1 Introduction
The ENE-trending Anti-Atlas Mountains extend at the northwestern fringe of the West African Craton, south of, and parallel to the High Atlas (Fig. 1). The origin of the elevation of the Anti-Atlas has been repeatedly questioned as, contrary to the High Atlas, Cenozoic shortening is virtually lacking here. G. Choubert [8] invoked a post-Cretaceous “pli de fond” (crustal folding). Recent works [34] emphasized the role of a high-temperature anomaly of the lithospheric mantle, also responsible for an important part of the Neogene uplift of the High Atlas itself. On the other hand, the Anti-Atlas region was affected by vertical movements coeval with the central Atlantic and Tethyan rifting, west and north of the Anti-Atlas, respectively, during the Late Permian-Late Triassic [11,28]. In the eastern Anti-Atlas, Malusa et al. [32] described by apatite fission track analysis a polyphase exhumation history during the Pangea break-up and Africa–Europe convergence.
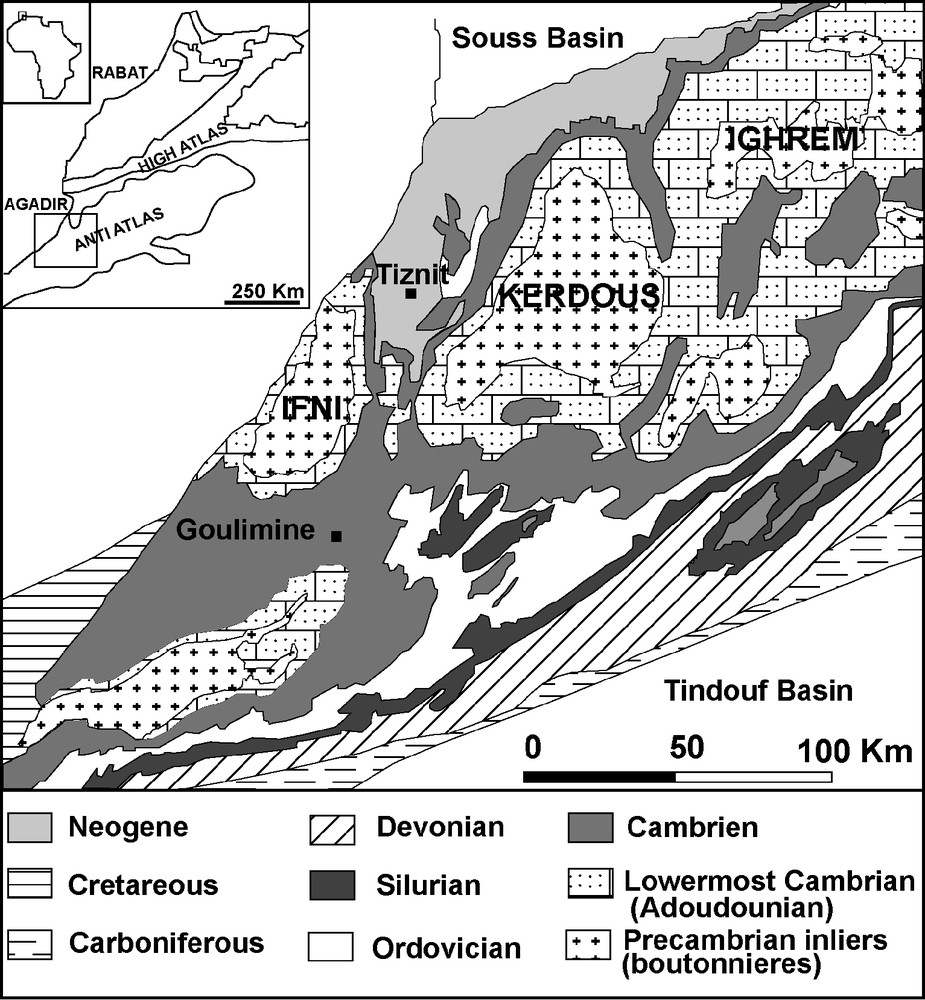
Sketch map and location of the Western Anti-Atlas, after [16]. AZ: Azrou Akchar anticline; JB: Jebel Bani; DP: Draa Plain; LP: Lakhssas Plateau; JO: Jebel Ouarkziz monocline.
Fig. 1. Carte schématique et localisation de l’Anti-Atlas occidental d’après [16]. AZ : Anticlinal d’Azrou Akchar ; JB : Jbel Bani ; DP : Plaine du Drâa ; LP : Plateau de Lakhssas ; JO : Monoclinal de Jbel Ouarkziz.
