig. 1), each one being considered as more representative of a given pedogenetic process (Table 1; [29]).

Pedogenesis of the studied solum based on the vertical transformation fronts of the successive pedogenetic processes. Processes identified by Baize and Chrétien [3] and Laveuf et al. [29].
Pédogenèse du solum étudié basée sur les fronts de transformation verticaux des processus pédogénétiques successifs. Les processus ont été identifiés lors d’études antérieures[3,29].
Pedogenetic processes, with their location in the solum horizons and the derived pedological features
Processus pédogénétiques, avec leur localisation dans le solum et les traits pédologiques dérivés
Pedogenetic process | Horizon of the considered transformation front | Pedological feature derived | Normalization proposed (fraction studied /material from which it developed) |
Decarbonatation | C | Residue of decarbonatation | Residue of decarbonatation /parent limestone |
Primary redox | Bc | Fe–Mn concretions | Fe–Mn concretions/bulk fraction of the C-horizon |
Secondary redox | Bgd | Fraction < 2 μm without Fe/Mn-(hydr)oxides | Residue of extraction/fraction < 2 μm |
Eluviation | A and E | Fraction < 2 μm | Fraction < 2 μm/fraction < 2 μm of the Bgd-horizon |
2.2 Pedogenetic processes and pedological features
For complete description of the soil profile and pedological processes, refer to Laveuf et al. [29]. We summarize here the main features.
In the C-horizon, not completely decarbonated, the dominant process is decarbonatation. To study this process, we experimentally decarbonated parent limestones (see below). The residues of decarbonatation were used as pedological features of this process instead of the C-horizon, as we could not find a horizon equivalent to the C one for the Lotharingian-aged limestone.
In the Bc-horizon, the dominant process is the occurrence of seasonal variations of redox conditions in the zone of water-table fluctuations, leading to the concentration and individualization of Fe/Mn-(hydr)oxides as Fe–Mn concretions [3,29]. This process is called hereafter primary redox conditions.
The occurrence of the BP-horizon (horizon rich in phosphate nodules) results from the inheritance of a bed of pluricentimetric phosphate nodules at the bottom of the Lotharingian-aged limestone [3,34]. Indeed, a precedent investigation on Zn behavior indicated that pedogenesis does not greatly influence the mineralogical composition of the limestone–phosphate nodules [29]. In this study, the inheritance of the REE composition will be checked by comparing the REE patterns of the BP-horizon and of the limestone–phosphate nodules. The horizons underlying the BP-horizon are considered as deriving from the autochthonous weathering of the Sinemurian-aged limestone, while the others are considered as deriving from the autochthonous weathering of the Lotharingian-aged limestone [3].
In the Bgd-horizon, the dominant process is the occurrence of unfavorable redox conditions for the stability of dispersed Fe/Mn-(hydr)oxides, leading to the dissolution of these cements of finest particle-size fractions [29]. This process is called hereafter secondary redox conditions.
In the A- and E-horizons, the dominant process is eluviation [29]. This process leads to the translocation of fines particles (mainly phyllosilicates in this solum) after a more or less complete dissolution of the cementing Fe/Mn-(hydr)oxides and a sufficient desaturation of the absorbing complex [6].
2.3 Samplings and experiments
All horizons and both parent limestones were sampled.
Blocks of unweathered limestone samples without phosphate nodules and limestone–phosphate nodules were isolated from the bulk samples. Their carbonates were dissolved as follow. About 10 g of roughly grounded rock were put in closed batches with 30 mL of MilliQ® water and placed onto a magnetic agitator at room temperature. The pH was monitored along the experiment. A solution of HCl 1M was introduced drop by drop, regulating the flow so as the pH remained greater than 5 to avoid partial or total dissolution of Fe/Mn-(hydr)oxides or phyllosilicates.
The Fe–Mn concretions of the Bc-horizons larger than a 1 mm were handpicked and cleaned. For each horizon, the less than 2 μm particle-size fraction was separated from the bulk soil by sedimentation in deionized water according to the Stockes law, after a first wet-sieve at 50 μm in deionized water. An eight-steps extraction procedure, adapted from Hall et al. [23] and Cornu et al. [16], was performed on 1 g of the bulk sample ground to 50 μm for the A-, E-, Bgd- and C-horizons and of the less than 50 μm fraction for the Bc-horizon, as described by Laveuf et al. [29]. Chemical and mineralogical controls were performed during sequential extractions to verify the correct dissolution of the minerals and the recovery rates. All controls were found to be acceptable and are available in Laveuf et al. [29].
