1 Introduction
The delicate exploitation of a coastal aquifer usually induces problems of quantity and quality [13]. Indeed, a prolonged drought can be the origin of the rupture of the seawater–freshwater interface equilibrium, which in turn may cause seawater intrusion [17,18]. This can be seen through the space-time evolution of piezometric heads and the water chemical quality of these aquifers. Tunisia, like all Mediterranean countries, has experienced considerable periods of drought during the last two decades. The resulting water deficit was compensated through an increased withdrawal from aquifers. However, aquifers close to coastal areas are the most threatened as they are marked by a very strong borehole density resulting from an intense overexploitation, and shown by an increase in the mineralization of their waters [10]. This constitutes a limiting factor for their use. In this work, we will try to demonstrate seawater intrusion and its extension in the Plio-Quaternary aquifer of Korba, a Tunisian aquifer which is very heavily exploited by farmers.
The main purpose of our hydrochemical study is to determine the spatial extension of the salinization and also to identify the origins and the mechanisms governing its contamination. This will be shown through the use of the chemical contents in conjunction with the different processes, which modify the water mixture characteristics [33]. This change is due to the absence of balance between the aquifer and the water mixture. Indeed, the carbonates and clays contribute in the dissolution and the precipitation of some minerals, with cation exchange, which acts in opposition to the changes caused by the marine intrusion. These processes are the principal factors modifying the geochemistry of the water salinized by the seawater intrusion [33].
2 Geographic and geologic situation
The study area, covering 430 km2 [30], is located in northeastern Tunisia, within the eastern coastal Plain of Cap-Bon (Fig. 1). This aquifer is bounded to the south by Oued Boulidine, to the north by Oued Lebna, to the west by Jebel Abdeerahmen and to the east by the Mediterranean Sea.
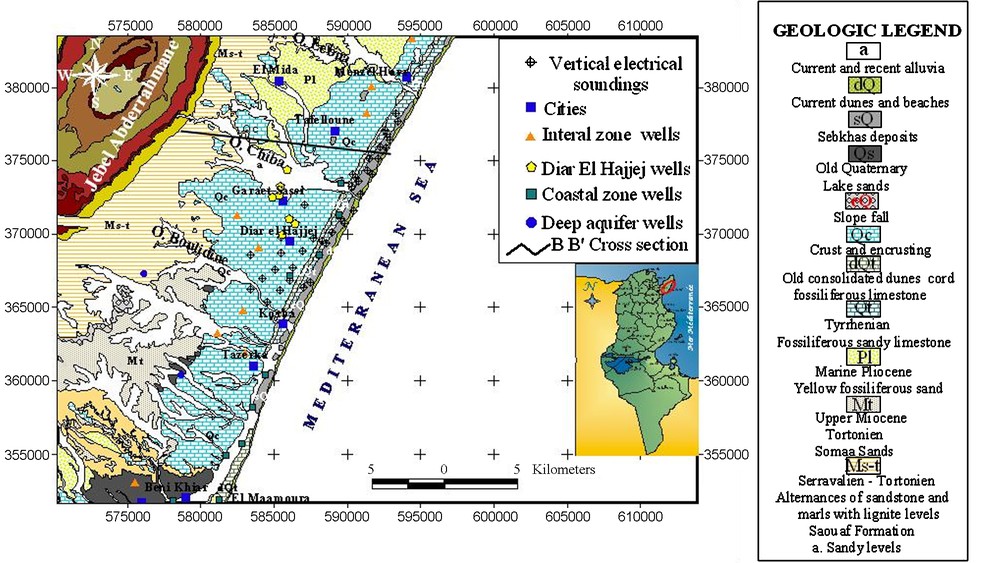
Geology and samples and VES location in the Korba aquifer (June 2006).
Fig. 1. Localisation de la géologie de l’échantillonnage et des sondages électroniques verbiaux dans l’aquifère de Korba (juin 2006).
The outcropping formations of the study area are represented by Mio-Plio-Quaternary sediments [5]. The lower part of the Middle Miocene corresponds to detrital deposits known as the Beglia Formation. The upper part is composed of lenticular sandstones and marls with lignite levels called the Saouaf Formation. In the study area, the Upper Miocene is absent. This is believed to have been caused by the widespread erosional activity due to the Miocene orogeny [14].
The trangressional marine Pliocene sediments were unconformably deposited on these folded and eroded formations. These deposits are mainly composed of sandstone-sand-marl alternations topped by sandstones and sand [11]. This facies changes laterally to argillaceous sands or to consolidated sandstones, more or less argillaceous.
The Quaternary deposits are usually composed of two units: the lower unit of marine facies corresponds to sandy limestone with molluscs indicating the maximum flooding surface (MFS) of the Tyrrhenian transgression. The upper unit is mainly composed of a continental facies [28] with the occurrence of oolitic limestone and coprolites or pelloïds. These deposits form, nowadays, coastal consolidated dunes built by wind following marine regression [7,28]. The old consolidated dunes cover the Tyrrhenian deposits [28]. The encrusted limestone extends over significant distances. They are very rich in calcite, silica, sometimes in gypsum and alumina and frequently coloured by iron salts. Finally, The Holocene deposits are formed by recent alluvia of Oued Chiba, by sebkhas deposits and current dunes and beaches.
