1 Introduction
The present climate of the Arabian Peninsula is intimately tied to the seasonal development of the monsoon winds and annual migration of the Intertropical Convergence Zone (ITCZ). Arabia is thus in a key position to study past changes in monsoon circulation and latitudinal movements of the ITCZ. However, deciphering such changes is a challenge given the paucity of suitable climate archives. Until the late 1990s, paleoclimatic reconstructions for the Arabian Peninsula were almost entirely based on 14C-dated lacustrine deposits from the Rub’ al-Khali and Nafud deserts [27,47] which indicated the occurrence of two pluvial intervals between 6000–9600 (Neolithic Wet Phase) and 17,000–32,000 years before present (B.P.) [27,39]. While lacustrine sediments from Arabia are, beyond doubt, sensitive indicators for pluvial conditions, they fail to deliver any conclusive information about the source of moisture and to provide continuous and highly resolved records of climate variability [24,25]. Likewise, the range of 14C-dating is limited to the last 50,000 yrs B.P. and thus too short in the context of modern human dispersal (“out of Africa”). Furthermore, 14C-dates were often measured on bulk sediments and thus have, in addition to analytical errors, large age uncertainties due to the so-called “hardwater effect” and contamination by leaching and reworking [19]. As a result of these shortcomings, the detailed terrestrial climatic history of the Arabian Peninsula remained largely unknown. A further source of information is marine sediments from the Red Sea and Arabian Sea [32,40]. In these sediments pollen and terrigenous dust can be used as proxies for continental aridity [2,40,43]. However, as with lacustrine sediments, marine sediment records have a fairly low temporal resolution, are based on 14C chronologies or wiggle matching, and are a proxy for monsoonal wind strength rather than for monsoon precipitation. Overall, both lacustrine and marine sediments provided a rather rudimentary picture of Pleistocene and Holocene changes of the hydrological cycle on the Arabian Peninsula. This changed in the late 1990s when the invention of optically stimulated luminescence (OSL) and uranium-series dating (Th–U dating hereinafter) allowed paleoclimatologists to use sand dunes and speleothems (stalagmites, stalactites and flowstones) for more extended and detailed paleoclimatic reconstructions. Since then the number of paleoclimate studies from the Arabian Peninsula has increased progressively [6–9,14–17,20,28,33–35,42]. Although aeolian sediments can be dated by OSL, age uncertainties are typically in the range of several hundreds to thousands of years [6,7,33,34], even for Holocene samples. Such large uncertainties make it difficult to correlate regional and hemispheric climatic events and to detect short-lived discontinuities in aeolian sequences. Crucial questions, such as about the origin of moisture and short-term (10–103 years) climatic fluctuations, remained largely unanswered. This gap of knowledge can be closed by studying the oxygen (δ18O) and hydrogen (δD) isotopic composition of speleothem calcite and fluid inclusions respectively. A major advantage of speleothems (stalagmites, stalactites and flowstones) is that they can be precisely dated back to approximately 600,000 yrs B.P. and Th–U age uncertainties are considerably smaller compared to 14C- and OSL-dating. As caves are abundant and scattered across the Arabian Peninsula, speleothems have the greatest value in delivering highly resolved and accurately dated paleoclimate records for up to the last 600,000 yrs B.P.
Stalagmites presented in this paper were collected from two caves in Oman, Hoti Cave (23°05′N, 57°21′E) in northern Oman and Qunf Cave (17°10′N, 54°18′E) in southern Oman [8,9,14–18,28] (Fig. 1). Paleoclimatic information was gained by dating periods of stalagmite growth and by measuring δ18O and δD of speleothem calcite and fluid inclusions respectively.
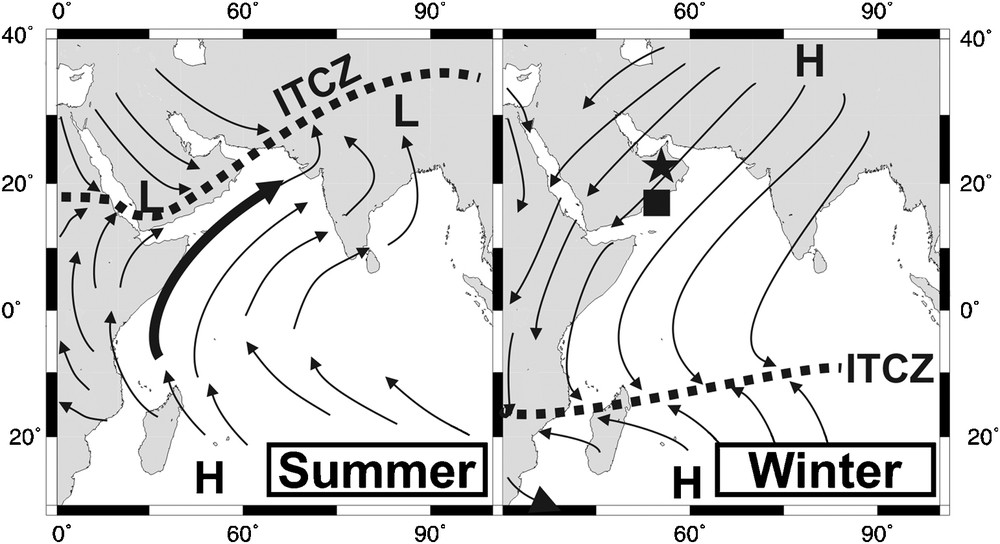
Wind pattern during northern hemisphere summer and winter. The thick black line denotes the approximate location of the East African low level jet, also known as Findlater or Somali jet. Dotted line denotes the location of the ITCZ, black symbols mark location of Hoti Cave (star symbol) and Qunf Cave (square).