All different limestone and soil fractions were analyzed for REE by ICP-MS after LiBO2 fusion at the “Service d’analyse des roches et des minéraux of the centre de recherche pétrologique et géochimique” of the CNRS, Vandoeuvre-lès-Nancy, France (SARM-CRPG).
3 REE pattern of the parent limestones, the limestone–phosphate nodules and the bulk soil fraction of the different horizons
The REE patterns of the Lotharingian- and Sinemurian-aged limestones are similar (Fig. 2). Both limestones are slightly depleted in light REE (LREE, La to Pr) and heavy REE (HREE, Ho to Lu), and slightly enriched in medium REE (MREE, Nd to Dy) compared to the upper continental crust (UCC; [40]). This enrichment is characteristic of the Lias epoch [30,37].
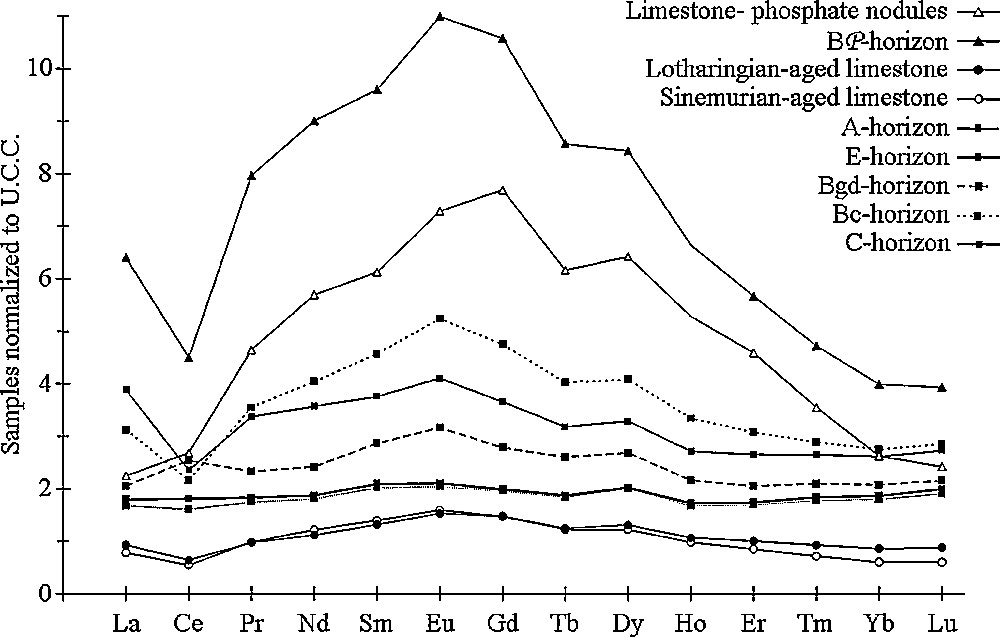
REE patterns of parent materials (limestone–phosphate nodules and Lotharingian- and Sinemurian-aged limestones) and of the soil horizons normalized to the upper continental crust (UCC).
Spectres de terres rares des matériaux parentaux (nodules phosphatés du calcaire et calcaires d’âge Lotharingien et Sinémurien) et des horizons du solum normalisés à la croûte continentale supérieure (UCC).
The limestones phosphate nodules are enriched in all REE, notably in MREE, compared to the UCC (Fig. 2). This enrichment of the phosphate nodules, mainly composed of fluorapatite [29], is related to the strong affinity of REE for phosphated compounds [24]. Experimental decarbonatation of the limestone–phosphate nodules influenced slightly their composition, since their REE pattern remained flat and no REE losses could be recorded (Fig. 3). As the REE stocks released into solution during experimental decarbonatation are assumed representative of those released into soil solution during pedogenetic decarbonatation, these results indicate that pedogenetic decarbonatation does not affect the REE composition of the limestone–phosphate nodules. The other pedogenetic processes do not influence their REE composition greatly, since the REE pattern of the BP-horizon, mainly composed of phosphate nodules, is close to that of the limestone–phosphate nodules (Fig. 2). These results confirm the inheritance of the phosphate nodules [29] and justify that the BP-horizon, mainly inherited, is not taken into account in the rest of this paper.