3 Hydrogeology
The hydrogeological study of the Korba area shows that the Plio-Quaternary detrital deposits constitute a potential shallow aquifer. The marls of the Middle Miocene form the impermeable substratum of this aquifer [14] (Fig. 2).
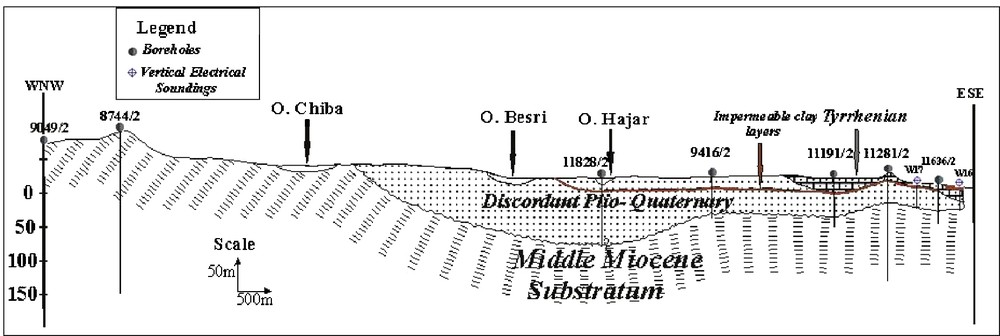
Geological cross section B–B’.
Fig. 2. Coupe géologique B–B’.
The aquifer recharge is primarily made through the carved glacis rivers having a very porous lithology. The intercommunication between the Pliocene formations and recent alluvia is absent. The Quaternary recharge is increased partly by Pliocene sediments infiltration. Part of the groundwater flow occurs within the Tyrrhenian limestone before reaching the sea. Indeed, the Tyrrhenian deposits constitute a good reservoir and a key point of coastal aquifer recharge. Meteoric water recharge of the aquifer system is thought to occur through the coastal consolidated barriers, which characterize the Tyrrhenian beach. This contribution is shown through its great infiltration capacity and its role as topographic obstacle against surface run off. This helps water infiltration downstream in case it escapes infiltration upstream [14].
The recent 2006 piezometric map (Fig. 3) shows piezometric depressions related to overexploitation of the aquifer with negative piezometric levels on the order of −12 m. This map (Fig. 3) shows a multidirectional flow mainly oriented to the piezometric depressions located at Diar El Hajjej-Garaet Sassi and to the east of Tafelloune.

Piezometric map of Korba aquifer (June 2006).
Fig. 3. Carte piézométrique de l’aquifère de Korba (juin 2006).
4 Methods
A multidisciplinary approach is needed in order to establish marine intrusion monitoring. Previous studies [3,4,9,12,19–21,23–25,29,32,33,35,36,38,41], through analytical, geophysical and modelling methods, have approached the problem and attempted to position the freshwater–seawater interface.
Other studies [1,15,20,22,26,27,34,38,39] defined the chemical processes and reactions, which characterize mineralization and would thus be responsible for groundwater chemical elements enrichment or impoverishment.
Geochemical properties were determined through different analyses, in order to characterize salinisation and the different factors monitoring its space-time evolution.
This article presents the analytical results of 28 samples, which were collected in June 2006. Twenty-six samples were collected from shallow wells and two were taken from the deep aquifer.
Electrical conductivity, temperature and pH were measured in situ. The cations (Na+, K+, Ca2+ and Mg2+) were analysed at “Laboratoire des resources minérales et environnement” of the Faculty of Sciences of Tunis using Atomic Absorption Spectrometry. Bicarbonates were determined by the volumetric method, Cl− was determined using the Mohr method and finally SO42− was, spectrophotometrically, analysed.
The interpretation of the analytical results is shown numerically and graphically through the saturation index (SI), ionic deviations, Piper Diagram and binary diagrams.
SI and ionic deviations were calculated to better understand the hydrogeochemical processes that take place in the aquifer. Calculation of the ionic deviations (Δ) corresponds to a comparison of the measured concentration of each constituent to its theoretical concentration of a known theoretical freshwater–seawater mixture calculated from the Cl concentration of the sample (see formula below) [16]:
Ci, sample is the measured concentration of the ion i in the sample, and Ci mix is the theoretical concentration of the ion i for the theoretical (conservative) mixture of freshwater–seawater. The theoretical mixture concentrations were calculated taking into account seawater contribution (fsea) based on the chloride contents in the sample (CCl, sample), the freshwater Cl concentration (CCl,f) and the seawater Cl concentration (CCl,sea):
This allows calculation of seawater contents (%) in each sample.
This seawater contribution was then used to calculate the theoretical concentration of each ion:
These calculations take into account that Cl is a conservative tracer [37]. In fact, Cl is not usually removed from the system due to its high solubility [2]. The only inputs are either from the aquifer matrix salts or from a salinization source like seawater intrusion etc.
The PHREEQC code was used to model calcite, dolomite and gypsum saturation states of the freshwater–seawater mixing process in a closed system. This code calculated the samples ionic strength and those of the theoretical mixtures between freshwater and seawater. Moreover, PHREEQC provided the geochemical composition of these theoretical mixtures.