Fig. 1. Direction des vents au cours de l’été et de l’hiver dans l’hémisphère nord. Le trait noir épais indique la localisation approximative du jet de mousson dans les basses couches, également appelé « Findlater » ou « Somali jet ». Le trait pointillé indique la localisation de l’ITCZ. Les symboles noirs indiquent la localisation des grottes d’Hoti (étoile) et de Qunf (carré).
2 Modern climatology
The present-day climate of the Arabian Peninsula is strongly governed by the reversing monsoonal winds and annual latitudinal migration of the ITCZ (Fig. 1). In spring the ITCZ moves northward across the Indian Ocean and reaches its northernmost position in August, at the peak of the Indian Summer Monsoon (ISM). From June to September, a strong low-level monsoonal airflow prevails and transports large quantities of moisture, which is then released as monsoon precipitation over the southernmost parts of Arabia and India. In autumn the ITCZ then retreats southward and reaches it southernmost position at approximately 20°S in January. The reversed pressure gradient during the winter months generates the moderate northeast monsoon.
In Northern Oman (Hoti Cave) precipitation originates from three sources:
- • Mediterranean frontal systems;
- • convectional or orographic precipitation;
- • tropical cyclones [45].
Precipitation associated with Mediterranean frontal systems usually occurs between December and March (Fig. 1), is widespread and lasts between several hours and a few days. In contrast, convectional or orographic precipitation is restricted to the mountainous regions, of short duration, limited spatial extent and occurs during the summer months. Tropical cyclones originate either in the southeastern Arabian Sea or in the Bay of Bengal and reach northern Oman once every 5 to 10 years, causing heavy precipitation for several days [45].
In southern Oman (Qunf Cave), the most important source of precipitation is the ISM. The ISM lasts from about June to September, when strong southwesterly winds blow over the Arabian Sea, inducing upwelling of colder water near the coast. Over these upwelling zones the moist air masses condense to form banks of fog and clouds, which are transported by the East African low level jet (also known as Findlater or Somali jet) to the south side of the Dhofar Mountains [17]. Monsoon rains fall as a fine drizzle, seldom exceeding more than 5 mm d−1. Occult precipitation, when the clouds condense at the ground and/or vegetation surfaces during the monsoon season, is another important source for groundwater recharge in this region. Monthly averages of rainfall (not including occult precipitation) show that about 75% of total annual rainfall falls during the monsoon season. Another potential source for rainfall is cyclones, which make landfall in southern Oman every 7 to 10 years, usually in March, April or May. These rainfall events, however, are of short duration (few days) and not the primary source of groundwater recharge as shown by a number of groundwater data from wells and karstic springs [10].
3 Speleothem-based paleoclimate reconstructions
The growing interest in speleothems (derived from the Greek words spelaion = cave and thema = deposit) as a paleoclimatic archive results from recent advances in dating techniques [37] and the steadily growing demand for well-dated and highly-resolved climatic time series matching those obtained from Greenland ice cores [21]. Speleothems are formed when calcium carbonate precipitates from degassing groundwater seeping into limestone caves and have several strengths as they [13]:
- • can grow continuously for 103–105 years and can be precisely dated by 230Th dating;
- • contain a number of physical and chemical proxy variables, such as annual growth layers, stable isotope ratios and trace elements;
- • faithfully capture the cave's response to the external environment;
- • show little or no secondary alteration.
For environmental and climate reconstructions mainly stalagmites were used due to their fairly simple growth geometry that facilitates sampling.
Chronologies of all stalagmites presented in this study are based on 230Th-dating, which provides absolute ages and, in contrast to 14C dating, in most cases no correction is needed. Because deposition of speleothems relies on sufficient water supply, intervals of speleothem growth are indicative of wetter climate conditions [9].