REE patterns of the decarbonated geological parent materials (limestone–phosphate nodules and Lotharingian- and Sinemurian-aged limestones) normalized to their corresponding carbonated parent materials. The pie charts depict the stocks of LREE (La to Pr), MREE (Nd to Dy) and HREE (Ho to Lu) of the different geological materials and the striped zones represent the average percentage of REE released into solution during the experiment of decarbonatation.
Spectres de terres rares dans les matériaux parentaux décarbonatés normalisés aux matériaux parentaux correspondants. Les graphiques à secteurs représentent les stocks de TR légères (La à Pr), moyennes (Nd à Dy) et lourdes (Ho à Lu) des différents matériaux parentaux et les zones hachurées indiquent le pourcentage moyen de TR libéré en solution au cours de l’expérience de décarbonatation.
The soil horizons are enriched in REE compared to the UCC (Fig. 2) and to the corresponding parent limestones (Fig. 4). The REE patterns of the soil horizons normalized to the UCC discriminate three groups of soil horizons with contrasted REE signatures, A and E, Bgd and Bc and C (Fig. 2). Thus, the contrasted REE signatures of the different horizons normalized to their corresponding parent limestones result from pedogenesis and not from the change of reference between surface and deep horizons (Fig. 4). If all soil horizons are REE enriched compared to the parent limestone, this enrichment decreases when going from the deepest C-horizon toward the surface (A- and E-horizons). This evolution is likely due to pedogenesis, since this process, much marked in the surface horizons than in the deepest ones, is known to induce a depletion in all REE (see e.g., [2,27,28]) and consists in the first difference in REE signature between the different horizons. The second difference in REE signature among them is related to a preferential retention of Ce in the A- to Bc-horizons, which exhibit a large positive Ce anomaly. Considering the well-known redox sensibility of Ce, this difference between the different horizons likely reflects some pedogenetic processes connected to variations in redox conditions. These results indicate that several pedogenetic processes can modify the REE distribution, which emphasizes the need for a new approach, allowing a distinction between the respective impact of these successive pedogenetic processes on REE mobilization. This approach is described in the following sections.

REE patterns of the bulk-soil fraction of each horizon normalized to the corresponding parent limestones. The A- to BP-horizons are issued from the weathering of the Lotharingian-aged limestone, while the Bc- and C-horizons are issued from the weathering of the Sinemurian-aged limestone.
Spectres de concentration des terres rares dans la fraction totale de chaque horizon normalisés au calcaire parental correspondant. Les horizons A à BP sont issus de l’altération du calcaire d’âge Lotharingien, tandis que les horizons Bc et C sont issus de l’altération du calcaire d’âge Sinémurien.
4 Impact of the successive pedogenetic processes on REE mobilization and fractionation
To differentiate the impact of the successive pedogenetic processes on REE mobilization and fractionation (Fig. 1), the main pedological features representing the main pedogenetic processes were normalized as proposed in Table 1.
4.1 Decarbonatation
For both limestones, decarbonatation induces REE enrichments in the residues (Fig. 3). The Lotharingian- and the Sinemurian-aged limestones are mainly composed of calcite (87.2 and 88.0%, respectively). During decarbonatation, REE are enriched by the preferential dissolution of calcium carbonate that leads to a leaching of Ca and a residual enrichment of less mobile elements (Table 2). However, the two limestones displayed contrasted behaviors during the decarbonatation experiment, with a loss of REE ranging from 25 to 75% of the REE stocks, depending on the limestone and group of REE under consideration (Fig. 3). Indeed, the REE loss was higher for the Lotharingian-aged limestone than for the Sinemurian-aged limestone and the Lotharingian-aged limestone preferentially lost MREE, while the Sinemurian-aged limestone preferentially lost LREE and HREE.