This software is a specialized code that uses the Mass Balance and Electroneutrality equations to simulate geochemical reactions, such as mixing of waters, addition of net irreversible reactions to solution, dissolving and precipitation phases to achieve equilibrium with the aqueous phase [31]. The modelled saturations states of these minerals and the geochemical compositions of mixing waters will be compared to the measured ones. This comparison would confirm the cationic exchange processes, precipitation, and mineral dissolutions.
Prior to these geochemical analyses, a geophysical survey combined to borehole logging data was applied on the study area. In fact, there is an empirical relation between the resistivity of water and its mineralization [8,25]. This geophysical method was implemented for the aquifer geometry study and the marine intrusion evaluation. In addition, the electrical resistivity of rocks depends on many factors such as groundwater salinity, saturation, aquifer lithology and porosity.
Electrical resistivity data have been used to identify the geographical extent of salinization in the aquifer, as resistivity decreases with increasing salinity. Indeed, 38 electric surveys using the Wenner process were carried out between Korba and Oued Lebna. The outcome of this geophysical survey is given below.
5 Geo-electrical study
To evaluate the extension of the marine intrusion in the coastal aquifer of the eastern coast of Cap-Bon, 38 vertical electrical soundings (VES) using the Wenner process were carried out in the area of Korba. Lines AB of these electric surveys are directed parallel to the coast. This orientation facilitates the quantitative interpretation of these electric soundings, carried out by the Winsev software. This geophysical study aims to construct an iso-resistivity map of seawater intrusion. The calibration of the VES results with borehole data enabled us to establish geological cross sections (Fig. 2), which shed more light on the geometry of the aquifer and estimate the vertical and horizontal configuration of the seawater intrusion. Thus, the main purpose of this study is to highlight the spatial distribution of the salinization and its extension. The iso-resistivity contour map (Fig. 4) shows zones of contamination which resistivity lies between 1 to 17 Ωm. These contamination zones characterize the coastal zone and the Diar El Hajjej and Garaet Sassi areas. Some zones of relatively high resistivity located along the coast are the consequence either of the direct infiltration of rainwater through the recent littoral sandy dunes or to the rise of the substratum. This map shows the extent of the marine invasion of the area of Diar El Hajjej compared to that of Tefelloune. Given these geophysical findings, a geochemical study was undertaken and the following presents the main results and interpretations. Former studies have showed the complementarity of these two methods [6,42].

Iso-resistivity contour map.
Fig. 4. Carte des contours d’iso-résistivité.
6 Hydrochemical results and discussion
Water sampling was carried out covering the Korba-Diar El Hajjej-Garaet Sassi zone and the coastal zone. The geophysical method enabled to detect low resistivities in these areas. However, during this study, we had recourse to a sampling, which exceeds these zones. This is to know the marine intrusion space extension and to do a comparison between the geochemical phenomena observed in these areas. Two samples were taken from the deep aquifer.