In order to better constrain the interpretation of the Anti-Atlas vertical movements, we undertook apatite and zircon thermochronological studies on the granites of its basement inliers (“boutonnières”). These basement massifs outcrop beneath a thick Paleozoic cover folded during the Alleghanian-Variscan orogeny [5,8,22,33]. The present paper reports on the zircon fission track (ZFT) analyses on samples from two of the westernmost Precambrian inliers, namely the Kerdous and Ifni boutonnières. Our results allow us to constrain the age of the post-Variscan uplift of the Western Anti-Atlas (WAA), and then, the maximum age of the Variscan deformation itself, which is poorly constrained geologically.
2 Geological background
The Precambrian terranes of the WAA include a variety of metamorphic and plutonic rocks (Figs. 1 and 2) formed during two superimposed orogenies [2,12,13,17,21,33,45]:
- • Paleoproterozoic schists and granitoids emplaced during the Eburnian orogeny (∼2 Ga);
- • their Neoproterozoic (Cryogenian) sedimentary cover (Quartzites and Limestones series : Lkest/Taghdout Group) deformed and recrystallized during the Pan-African orogeny (∼ 660 Ma;);
- • several granitoid generations, emplaced in the Pan-African belt from 610 to 550 Ma;
- • coeval, late- to post-orogenic volcano-sedimentary series (Anezi and Ouarzazate/Tanalt Group, respectively) dated from the Ediacaran.

Sampling maps and results (zircon fission track mean ages). Geological background after the geological map of Morocco, scale 1:1,000,000 [24].
Fig. 2. Carte d’échantillonnage et résultats (Âges moyens traces de fission sur zircon) d’après la carte géologique du Maroc, échelle 1 : 1 000 000 [24].
This complex basement is overlain by a thick sedimentary cover ranging from the Late Ediacaran-Cambrian (“Adoudounian” and Lower Cambrian carbonates; Middle Cambrian greywackes) to the Ordovician siliciclastic series, and to the Silurian shales and Devonian carbonates, sandstones and shales, folded during the Variscan orogeny [5,21,33,43]. Virtually undeformed Carboniferous formations only occur southeast of the WAA, in the Tindouf cratonic basin.
The earliest post-Variscan cover consists of Triassic-Liassic deposits in the Ifni–Tan-Tan coastal basin [1] and the central part of the Souss Basin [15], whereas a Cretaceous onlap is observed elsewhere (southern Souss Basin, Siroua, and Tindouf Basin [15,24]).
3 Methodology and results
Fission tracks are linear zones of damage that form as a result of the spontaneous fission of 238U in the crystal lattice. Tracks are unstable and anneal at high temperature. The range of temperatures over which the tracks anneal is called the partial annealing zone (PAZ). Comparing the density of natural tracks to that of the tracks produced in an external detector by the fission of 235U induced by irradiation of the samples allows us to determine the time when the natural samples cooled below the PAZ [26]. The zircon PAZ (ZPAZ) is considered to be bracketed between ∼330 °C and 230 °C at cooling rates greater than 10 °C per megayear [37,44]. Consistently, the ZPAZ has been constrained between 300 ± 20 °C (FeMg-carpholite isograd) and ∼250 °C in the natural example of the Oman Mountains [39].
Ten samples have been analysed from the WAA basement (Table 1; Fig. 3). Two of them were collected in the Ifni massif (GDI 3, 4), and the remaining eight samples in the Kerdous massif. Zircon grains in the 80–160 μm size range were obtained after crushing and sieving of 3–5 kg rock samples followed by conventional heavy liquids and magnetic separation techniques. They were prepared according to [18], included in a FEP teflon sheet and etched for 18–24 h at 220 °C in an NaOH–KOH eutectic. They were dated by the external detector method using the Zeta calibration [27]. Induced tracks in the kapton foils used as external detectors were revealed with a boiling solution of NaOCl (14%) plus NaCl (12%) during 8 min.
Analytical results: n is the number of grains counted. ρs, ρi and ρd are, respectively, the track density for the standard, the fossil track density and the induced track density. Ns, Ni and Nd are the corresponding number of traces. P(χ2) is the Chi square probability. Zircon fission track ages provided here are central ages.
Tableau 1 Résultats analytiques : n est le nombre de grains comptés. ρs, ρi et ρd sont la densité de traces pour le standard, la densité des traces fossiles et la densité des traces induites. Ns, Ni et Nd sont les nombres de traces correspondants. P(χ2) est la probabilité de Khi2. Les âges traces de fission sur zircon représentés sont des âges centraux.