Concentrations of major elements (in g/kg) in the bulk and the residues of the Lotharingian- and Sinemurian-aged limestones, with corresponding initial stock losses (in percentage) during experimental decarbonatation
Concentrations des éléments majeurs (en g/kg) des échantillons bruts et des résidus des calcaires d’âge Lotharingien et Sinémurien, avec les pertes correspondantes du stock initial (en pourcentage) durant la décarbonatation expérimentale
Al | Ca | Fe | K | Mg | Mn | P | Si | Ti | ||
g/kg | ||||||||||
Lotharingian-aged limestone | Bulk | 9.3 | 333.4 | 23.7 | 4.1 | 2.8 | 1.9 | 4.0 | 26.8 | 0.7 |
Residue | 65.9 | 14.4 | 164.0 | 28.4 | 7.3 | 0.9 | 14.4 | 182.6 | 5.1 | |
% lost | 9 | 99 | 11 | 12 | 67 | 94 | 53 | 13 | 13 | |
Sinemurian-aged limestone | Bulk | 8.3 | 352.5 | 10.3 | 3.1 | 4.3 | 2.3 | 5.6 | 21.9 | 0.5 |
Residue | 59.3 | 91.7 | 81.4 | 21.3 | 6.2 | 0.4 | 44.6 | 162.8 | 3.9 | |
% lost | 17 | 97 | 8 | 20 | 83 | 98 | 8 | 13 | 10 |
These differences in the geochemical behavior of REE between the two limestones may arise from differences in the REE-bearing minerals, as evidenced by their composition in major elements (Table 2). By comparing the stock losses of major elements during decarbonatation, it appears that Al, K, Mg and P are not lost similarly in both limestones, while Ca, Fe, Mn, Si, Ti are about (Table 2). Indeed, the Lotharingian-aged limestone lost more P and less Al, K and Mg than the Sinemurian-aged limestone. The preferential loss of MREE in the Lotharingian-aged limestone may thus be related to a lower proportion of MREE in the residual minerals [11] or to the occurrence of P-bearing minerals [24]. However, it is not due to a potential dissolution of some phosphate nodules, which are noteworthy MREE bearers [41], since the decarbonatation experiment of phosphate nodules does not induce REE losses or fractionations (Fig. 3). The preferential loss of HREE from the Sinemurian-aged limestone is in agreement with the literature, since, in carbonated environments, the HREE are preferentially complexed by carbonate ions and carried away [14]. The preferential loss of LREE and HREE in the Sinemurian-aged limestone may also be due to the occurrence of sulfated compounds, as evidenced by Laveuf et al. [29] or of Al–Mg–K-bearing minerals. However, these minerals could not be identified at the moment and further analyses are in progress to reach this goal.
Note that to study the fate of REE, we must not consider only the values of the normalized concentrations, which show an enrichment of the decarbonatation residues compared to the parent limestones, but also the evolution of REE stocks (see pie charts in Fig. 3), which show important REE losses during decarbonatation. This statement is crucial when the pedogenetic process induces a high variation of volume as demonstrated for other elements [21].
4.2 Redox conditions
4.2.1 Primary redox conditions
As evidenced by Laveuf et al. [29], parent limestones are free of ferruginous Fe oolithes, which indicates that Fe–Mn concretions are of pedogenetic origin [3]. Seasonal variations of redox conditions in the zone of water table fluctuations lead to the formation of Fe–Mn concretions that are particularly abundant and well individualized in this concretion-rich Bc-horizon [3,29]. In the Fe–Mn concretions of the Bc-horizon, all REE are one to two times enriched compared to the bulk fraction of the C-horizon, except Ce, which is up to 3.5 times enriched (Fig. 5). This strong positive Ce anomaly in the pedogenetic Fe–Mn concretions is likely related to the occurrence of Fe/Mn-(hydr)oxides in these concretions. During primary redox conditions, the fraction of Ce3+ released upon the dissolution of primary Ce-bearing minerals can be oxidized to Ce4+ by secondary Fe/Mn-(hydr)oxides. Once oxidized, Ce is much less mobile because of the stronger sorption of Ce4+ onto Fe/Mn-(hydr)oxides [13,27,35] or its possible precipitation as cerianite (CeO2) [4,8,20]. This very low mobility of Ce4+ in soil profiles – when Fe/Mn-(hydr)oxides capable of oxidizing Ce3+ to Ce4+ occur – can then lead to the appearance of a positive Ce anomaly in REE patterns [24,41]. A strong geochemical relationship between Ce and Mn in earth-surface environments has been evidenced by several studies [13,27,35,39], notably cerianite with Mn oxides [8], likely because of the close redox behaviors of Ce and Mn [10]. As concentrations of Ce and Mn in the Fe–Mn concretions are more much enriched than for Fe (Table 3), it may indicate an association of Ce with Mn oxides.

REE patterns of the Fe–Mn concretions of the Bc-horizon for different depths normalized to the bulk fraction of the C-horizon.
Spectres de terres rares des concrétions Fe–Mn de l’horizon Bc pour différentes profondeurs normalizes, à la fraction totale de l’horizon C.