The most important and frequently used climate-related parameter in speleothems is δ18O of calcite (δ18OC hereinafter). Provided that a stalagmite grows in isotopic equilibrium with its parent drip water, δ18OC is dependent on temperature; an increase of 1 °C in cave air temperature, for instance, results in a negative isotopic shift of ≈ 0.25‰ in δ18OC [23]. However, in all caves the temperature-dependent fractionation of 18O during calcite precipitation is masked by precipitation-controlled variations in δ18O of cave drip water from which the speleothem is formed. δ18OC values are therefore primarily influenced by δ18O of cave seepage waters and meteoric precipitation respectively, whereas δ18O and δD of precipitation (δ18OP and δDP hereinafter) are controlled on different time scales by a variety of climatic variables [38], such as the amount of rainfall (the so-called “amount effect”), surface air temperature, seasonality of precipitation (e.g., proportion of winter and summer precipitation), shifts in the source of moisture and/or storm tracks (so-called “source effect”) as well as changes in δ18O of the ocean (so-called “ice volume” effect) [38]. This makes δ18OC and δD in speleothem fluid inclusions (δDFI hereinafter) useful parameters for reconstructing climate-related changes in δ18OP and δDP.
Analytical methods for δ18OC and δDFI measurements are described in detail in ref. [14]. The proxy quality of each of these parameters is described hereinafter.
4 Oxygen and hydrogen isotopic composition of precipitation, groundwater and cave drip water in Oman
Before variations in δ18OC and δDFI in stalagmites from Oman can be correctly interpreted in terms of climate, the basic principles controlling modern δ18OP and δDP must be well understood. The few existing isotopic studies in northern Oman reveal that precipitation from northern (Mediterranean frontal systems) and southern (Arabian Sea and Bay of Bengal) moisture sources differ greatly in their isotopic composition (Fig. 2a). While precipitation from a northern moisture source ranges from −4.5 to +1.0‰ (Vienna Standard Mean Ocean Water [VSMOW]) in δ18OP and from −25 to +5‰ (VSMOW) in δDP, precipitation from a southern source is much more depleted, with δ18Op values varying between −10 and −2‰ and δDP values between −75 and −15‰ (Fig. 2a). The two sources of moisture define separate Local Meteoric Water Lines, a Northern Local Meteoric Water Line (N-LMWL) (δD = 5.0 δ18O + 10.7) and a Southern Local Meteoric Water Line (S-LMWL) (δD = 7.1 δ18O − 1.1) [45,46]. The isotopic composition of young, tritium-containing groundwater samples (n = 11) in the vicinity of Hoti Cave and cave drip water samples (n = 3) plot between both local meteoric water lines, indicating that precipitation from both moisture sources recharges local groundwater [14,26,45,46].
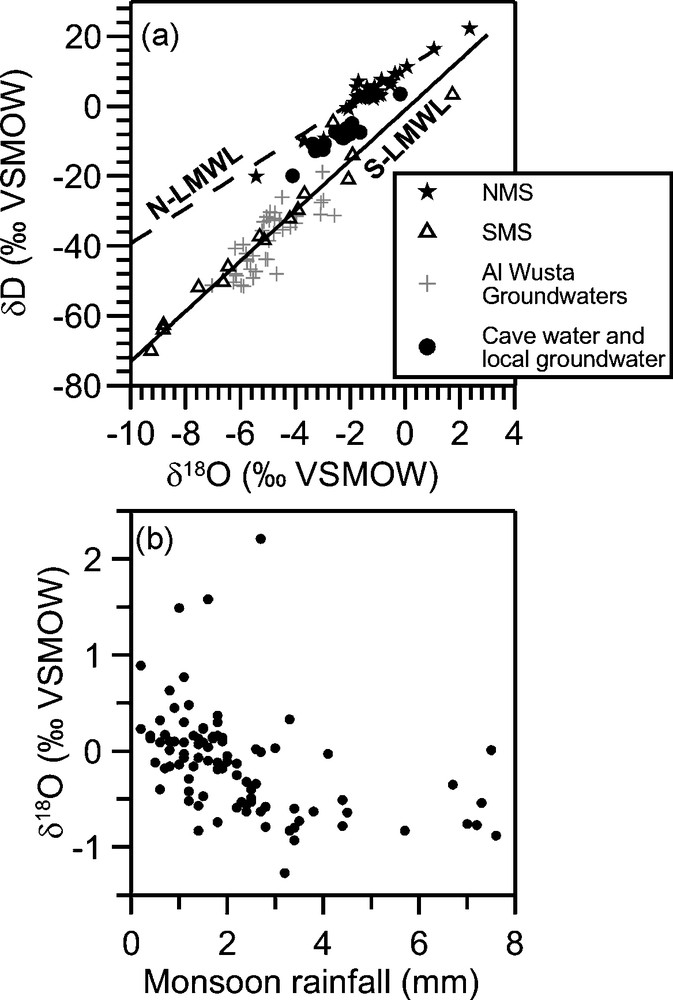
a: isotopic composition (δD and δ18O) of precipitation, local groundwaters and cave drip waters for northern Oman and Hoti Cave respectively (NMS: northern moisture source; SMS: southern moisture source); b: isotopic composition of monsoon precipitation in Southern Oman.