Samples | Origin | n | N s | ρs × 106 (t/cm2) | N i | ρi × 105 (t/cm2) | ρd × 105 (t/cm2) | P(χ2) (%) | Ages (Ma ± 1σ) |
GDI3 | Ifni granodiorite | 8 | 779 | 4.2 | 87 | 4.6 | 2.20 | 99.9 | 326.5 37 |
GDI4 | Ifni granodiorite | 12 | 974 | 13.7 | 105 | 14.8 | 2.20 | 100 | 337.9 35 |
AGCHTIM | Tafraout granite | 12 | 1388 | 9.6 | 141 | 9.8 | 2.20 | 100 | 358.0 31 |
TAF7 | Tafraout granite | 19 | 2685 | 6.9 | 300 | 7.7 | 2.20 | 99.2 | 326.3 20 |
TAF9 | Tafraout granite | 14 | 2019 | 6.3 | 224 | 6.9 | 2.20 | 99.6 | 328.6 23 |
TAF12 | Tasserirt orthogneiss | 12 | 1087 | 10.3 | 121 | 11.4 | 2.20 | 99.9 | 327.5 31 |
TAF15 | Kerdous Schists | 9 | 954 | 16.2 | 109 | 18.5 | 2.20 | 99.8 | 319.3 32 |
TAF20 | Tasserirt orthogneiss | 14 | 1846 | 5.7 | 208 | 6.4 | 2.20 | 99.8 | 323.7 24 |
TAF21 | Kerdous Schists | 8 | 703 | 12.8 | 79 | 14.4 | 2.20 | 99.9 | 324.5 38 |
TAF22 | Kerdous Schists | 9 | 1374 | 6.7 | 149 | 7.2 | 2.20 | 99.4 | 336.0 29 |
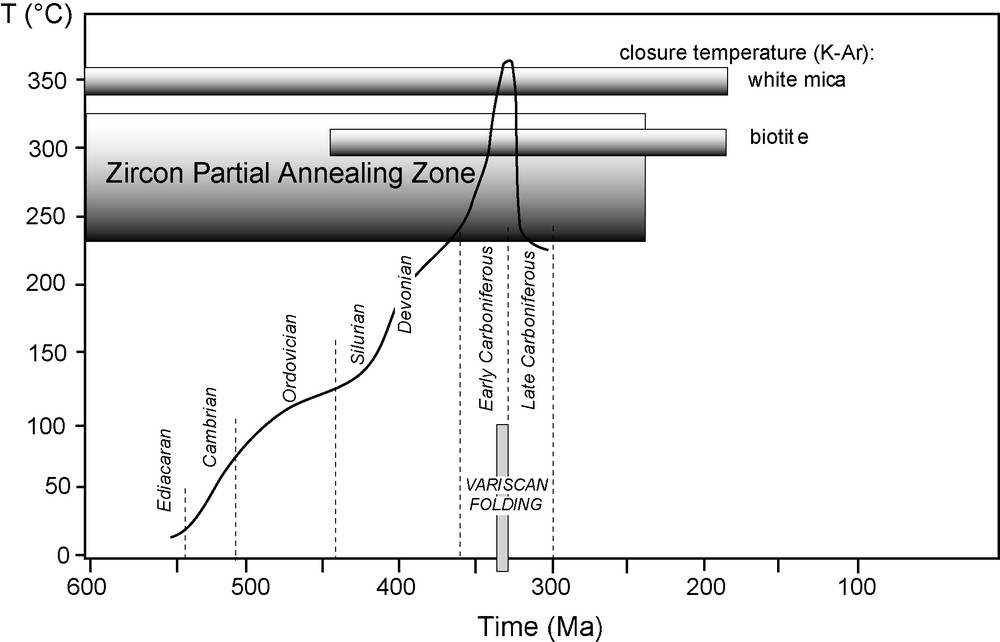
Diagrammatic temperature–time (T–t) path of the western Anti-Atlas basement rocks (Kerdous and Ifni Massifs). Zircon partial annealing zone (ZPAZ) after [37,44]. Mica closure temperatures after [25]. Geological time scale after [19].
Fig. 3 Courbe Chemin température–temps (T–t) du socle de l’Anti-Atlas occidental (Massifs de Kerdous et Ifni). Zone de Rétention Partielle du Zircon (ZPAZ) d’après [37,44]. Température de fermeture du mica d’après [25]. Échelle des temps géologiques d’après [19].
ZFT analyses yield a weighted mean age of 328 ± 30 Ma. All samples pass the χ2 test [20], which means that, in any sample, the ages measured in all crystals are concordant within the statistical uncertainty.
4 Discussion
All our ZFT central ages are equal within the statistical uncertainty to the weighted mean ZFT age (328 ± 30 Ma), whatever the nature and crystallization age of the sample will be. This implies a complete resetting of the Precambrian zircon chronometers by heating above the maximum ZPAZ temperature, i.e. at T greater than 330 °C (see Section 3) before 328 ± 30 Ma, followed by rapid cooling at T below 230 °C (low T boundary of ZPAZ).