Iron, manganese and cerium concentrations of the Fe–Mn concretions, of the less than 50 μm fraction of the Bc-horizon (115–125 cm) and of the bulk fraction of the C-horizon from which the Bc-horizon was developed
Concentrations en fer, manganèse et cérium des concrétions Fe–Mn, de la fraction inférieure à 50 μm de l’horizon Bc (115–125 cm) et de la fraction totale de l’horizon C au dépend de laquelle l’horizon Bc s’est développé
Fe | Mn | Ce | ||
g/kg | g/kg | mg/kg | ||
Bc-horizon (115–125 cm) | Fe–Mn concretions | 151 | 68 | 538.0 |
< 50 μm fraction | 75 | 6.1 | 96.3 | |
C-horizon | 90 | 8.0 | 150.3 |
4.2.2 Secondary redox conditions
Seasonal variations of redox conditions in the Bgd-horizon lead to the dissolution of dispersed Fe/Mn-(hydr)oxides that cement the finest fractions [29]. To study the impact of such redox processes on REE fractionation, we studied the less than 2 μm fraction normalized to the residue of extraction [29]. Indeed, the less than 2 μm particle-size fraction consists of Fe and Mn oxihydroxides, of phyllosilicates and of quartz. The residue of extraction consists of phyllosilicates and quartz. As REE do not bind to quartz, they are therefore bound to the phyllosilicates and to the Fe/Mn-(hydr)oxides. Therefore, the normalization of the less than 2 μm particle-size fraction to the residue of extraction allows to depict the REE pattern of the Fe/Mn-(hydr)oxides, since these minerals are totally absent in the residue of extraction and since phyllosilicates occur in both samples. Globally, the less than 2 μm fractions are enriched in MREE compared to the residues of extraction (Fig. 6), which indicates that Fe/Mn-(hydr)oxides are enriched in MREE. Consequently, the more the horizons will be affected by secondary redox conditions, the less the MREE will be enriched in the less than 2 μm fractions compared to the residues of extraction. This assumption is supported by the known MREE enrichment of colloidal Fe particles in soil waters [17,20]. That is why, along the studied solum, MREE enrichment decreases toward soil surface. This global trend also confirms our pedological observations, that is, that the Bc- and C-horizons are not affected by secondary redox processes, on the opposite to the A-, E- and Bgd-horizons.
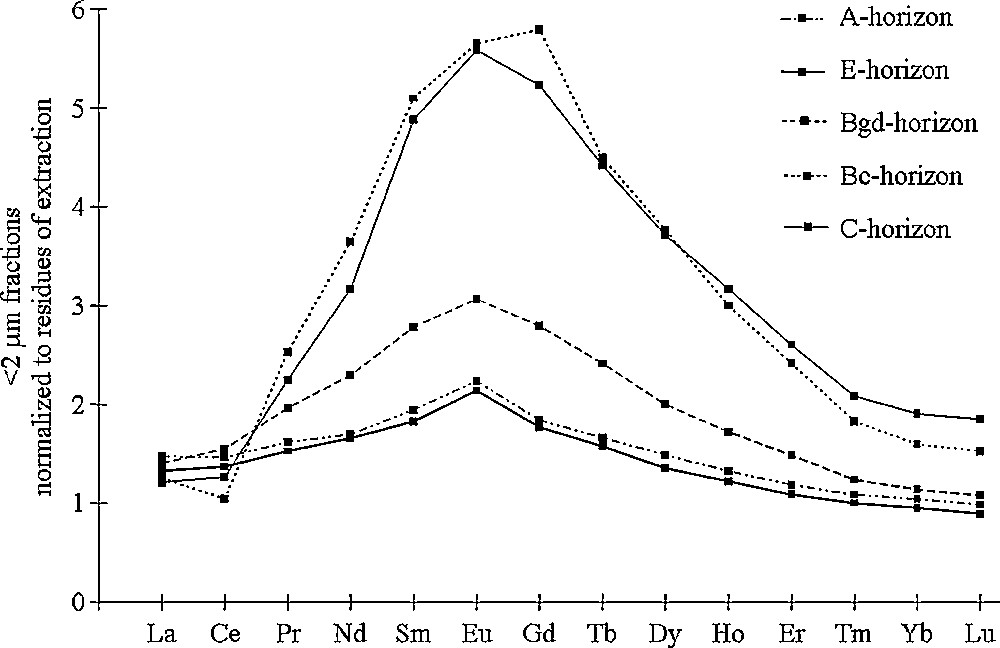
REE patterns of the less than 2 μm particle-size fractions of each horizon normalized to the residue of extractions of the corresponding bulk-soil fractions.