Water samples collected from these areas show high chloride contents (Fig. 5).The determination of the groundwater salinity origin was based on a spatial evolution of the total dissolved salt (TDS) and the major elements contents (Table 1). The high values of the TDS of water of the aquifer relate to the zones characterized by significant piezometric depressions such as the areas of Diar El Hajjej-Garaet Sassi and Korba Tazerka.

Iso-chloride (ppm) contour map of Korba aquifer (June 2006).
Fig. 5. Carte des contours d’iso-valeur des chlorures (ppm) de l’aquifère de Korba.
Sampling Analytical results of Korba aquifer (June 2006) (ppm).
Tableau 1 Résultats analytiques des échantillons d’eau de l’aquifère de Korba (juin 2006) (ppm).
No. | Na+ | K+ | Ca2+ | Mg2+ | Cl− | HCO3− | SO42− | Error % | Mg/Ca | SO4/Cl | TDS | Ionic strength |
1 | 594 | 41 | 388 | 168 | 878 | 325 | 1557 | −2.03 | 0.71 | 1.31 | 3951 | 7.36E-02 |
2 | 302 | 60 | 198 | 87 | 457 | 343 | 568 | 2.26 | 0.73 | 0.92 | 2014 | 3.86E-02 |
3 | 440 | 18 | 296 | 133 | 1124 | 412 | 323 | 0.16 | 0.74 | 0.21 | 2746 | 5.62E-02 |
4 | 518 | 44 | 219 | 106 | 983 | 331 | 438 | 1.16 | 0.80 | 0.33 | 2639 | 5.15E-02 |
5 | 215 | 24 | 128 | 45 | 351 | 308 | 160 | 4.59 | 0.58 | 0.34 | 1231 | 2.40E-02 |
6 | 979 | 61 | 240 | 85 | 1510 | 436 | 431 | 3.57 | 0.58 | 0.21 | 3741 | 6.98E-02 |
7 | 204 | 9 | 90 | 56 | 386 | 198 | 165 | 1.58 | 1.02 | 0.32 | 1106 | 2.23E-02 |
8 | 743 | 24 | 314 | 110 | 1370 | 360 | 605 | 0.45 | 0.58 | 0.33 | 3526 | 6.81E-02 |
9 | 437 | 9 | 333 | 60 | 983 | 215 | 490 | −0.75 | 0.30 | 0.37 | 2528 | 5.07E-02 |
10 | 457 | 10 | 208 | 54 | 808 | 383 | 290 | −0.24 | 0.43 | 0.26 | 2209 | 4.19E-02 |
11 | 279 | 4 | 159 | 103 | 737 | 285 | 318 | −5.66 | 1.07 | 0.32 | 1885 | 3.77E-02 |
12 | 166 | 9 | 61 | 48 | 281 | 180 | 163 | 0.69 | 1.30 | 0.43 | 908 | 1.81E-02 |
13 | 1138 | 23 | 426 | 301 | 2458 | 360 | 872 | 1.43 | 1.16 | 0.26 | 5577 | 1.13E-01 |
14 | 279 | 212 | 179 | 90 | 457 | 459 | 502 | 4.73 | 0.83 | 0.81 | 2178 | 3.96E-02 |
15 | 391 | 20 | 216 | 109 | 843 | 325 | 316 | 2.23 | 0.83 | 0.28 | 2220 | 4.51E-02 |
16 | 259 | 16 | 266 | 127 | 597 | 354 | 478 | 4.07 | 0.79 | 0.59 | 2097 | 4.35E-02 |
17 | 849 | 0 | 346 | 128 | 1475 | 244 | 935 | −0.28 | 0.61 | 0.47 | 3977 | 7.67E-02 |
18 | 340 | 14 | 136 | 167 | 843 | 354 | 229 | 1.81 | 2.03 | 0.20 | 2081 | 4.39E-02 |
19 | 514 | 18 | 278 | 269 | 1440 | 372 | 292 | 5.41 | 1.59 | 0.15 | 3183 | 7.12E-02 |
20 | 1198 | 112 | 226 | 270 | 2845 | 459 | 57 | −0.25 | 1.97 | 0.01 | 5166 | 1.05E-01 |
21 | 402 | 15 | 149 | 143 | 913 | 343 | 219 | 1.57 | 1.58 | 0.18 | 2184 | 4.50E-02 |
22 | 337 | 10 | 173 | 187 | 878 | 354 | 203 | 5.62 | 1.78 | 0.17 | 2142 | 4.73E-02 |
23 | 333 | 12 | 271 | 201 | 913 | 267 | 466 | 5.91 | 1.22 | 0.38 | 2464 | 5.44E-02 |
24 | 560 | 27 | 470 | 193 | 1756 | 227 | 433 | 1.71 | 0.68 | 0.18 | 3666 | 8.01E-02 |
25 | 563 | 14 | 385 | 159 | 1545 | 238 | 356 | 2.00 | 0.68 | 0.17 | 3260 | 7.01E-02 |
26 | 536 | 12 | 492 | 199 | 1861 | 238 | 372 | 0.35 | 0.67 | 0.15 | 3711 | 8.21E-02 |
27 | 542 | 15 | 179 | 154 | 983 | 378 | 377 | 4.38 | 1.42 | 0.28 | 2628 | 5.30E-02 |
28 | 511 | 15 | 120 | 94 | 632 | 627 | 207 | 5.71 | 1.30 | 0.24 | 2207 | 3.59E-02 |
The Piper Diagram illustrates three chemical facies (Fig. 6): Na-Cl facies, Ca-Mg-Cl facies and SO4-mixed facies. The last one characterizes one sample of the zone of Beni Khiar. The Ca–HCO3 water type is absent. This facies characterizes the shorter residence time waters of the detritic aquifers [1].
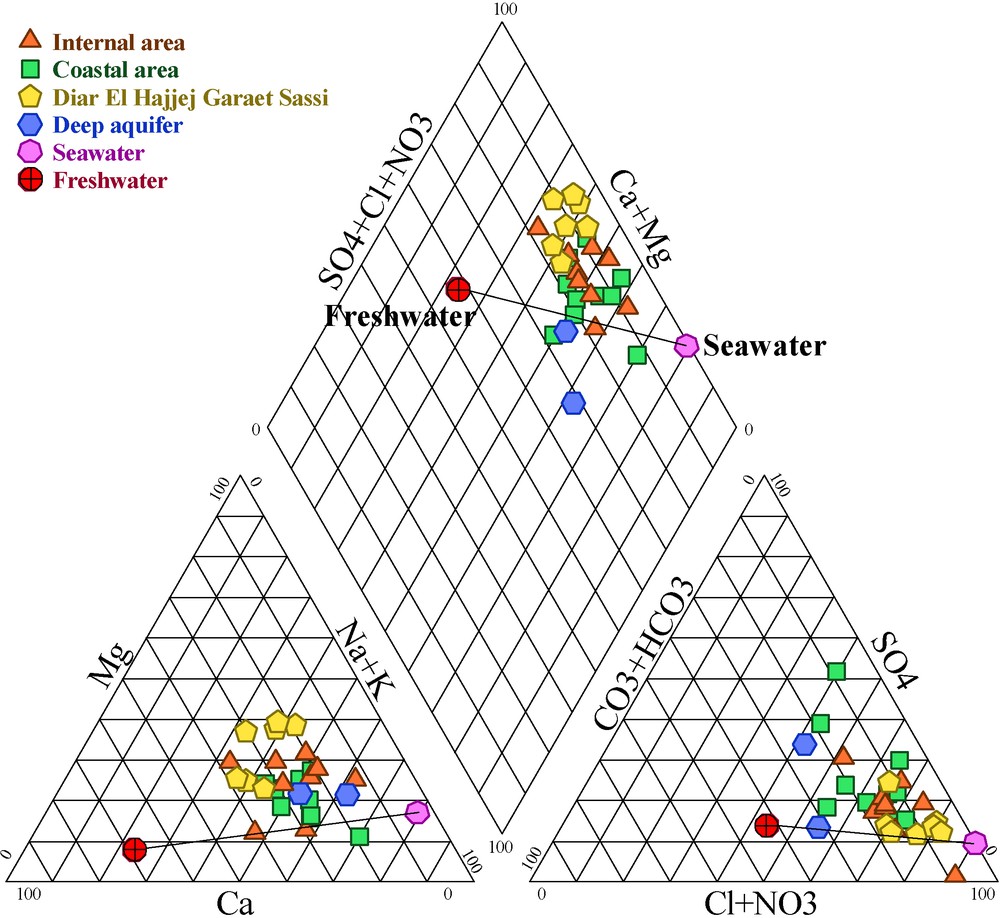
Water sampling analytical results plotted in the Piper Diagram (June 2006 survey).