Fig. 2. a : composition isotopique (δD et δ18O) des précipitations, des eaux souterraines locales et des eaux de percolation pour l’Oman septentrional et la grotte d’Hoti (NMS : précipitations originaires du nord ; SMS : précipitations originaires du sud) ; b : composition isotopique des précipitations de mousson en Oman méridional.
In Southern Oman δ18OP values of monsoon precipitation vary between –1 and 1.0‰ (VSMOW) and are thus very close to original seawater values of around 0.0‰ (VSMOW) (Fig. 2b). This is because precipitation has a very short meteorological history and fractionation effects during evaporation and condensation cancel one another [10]. Typical for many tropical regions, a moderate inverse correlation exists between the amount and δ18O of monsoon precipitation (the so-called “amount effect”), with higher monsoon rainfall exhibiting more negative δ18O values [38].
When no evaporation occurs at the surface or in the epikarst, δD and δ18O of cave drip waters are close to the mean annual isotopic composition of precipitation. However, in regions with an arid or semiarid climate, such as in Oman, evaporative processes can bias the isotopic composition of infiltrating water with respect to precipitation [3]. In this case, δ18O of cave drip waters and δ18OC would become more positive. Evaporative effects, however, seem to be negligible as δD and δ18O values of cave drip waters in all studied caves in Oman are identical or very close to those of precipitation above the cave [4,16].
In summary, in Oman δ18OP and δDP are primarily influenced by variations in the amount and/or changes in the source (Mediterranean versus Indian Ocean) of precipitation. Furthermore, the isotopic composition of cave drip waters in all studied caves in Oman is identical or very similar to that of surface precipitation. Therefore, speleothems formed from these waters can be considered to be faithful recorders for δ18O and δD of local precipitation in the past.
5 Late and Mid-Pleistocene climate variability
Because speleothem growth is dependent on the availability of water, intervals of speleothem deposition are a first hint for more pluvial conditions. Based on a total of 98 Th–U dates performed on 11 stalagmites and one flowstone from Hoti Cave, at least five periods of speleothem formation occurred since around 330 kyr B.P. (Fig. 3) [8,9,14–18,28]. Despite considerable age uncertainties for Th–U ages older than 200 kyr, speleothem deposition in Hoti Cave seems to fall within peak interglacial periods, corresponding to marine isotopic stages (MIS) 1 (Holocene), 5.a (5.1), 5.e (5.5), 7a (7.1), and 9 [22]. An important feature of stalagmite H13 is that it comprises several periods of speleothem deposition, which are interrupted by long periods of nondeposition. Because growth was reactivated multiple times within a single sample, the intervening hiatuses are taken to have been continually dry.
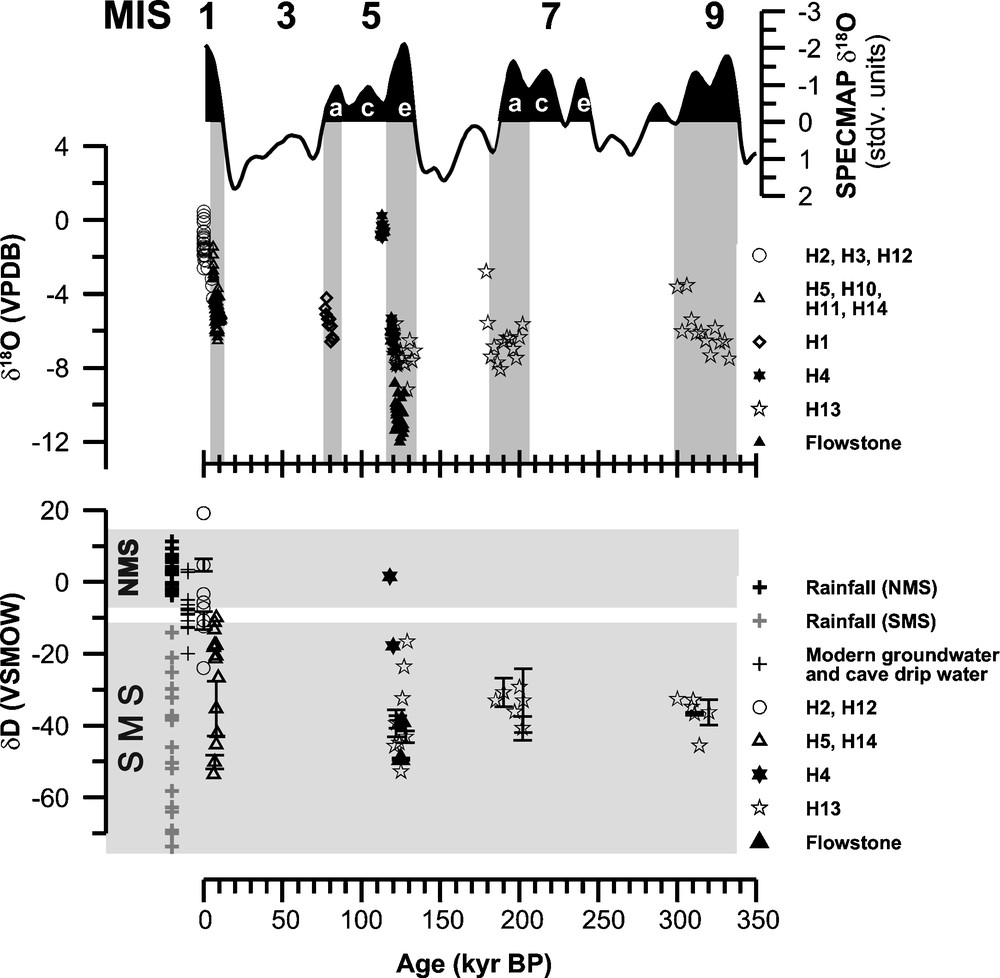