Spectres de terres rares des fractions granulométriques inférieures à 2 μm de chaque horizon normalisés au résidu d’extraction de la fraction totale correspondante.
4.3 Eluviation
Eluviation is a physical process, involving the translocation of fine particles, mainly phyllosilicates. Since this process is only physical, it should not fractionate REE within the less than 2 μm fraction, as the whole fraction is supposed to be removed. However, the normalization of the less than 2 μm fraction of the A- and E-horizons to that of the Bgd-horizon, which is neither eluviated nor significantly illuviated, evidences that eluviation fractionates REE in these soil horizons (Fig. 7). All REE, and particularly MREE, are depleted in the less than 2 μm fraction of the A- and E- horizons compared to that of the Bgd-horizon, except Ce which exhibits a positive anomaly. The positive Ce anomaly is likely due to the fact that Ce is not bound to eluviated phyllosilicates and is thus not affected by this pedogenetic process. This hypothesis has to be confirmed, notably by the finding of the Ce-bearing phases. The larger loss of MREE, compared to the other REE, can be due to a preferential association of MREE with phyllosilicates. However, this hypothesis is in contradiction with Cao et al. [11], who showed with chemical extractions that MREE have lower proportions of residual form than LREE and HREE. Another explanation could be the preferential eluviation of the smallest phyllosilicates (smectite, illite, vermiculites; [36]), since they do not exhibit the same REE compositions as the biggest ones (kaolinite and chlorite; [14,15,42]). Finally, the integration of another pedogenetic process because of an insufficient segregation of the REE pattern of the phyllosilicates through our normalization could also explain the observed differences.

REE patterns of the less than 2 μm fraction of the A- and E-horizons normalized to the less than 2 μm fraction of the Bgd-horizon.
Spectres de terres rares dans la fraction inférieure à 2 μm des horizons A et E, normalisés à la fraction inférieure à 2 μm de l’horizon Bgd.
5 Conclusion
Despite some remaining difficulties and uncertainties, the first results obtained in this study indicate that the proposed REE normalization approach is informative and that it could be used to exploit the potential of REE as tracers of pedogenetic processes. Concerning redox conditions, results obtained on our selected solum demonstrate that the two stages of redox conditions identified acted differently on the Fe/Mn-(hydr)oxides and associated REE. Primary redox conditions lead to the formation of Fe–Mn concretions in which all REE are enriched, but which exhibit a strong positive Ce anomaly because of preferential sorption onto Fe/Mn-(hydr)oxides. Cerium may thus be used as a useful tracer of the quantitative mobilization of Fe/Mn during primary redox conditions. Secondary redox conditions induce the dissolution of the Fe/Mn-(hydr)oxides that cement the finest particle-size fractions and the concomitant release of associated MREE. MREE may thus potentially serve as tracers of the loss of Fe/Mn-(hydr)oxides during secondary redox conditions. Concerning the role of eluviation on REE fractionation, our approach is not sufficiently constrained, probably because of a preferential translocation of some phyllosilicates with different REE compositions or an insufficient enhancement of the signature of the pedological feature resulting from eluviation through our normalization.
However, since the proposed approach is based on the normalization of the REE concentrations of a pedological feature in a given horizon to the REE concentrations of the material from which it developed, it necessitates that the pedogenetic process, related to the selected pedological feature, is dominant. This condition indicates that a more accurate identification of the pedogenetic processes related to the various possible pedological features is strongly required to better differentiate the impact of these processes on REE fractionation. For instance, quantification of the preferential translocation of some phyllosilicates with different REE compositions is fundamental to better understand the role of eluviation on REE fractionation. In addition, the REE normalization approach proposed would strongly benefit from a better characterization of the material from which the studied pedological feature has developed. For instance, actual speciations of Ce and MREE in the Fe–Mn concretions are needed to emphasize the relative role of primary and secondary redox conditions on REE fractionation.
Acknowledgments
The authors would particularly like to thank O. Josière for technical support and D. Baize for scientific comments and advices. This work has been carried out thanks to the financial support of the Projet Innovant Terres Rares of the INRA Department “Environnement et Agronomie” and of the “région Centre”. It also benefited from funding by the “GDR TRANSMET” Program of the Centre national de la recherche scientifique (CNRS) through the action “Comportement biogéochimique, minéralogique et cristallochimique des éléments métalliques et des métalloïdes à proximité des gisements et des anomalies géochimiques”.