Fig. 6. Représentation des résultats analytiques des échantillons d’eau dans le diagramme de Piper (juin 2006).
The calculation of seawater contents (%) presents values lower than 14% of seawater.
Calcite, dolomite and gypsum SI were also calculated using the PHREEQC 2.8 software to verify the salinization phenomena, the exchange processes, the precipitation and dissolution of some minerals, and the dolomitization processes. Gypsum SI were modelled for different mixing rates to assess the possibility of SO42− reduction or an alternative SO42− source (such as gypsum dissolution). Fig. 7 shows calcite, dolomite and gypsum saturation index versus seawater in the mixture. Also shown is the theoretical line of conservative mixing.

SI of calcite (a), gypsum (b), dolomite (c) and halite (d) of the Korba aquifer samples. SI of the conservative mixture of freshwater and seawater.
Fig. 7. Index de saturation de la calcite (a), du gypse (b), de la dolomite (c) et de l’halite (d) dans les échantillons de l’aquifère de Korba. Index de saturation du mélange conservatif eau douce/eau de mer.
The Mg/Ca ratios of the aquifer water are modelled by the PHREEQC software and calculated from the concentration of chlorides. According to the proportions of seawater, the Mg/Ca ratios modelled by the two methods have the same shape, which enhances the reliability of these methods for the calculation of theoretical concentrations.
The PHREEQC code was also used to calculate the ionic strength.
Debye-Hückel has proved to be remarkably successful in predicting activity coefficients in dilute solution. The extended Debye-Hückel Equation is most useful at concentrations less than 0.1 M, which includes many natural waters and provides adequate approximation for activity coefficients up to ionic strengths of about 1 M, which would include most solutions of geological interest, including seawater. The Davies equation is slightly more accurate in the range of 0.1 to 1 M ionic strength. Above these concentrations, both the Davies and Debye-Hückel equations are increasingly inaccurate [40]. The ionic strength values calculated lie between 1.81E-02 and 7.30E-01 (Table 1). The last value is that of seawater. This means that the use of the PHREEQC code is validated in the case of the explored salinity ranges.
6.1 Diar El Hajjej and Garaet Sassi
The relationship between Na+ and Cl− (Fig. 8a) demonstrates low Na+ contents in these samples. In fact, the Diar El Hajjej Garaet Sassi Korba samples are positioned lower than the theoretical mixing freshwater–seawater line. These samples are especially controlled by an inverse cation exchange between Na and Ca-Mg. This behaviour is documented by a majority of samples enriched in Ca2+ and/or Mg2+ with respect to the theoretical mixture (Fig. 8b and c). Divergences from the lines could be attributed to an ion exchange phenomenon. In fact, many samples present an excess of calcium and/or Mg2+ and a deficit of sodium compared to chlorides. When seawater intrudes a freshwater aquifer, an inverse cation exchange occurs and sodium is captured by the exchanger (clay), while Ca2+ and/or Mg2+ is released, thus water quality changes from Na-Cl rich to CaCl2-rich water and/or Mg-Cl2[12].

Na+, Mg2+, Ca2+/Cl− relationships (meq/l) (a,b,c), ΔNa+, ΔCa2+ and ΔMg2+ relationships with % seawater (d), Mg2+/Ca2+ ratio relationship with % seawater (e) and SO42−/Cl− ratio relationship with chlorides (f) in Diar El Hajjej Garaet Sassi area (June 2006).
Fig. 8. Relations Na+, Mg2+, Ca2+/Cl− (meq/l) (a,b,c) de ΔNa+, ΔCa2+ et ΔMg2+ avec le pourcentage d’eau de mer (d), du rapport Mg2+/Ca2+ avec le pourcentage d’eau de mer (e) et du rapport SO42-/Cl- avec les chlorures (f) dans la zone de Diar El Hajjej Garaet Sassi (juin 2006).
This behaviour is confirmed by the calculation of ΔNa+, ΔCa2+ and ΔMg2+.
In fact, the ΔNa+ is usually negative and the ΔCa2+ and ΔMg2+ are positive (Fig. 8d). The most logical explanation for this excess of Ca2+ and Mg2+ is an inverse cation exchange. This confirms the mixture of freshwaters with seawater. The higher the deviation (Δ), the more important are the processes modifying the content of the mixing.