Composite δ18OC record for Hoti Cave. Also shown is the SPECMAP [22] marine oxygen isotope curve and marine oxygen isotope stage (MIS) numbers. Composite δDFI for Hoti Cave (including the results of duplicate samples, black dots with error bars) is also shown. The grey shaded areas mark the isotopic range of precipitation originating from a Northern (NMS) or Southern (SMS) Moisture Source.
Fig. 3. Enregistrement composite du δ18OC à Hoti. Sont également figurés la courbe des valeurs isotopiques de l’oxygène des sédiments marins (SPECMAP) et les numéros des stades isotopiques marins (MIS) [22]. Enregistrement composite de δDFI pour Hoti (incluant le résultat des échantillons dupliqués – points noirs et barres d’erreur inclus). Les zones grisées montrent l’éventail des valeurs isotopiques des précipitations originaires du Nord (NMS) et du Sud (SMS).
While Th–U dates reveal at least five pluvial periods in northern Oman, it is unknown from where the moisture originated. Such information can be obtained by δ18C and δDFI as both are a proxy for the isotopic composition of cave seepage water and meteoric precipitation respectively. Based on isotopic studies in northern Oman—outlined in section 4—changes in δ18OP and δDP are known to be primarily driven by the origin of the atmospheric vapor and in addition by the amount of precipitation (“amount effect”) (Fig. 2a and b). Under modern climatic conditions, with most of total annual precipitation originating from a northern (Mediterranean) moisture source during winter and spring, actively growing stalagmites from Hoti Cave (samples H2, H3 and H12) exhibit δ18OC and δDFI values between −2.6 to −1.1‰ (VPDB) and −7.6 and −3.3‰ (VSMOW) respectively (Fig. 3). In contrast, δ18OC and δDFI values of stalagmites deposited during interglacial pluvial periods are much more negative and range from −8 to −4‰ (VPDB) and −53 to −20‰ (VSMOW) respectively (Fig. 3). Such a distinct isotopic difference cannot be explained by higher cave air or surface air temperatures during peak interglacial periods, because higher temperatures would influence solely the temperature-dependent fractionation of δ18O during calcite precipitation (“cave temperature effect”, approximately −0.25‰ per 1 °C increase [23]), but not δDFI. Furthermore, a depletion of 1‰ in δ18OC would require a temperature increase of 4.5 °C, but groundwater data from Oman demonstrate that temperatures during the Early and Middle Holocene were within 1–2 °C of present-day temperatures [45]. Therefore, it is highly unlikely that much lower interglacial δ18OC and δDFI values are the result of temperature changes. Rather, δ18OC variations of this magnitude most likely resulted from significant changes in δ18OP and δDP. Based on the isotopic studies on modern precipitation in Oman, such negative δ18OC and δDFI values are characteristic for meteoric precipitation originating from a southern moisture source (Indian Ocean), indicating that northern Oman was under the direct influence of the ISM and precipitation was considerably higher during peak interglacial than today.