Our ZFT ages are consistent with previously published K–Ar datings, i.e.:
- • nine dates ranging between 357 ± 9 Ma and 291 ± 7 Ma (mean age 315 ± 9 Ma) obtained by Bonhomme and Hassenforder [3] from recrystallized white micas (fraction less than 2 μm) from the Anezi and Tanalt formations of the Kerdous massif and contiguous Cambrian formations of the Lakhssas Plateau;
- • the resetting of the biotite grains from the Tasserirt orthogneiss and Tafraout granite between 345 ± 8 Ma and 316 ± 7 Ma [31], cited in [42].
The fact that the ages obtained by ZFT analysis (closure temperature slightly above 230 °C) and those yielded by isotopic systems with different closure temperatures (K–Ar white mica: ∼350 °C; K–Ar biotite: ∼300 °C; [25]) are all grouped around 330–320 Ma suggests that the thermal event responsible for the resetting of these different systems occurred at about this time (Late Visean-Namurian [19]) and was followed by rapid cooling.
Heating of the WAA Precambrian and Lower Paleozoic units above 320–330 °C (this study) or even above 350 °C [3] may be a priori assigned either to burial or syntectonic metamorphism. The role of burial metamorphism in the development of pyrophyllite and illite-chlorite assemblages in the Cambrian-Ordovician formations of the Algerian Saharan Basin has been advocated by Chennaux and Dunoyer de Segonzac [7] and by the latter author in the Adrar Zouggar well of WAA [10]. At the scale of the entire south-western flank of the Anti-Atlas, Ruiz et al. [38] observed that the “illite crystallinity” [14] increases with stratigraphic age and correlates with estimated paleo-overburden, thus fulfilling the conditions of burial metamorphism. Indeed, during the Late Visean-Namurian, the overburden above the WAA Precambrian basement can be estimated at a maximum of 10 km [5] and a minimum of 8 km, taking into account the lateral variations in the stratigraphy of the successive formations [4,9,33,35]. Therefore, assuming a conservative geothermal gradient of 30 °C/km, consistent with the location of the WAA sedimentary basin onto a metacratonic lithosphere, burial heating caused temperature to reach 240–300 °C at the bottom of the Paleozoic cover. This could be enough to account for partial resetting of the zircon in the ZPAZ, but not to account for the white micas recrystallization and the epizonal illite crystallinity [35]. Thus, we must consider a syntectonic thermal event, caused by the tectonic thickening of the folded Paleozoic series and/or the influx of hot pressurized fluids.
In view of the published cross-sections of the WAA fold trains [4,5,33], thickening attained a maximum of 20%. This could have caused heating of the basement up to 300–350 °C, but hardly explain the temperature of ∼350 °C in the Ordovician formations. Then the role of pressurized fluids is likely, and it is in fact consistent with the structure of the western border of the Anti-Atlas in the Ifni-Bas Draa area, which corresponds to that of an accretionary prism at the front of the Mauritanide belt [33,40,41,43]. It is worth noting that the importance of late Variscan hydrothermal recrystallizations has been recognized in other Precambrian inliers from the WAA [16] and eastern High Atlas [36], being dated there at 301 ± 7 Ma and 293 ± 7 Ma, respectively.
We conclude that the studied basement rocks have been heated, together with the overlying Lower Paleozoic series, during the syntectonic event related to the Variscan Orogeny. This is consistent with the fact that folding of these sedimentary units is associated with the development of axial planar cleavage [21,33,41,43]. Moreover, the fact, mentioned above, that heating over ca 350 °C was followed by rapid cooling at 330–320 Ma also supports a causal link with the Variscan Orogeny. This high rate cooling can be ascribed to the post-orogenic erosion of the WAA belt, consistent with the northwestern sources of the detrital input in the Jbel Reouina sandstones (Upper Namurian) of the Tindouf Basin [6]. The Mauritanide Orogeny itself has been dated at 330–310 Ma [29,30], which is also the age of the main Variscan folding in the western Meseta Domain at the northern border of the Anti-Atlas [23,33].
5 Conclusion
ZFT analyses on the WAA Precambrian rocks show that they attained temperature in excess of 320–330 °C at about 330 Ma (Late Visean-Serpukhovian) before being submitted to rapid cooling at T below 230 °C.
Heating of the basement first occurred during its progressive burial beneath ∼8 km thick Paleozoic deposits. However, taking in account the previously published K–Ar datings of the recrystallization of the Ediacaran-Cambrian series, we assume that peak temperature was only attained during the Variscan folding event.
The subsequent exhumation and cooling can be assigned to the rapid erosion of the young mountain belt during the Late Carboniferous, consistent with the coeval accumulation of continental molasses in the Tindouf Basin south of the WAA.
Acknowledgments
We are indebted to P. Van deer Beck and E. Labrin (Joseph-Fourier University, Grenoble) for their help in irradiation process. Thanks are due to A. Cheilletz (CRPG Nancy) and J.P. Liégeois (Royal Museum for Central Africa, Tervuren) for their accurate and constructive reviews. This work was supported by the Volubilis program (MA/05/125).