The Mg2+/Ca2+ ratio increases proportionally to seawater in the mixture [34,38,39]. The SO4/Cl ratio decreases as the seawater proportion in the mixture increases [34,38,39].
This seawater contamination hypothesis is conformed by the fact that the highest Mg2+/Ca2+ ratio values (Fig. 8e) and the weakest SO42−/Cl− ratios (Fig. 8f) characterize the Diar El Hajjej and Garaet Sassi areas samples.
Some Diar El Hajjej area samples are characterized by low SO42−/Cl− ratio (between 0.148 and 0.182) and an average Mg2+/Ca2+ ratio (between 0.668 and 0.681). This is caused by their enrichment in calcium, which originates by the inverse cation exchange between water and clays of the substratum [38].
The samples of Diar El Hajjej-Garaet Sassi areas present SI higher than the modelled values of the conservative mixture. Differences between the theoretical saturation states and the ones calculated for the samples arise due to the non-conservative nature of dissolution and precipitation of the calcite and the CO2 flux () [33]. The Diar El Hajjej-Garaet Sassi samples plot above the calcite SI line for the conservative mixing and all these samples are oversaturated in this mineral. These samples are oversaturated with respect to gypsum. Gypsum SI are generally higher than the theoretical conservative mixing line. This requires a source in SO4 other than seawater like the dissolution of gypsum present in the catchment area or the evaporation of the irrigation water excess. The dolomite SI are higher than 0.
The basic requirement for the deposition of dolomite is that the [Mg]/[Ca] ratio has to exceed 1. This condition is met in the majority of the samples. These ratios are characterized by values higher than those modelled suggesting the presence of another Mg2+ source different from seawater. This water reached the precipitation condition of dolomite.
The Mg/Ca ratios of three samples are lower than those modelled with proportion higher than 5% in seawater. These samples are characterized by dolomite SI > 0.This confirms the assumptions of the dolomite precipitation causing thus the Mg2+/Ca2+ ratio reduction or the inverse cation exchange Na+-Ca2+ and the salinization.
The halite SI follows the conservative mixing line direction. This confirms the marine origin of this mineral.
6.2 Coastal area
The Na+ and Cl− relationship demonstrates that the coastal area samples plot above the seawater-freshwater mixture line (Fig. 9a). This is confirmed by the fact that ΔNa+ is positive in most samples of this area. The most logical explanation for this excess of Na+ is that a direct cation exchange is taking place with the clay substratum. This exchange produces Na+ release to the solution and Ca2+ and/or Mg2+ capture from it. This process is caused by the water flushing. Fig. 9b and c show that most of the samples plot above the mixing line. Two samples taken from the coastal zone have positive ΔNa+ and negative ΔCa2+ and/or ΔMg2+ (Fig. 9d). This shows the contribution of the coastal dunes to the recharge of the aquifer and the presence in this zone of clay layers playing the role of barrier against seawater intrusion.
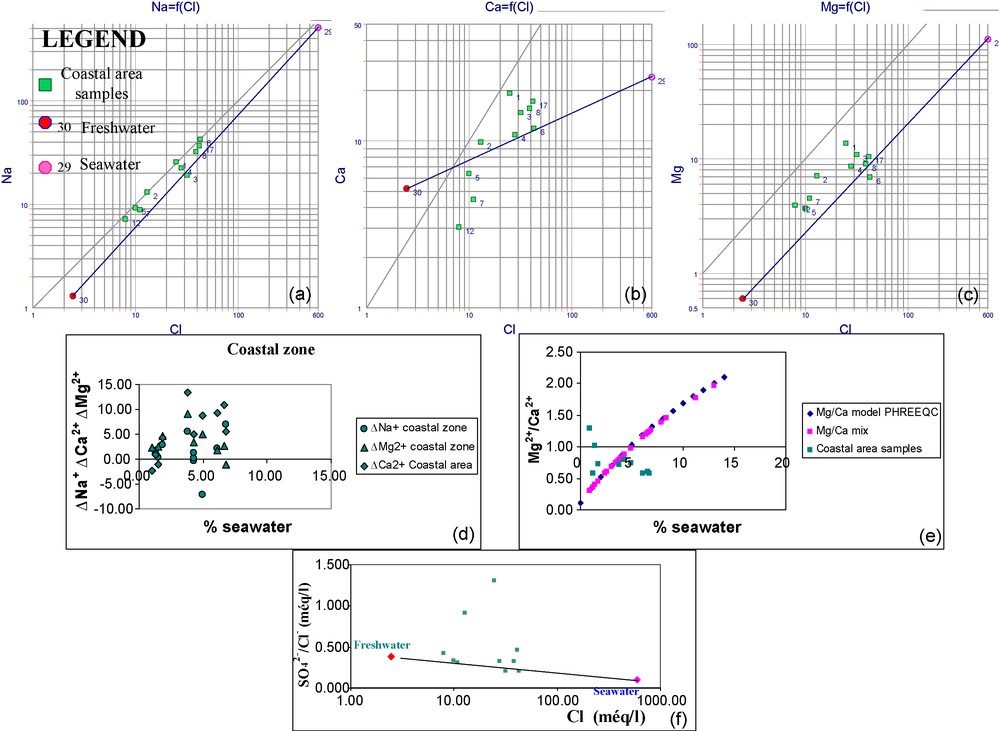
Na+, Mg2+, Ca2+/Cl− relationships (meq/l) (a,b,c), ΔNa+, ΔCa2+ and ΔMg2+ relationships with % seawater (d), Mg2+/Ca2+ ratio relationship with % seawater (e) and SO42−/Cl− ratio relationship with chlorides (f) in the coastal area (June 2006).