Interestingly, differences in δ18OC values between individual interglacial periods are also apparent (Fig. 3). δ18OC values of stalagmites deposited during the Early to Middle Holocene and MIS 5a generally vary between −6.5 and −4.0‰ (VPDB), whereas MIS 5e, 7 and 9 speleothems show on average lower δ18OC values, ranging from −8 to −5‰ (VPDB). The lowest δ18OC values between −12 and −9‰ (VPDB) were measured in a flowstone from Hoti Cave that was deposited on the cave wall during MIS 5.5, though such negative δ18OC values are rather characteristic for extreme monsoon rainfall events when water was flowing down the cave wall on which the flowstone was deposited. As δ18OP and δDP are inversely correlated with the amount of monsoon precipitation ([16], Fig. 2b), more negative δ18O values of calcite deposited during MIS 5.5, 7 and 9 suggest that ISM precipitation was even higher than during the Early and Middle Holocene (Fig. 3).
A recently published stacked marine Indian Monsoon (IM) record (Fig. 4), which is based on five independent proxies for IM wind strength (lithogenic grain size; barium mass accumulation rate (MAR); δ15N; abundance of Globigerina bulloides and opal MAR) from two cores from the northern Arabian Sea, suggests that equally strong IM winds occurred during glacial as well as during interglacial periods [11]. During MIS 3, for instance, the stacked marine indicators of IM wind intensity are even higher than those during the Holocene and MIS 5.5 (Fig. 4). This is in stark contrast to the timing of pluvial periods recorded in Northern Oman, which are restricted to peak interglacial periods. The apparent differences between the marine and terrestrial monsoon records indicate that precessional forcing in wind intensity does not necessarily lead to pluvial conditions on the Arabian Peninsula. Instead, glacial boundary conditions (e.g., ice volume) seem to be an important control for pluvial climatic conditions, which tend to coincide with minimum glacial boundary and maximum insolation forcing.
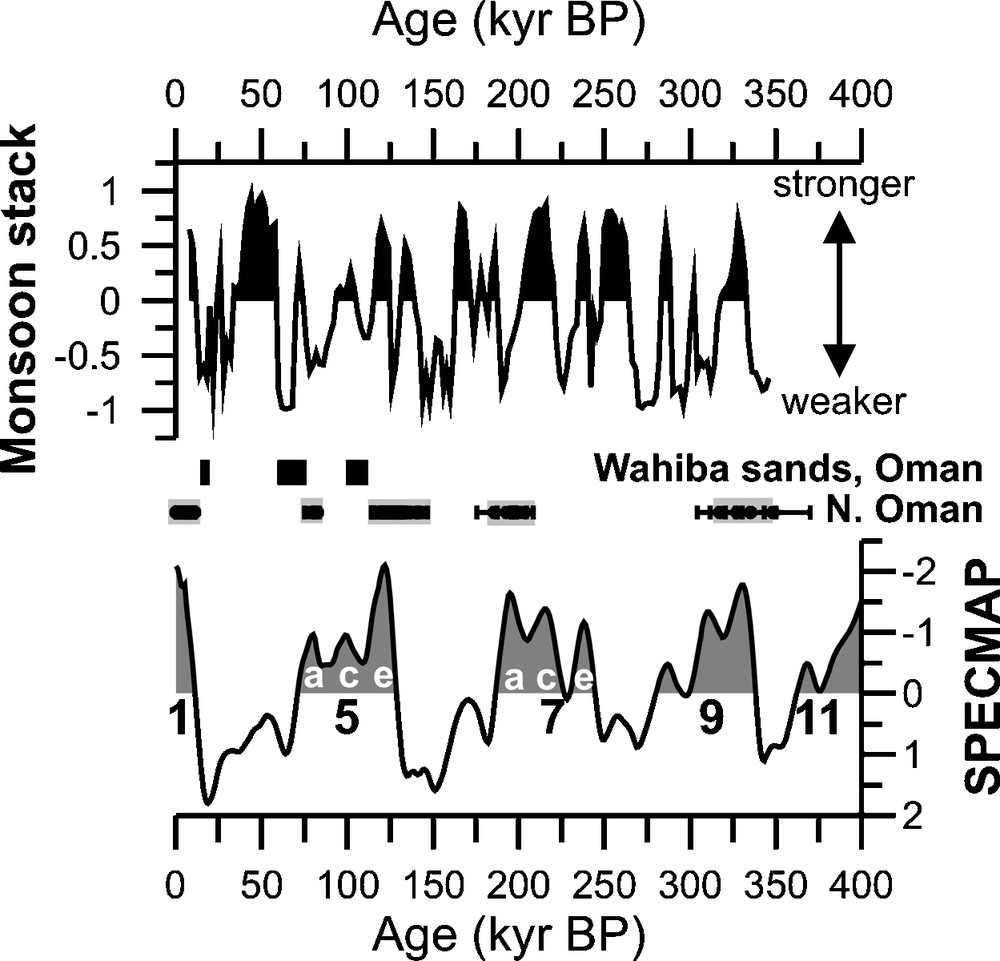
Comparison between a stacked record of monsoon wind strength [11] and phases of speleothem deposition in Hoti Cave, northern Oman. Positive monsoon stack values indicated stronger summer monsoon winds. Black bars denote phases of dune activity due to increased aridity in the Wahiba sands [33].