Fig. 9. Relations Na+, Mg2+, Ca2+/Cl− (meq/l) (a,b,c) de ΔNa+, ΔCa2+ et ΔMg2+ avec le pourcentage d’eau de mer (d), du rapport Mg2+/Ca2+ avec le pourcentage d’eau de mer (e) et du rapport SO42−/Cl− avec les chlorures (f) dans la zone côtière (juin 2006).
In general, ΔNa+ ΔCa2+ and ΔMg2+ are positive (Fig. 9d). This confirms the hypothesis of the aquifer flushing in the coastal area. The excess of Ca2+ and Mg2+ is due to the dissolution of the carbonates and the gypsum present in the catchment area and dispersed in the aquifer. This phenomenon is accompanied by a sulphate-enrichment confirming the hypothesis of gypsum dissolution. The Ca2+ and Mg2+ excesses cause the calcite, dolomite oversaturations. This is shown in the SI of these minerals calculated by PHREEQC.
Two samples only (No. 3 and 4) have negative ΔNa+. These samples are located in the north of Beni Khiar. They are characterized by an inverse cation exchange.
The most coastal samples present average Mg2+/Ca2+ and SO42−/Cl− ratios values (Fig. 9e and f). These samples are located in the coastal consolidated dunes of the Tyrrhenian. In this area, the aquifer is strongly recharged by meteoric water [14]. This confirms the water flushing theory of the aquifer in the coastal zone.
The majority of samples present SI higher than the modelled values of the conservative mixture. The basic requirement for the deposition of dolomite is that the [Mg]/[Ca] ratio exceeds 1. This condition is met only in two of these samples (No. 7 and 12). In spite of this, the dolomite and calcite SI are negative and dolomitization is not reached. This situation is due to the low salinity (TDS) of these water samples.
The gypsum and halite SI are negative but they are higher than the theoretical conservative mixing line. The behaviour of the halite SI is explained by the excess of Na+. In fact, all the samples plot above the theoretical mixing line. The gypsum SI behaviour requires a source in SO4 other than seawater like the dissolution of gypsum present in the catchment area or the evaporation of the irrigation water excess.
6.3 Internal zone and deep aquifer
The Na+ and Cl− relationship indicates that the majority of the internal area samples approach the mixing line (Fig. 10a) indicating a weak interaction between the freshwater–seawater mixing and the substratum clay. Fig. 10b and c show the calcium and the magnesium behaviour. These cations have the most distinctive distribution pattern in the mixing water sample and exhibit concentrations that exceed the theoretical concentrations. This is produced by the inverse exchange between Na and Ca-Mg caused by seawater intrusion and the dissolution of dolomite.
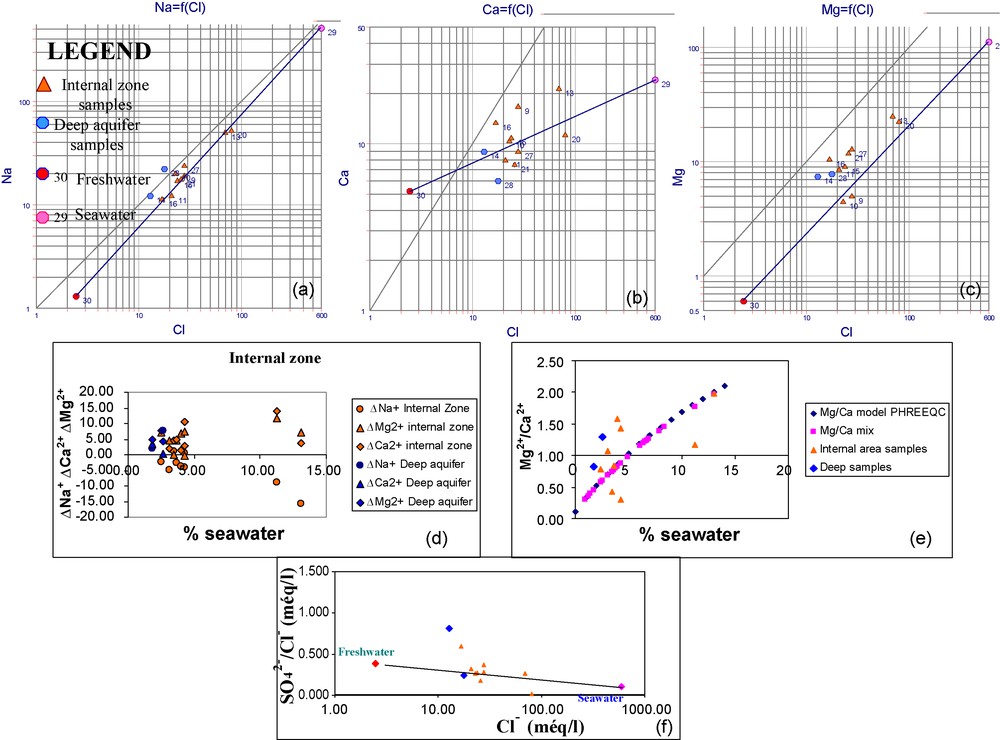
Na+, Mg2+, Ca2+/Cl− relationships (meq/l) (a,b,c), ΔNa+, ΔCa2+ and ΔMg2+ relationships with % seawater (d), Mg2+/Ca2+ ratio relationship with % seawater (e) and SO42−/Cl− ratio relationship with chlorides (f) in the internal area and the deep aquifer (June 2006).
Fig. 10. Relations Na+, Mg2+, Ca2+/Cl− (meq/l) (a,b,c) de ΔNa+, ΔCa2+ et ΔMg2+ avec le pourcentage d’eau de mer (d), du rapport Mg2+/Ca2+ avec le pourcentage d’eau de mer (e) et du rapport SO42−/Cl− avec les chlorures (f) dans la zone interne et dans l’aquifère.
The ΔNa+ is usually negative but these values approach 0 (Fig. 10d). This fact confirms the weak interaction between the mixture and the substratum. As an exception, samples No. 13 and 20 are characterized by negative weak ΔNa+ values and positive ΔCa2+ and ΔMg2+ values. This is in favour with the inverse cation exchange.
ΔCa2+ and ΔMg2+ are positive in all the samples except the samples No. 9 (Fig. 10d). The probable origin of this excess of Mg2+ and Ca2+, in addition to the inverse cation exchange, is the dissolution of the gypsum and the dolomite.
Mg/Ca is higher than 1 or near to 1 (Fig. 10e). This confirms the dolomitization phenomenon.
Only samples No. 9 and 10 have low Mg/Ca values. These values are lower than those modelled by PHREEQC and by those calculated from the Cl concentration. This resulted in weak dolomite saturations indexes. Dolomite and calcite IS are usually positive in this area. Gypsum SI are higher than those modelled. Halite SI are on the mixture line.
In the deep aquifer, the Na+, Ca2+, Mg2+ and Cl− (Fig. 10a–c) relationships demonstrates that these samples plot above the seawater-freshwater mixture line. This is confirmed by positive ΔNa+, ΔCa2+ and ΔMg2+ (Fig. 10d). This Na+ excess is caused by a direct cation exchange with the clay present in the aquifer. This process is caused by the water flushing.
Sample No. 14 presents close Mg2+/Ca2+ (Fig. 10e) and SO42−/Cl− ratios values (Fig. 10f) (respectively, 0.812 and 0.826). This is where the aquifer is recharged by meteoric water. This confirms the water flushing theory of the aquifer in this area. Calcite and dolomite SI are positive in this sample. This explains the Mg/Ca ratio reduction.
On the other hand, sample No. 28 is characterized by a high Mg2+/Ca2+ ratio and a low SO42−/Cl− ratio. In spite of this, the dolomite and calcite SI are negative and dolomitization is not reached. This situation is due to the low salinity (TDS) of this water sample. The gypsum SI is negative but it is lower than the theoretical conservative mixing line.
The behaviour of the halite SI of the two samples is explained by the excess of Na+. In fact, these SI are slightly higher than those modelled.
7 Conclusion
The aquifer of Korba shows especially high values of TDS in the coastal zones, the Diar El Hajjej, Garaet Sassi and Tazerka-Korba. These high TDS values are due to contamination by sea water. This assumption is justified by negative piezometric values, strong chloride concentrations and weak electric resistivity. In the various studied diagrams, we observe a tendency between sample composition and marine water composition, which gives an additional argument in favour of the presence of a marine intrusion in the coastal zone, areas of Diar El Hajjej, Garaet Sassi and Tazerka-Korba.
This seawater intrusion is accompanied by other processes, which modify the hydrochemistry of the coastal aquifer. The most remarkable process is that of the inverse cation exchange, characteristic of the changes of the theoretical mixture of seawater-freshwater, which is carried out between clays and the aquifer water. This exchange consists in the release of Ca2+ and/or Mg2+ and the adsorption of Na+. This process characterizes Diar El Hajjej-Garaet Sassi and the internal areas.
The coastal zone is characterized by a low salinity and weak concentrations chloride. These low (Cl−) values are due to the infiltration of meteoric water in the Tyrrhenian consolidated dunes [14] and to the presence of clay layers playing as a barrier against seawater intrusion (Fig. 2). Moreover, the direct cation exchange between Na+ and Ca2+ and/or Mg2+ (Na+ release and adsorption of Ca2+ and/or Mg2+) indicating a local flushing of water of the aquifer is detected in this zone. This phenomenon is accompanied by a sulphate enrichment. The most probable source of sulphates is the dissolution of the gypsum dispersed in the aquifer.
Other processes such as dolomitization (dolomite precipitation) exist in this aquifer, but they are not as obvious as the cations exchange processes. Dolomitization is indicated by an Mg2+/Ca2+ ratio higher than 1, negative values of ΔMg2+ and positive values of ΔCa2+ and dolomite SI > 0.
It is believed that due to the increased demand for freshwater in agriculture, the area of saltwater intrusion will eventually spread, particularly during dry periods. Consequently, both groundwater monitoring and management and groundwater conservation are essential for efficient surveillance of the saltwater intrusion.