Fig. 4. Comparaison des enregistrements compilés de l’intensité des vents [11] et des phases d’accrétion des spéléothèmes à Hoti, Oman septentrional. Les valeurs positives indiquent les phases de forte activité de mousson. Les traits noirs indiquent les phases d’activité dunaire en relation avec l’aridité accrue dans la région des Wahiba sands [33].
6 Holocene climate variability
Holocene climatic reconstructions are primarily based on stalagmites H5 and H12 from Hoti Cave in northern and stalagmite Q5 from Qunf Cave in southern Oman. The combination of precise 230Th ages with small errors of 1–2% and highly-resolved δ18OC profiles allows us to reconstruct the Holocene climatic history of the Southern Arabian Peninsula in unprecedented detail. In Oman speleothem deposition—indicative of more humid conditions—starts between 10.5 and 10.1 kyr B.P. (Fig. 5). While marine cores from the Arabian Sea suggest a two-step increase in monsoon intensity at ≈ 13–12.5 and at 11–9.5 kyr B.P. respectively [29,40], there is no speleothem-evidence in Oman for an onset in monsoon precipitation prior ≈ 10.5 kyr B.P. [17]. This onset date for monsoon precipitation in southern Arabia is in good agreement with a 14C-dated peat section from the Dhamar highlands in Yemen, which has a basal age of 10.3–10.6 kyr B.P. [12]. Terrestrial records from southern Arabia thus suggest that the summer ITCZ and the associated ISM rainfall belt were most likely located south of the Arabian Peninsula prior to ≈ 10.6 kyr B.P. A key question is whether the timing for the onset of pluvial conditions in southern Arabia was synchronous or rather time-transgressive in nature. The stalagmites suggest that monsoon precipitation starts and peaks around 400 years later in northern than in southern Oman (Fig. 5). As Hoti Cave (57° 21′E, 23° 05′N) is located approximately 600 km to the north of Qunf Cave (17°10N, 54°18 E), this 400-year time lag resulted most likely from the northward migration of the ITCZ and the associated monsoon rainfall belt. Supporting evidence for our observation comes from the Awafi lacustrine sediment sequence (25 N) in the United Arab Emirates, which indicates a cessation of dune emplacement due to increasing precipitation at ≈ 9.0 kyr B.P. [30,31], significantly later than in Oman. These lines of evidence reveal that the onset of Early Holocene pluvial conditions at a time of maximum solar insolation was thus not synchronous but rather time-transgressive across Arabia.
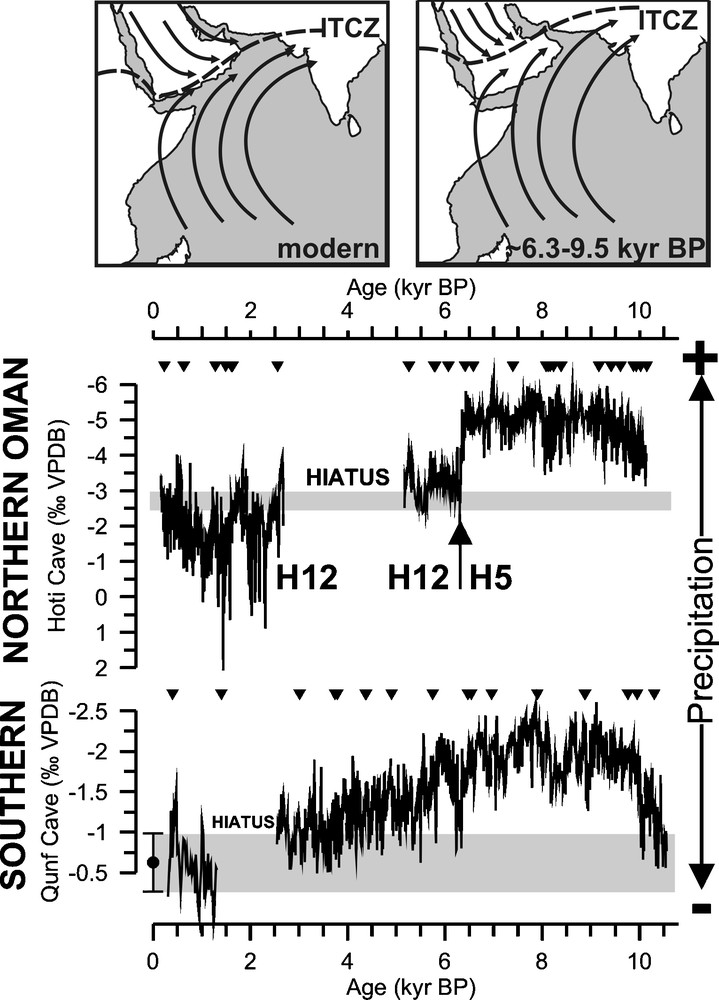
Holocene δ18O-profiles from Hoti and Qunf Cave. Gray shaded boxes denote δ18O values of modern stalagmites. The upper figures show the generalized summer circulation patterns at present (left figure) and between approximately 9.5 and 6.3 kyr B.P.
Fig. 5. Profil isotopique holocène des valeurs de δ18O à Hoti et Qunf. Les boîtes grisées montrent les valeurs isotopiques actuelles des stalagmites. Les figures du haut montrent la circulation atmosphérique générale en été aujourd’hui (figure de gauche) et entre approximativement 9,5 et 6,3 kans BP.
The marked decrease in δ18OC between ≈ 10.5 and ≈ 9.2 kyr B.P. indicates a steady rise in monsoon precipitation due to a rapid strengthening of the ISM and northward displacement of the summer ITCZ as a result of high solar insolation (Fig. 5, [15]). An area that is particularly sensitive to displacements in the position of the summer ITCZ is southern Oman (Qunf Cave), where currently strong convective cloud development is prevented by a temperature inversion due to the convergence between hot, dry northwesterly winds blowing from the Arabian Desert and the relatively cool and moist low-level southwest monsoon winds. The height of this temperature inversion is dynamically linked to the mean latitudinal summer position of the ITCZ and to the wind pattern over southern Arabia [41]. During the Early and Middle Holocene, a northward displacement of the ITCZ into the Arabian Peninsula would have led to a rise of this temperature inversion and thus stronger convective cloud development and higher monsoonal rainfall over southern Oman as suggested by the Q5 δ18O profile (Fig. 5). However, the amount of monsoon precipitation is not only dependent on the mean latitudinal summer position of the ITCZ, because the low-level monsoon winds transport large amounts of moisture. Therefore, convective cloud development is also driven by the overall influx of moisture to southern Oman. Weaker (stronger) monsoon winds would reduce (increase) moisture flux and intensity of convective activity and hence, monsoon rainfall over southern Oman. In conclusion, we suggest that displacements of the ITCZ and variations in monsoon intensity act in tandem, such that periods of a northward (southward) displaced ITCZ coincide with intensified (reduced) monsoon circulation and stronger (weaker) convection above southern Oman. Because of the “amount effect”, δ18OP values are expected to become more negative with increasing precipitation. Stalagmite Q5 δ18OC values from southern Oman, therefore, reflect changes in the amount of monsoon precipitation, which are likely controlled by the mean latitudinal summer position of the ITCZ and by the strength of the ISM and intensity of convective activity.
The Hoti and Qunf Cave stalagmite δ18O profiles support the common notion of a wet Early to Middle Holocene pluvial period; monsoon precipitation was generally high between approximately 9.5 and 6.3 kyr B.P. (Neolithic Wet Phase). At around 6.3 kyr B.P., a distinct positive shift of 1 to 3‰ indicates a marked decrease in monsoon precipitation. In northern Oman (Hoti Cave), this isotopic shift of 3‰ marks the termination of the Early to Mid-Holocene monsoonal period as a result of a southward latitudinal shift of the summer ITCZ. After 6.3 kyr B.P., high H12 δ18OC values of around −3‰ indicate that most of precipitation originated from a northern (Mediterranean) moisture source (Fig. 5). This isotopic shift also implies a change in the seasonality of precipitation, viz. from summer (prior 6.3 kyr B.P.) to winter/spring rainfall. Conclusively, Hoti Cave stalagmite δ18OC values indicate that the summer ITCZ and the associated monsoonal rainfall belt were located mainly south of Hoti Cave. Qunf Cave in southern Oman, however, was still affected by monsoon precipitation after 6.3 kyr B.P. (as it is today) and Q5 δ18OC values continue to decrease until the hiatus at ≈ 2.7 kyr B.P. (Fig. 5). The ultimate cause for the interruption in stalagmite deposition between ≈ 2.7 and 1.4 kyr B.P. is uncertain, and a blocking of the fissure feeding stalagmite Q5 or a significant drop in monsoon precipitation are plausible. Interestingly, the hiatus in stalagmite Q5 coincides with an interval of enhanced dune deposition in the Liwa region [6] and the Wahiba Sands [35] in the United Arab Emirates and Oman, respectively, suggesting that the hiatus between ≈ 2.7 and 1.4 kyr B.P. resulted from a marked decrease in ISM precipitation. As mentioned before, in southern Oman the long-term gradual decrease in Q5 δ18OC since the mid-Holocene reveals a continuous southward migration of the summer ITCZ and reduction in monsoon precipitation. The comparison between stalagmite δ18OC time series from Hoti Cave (23°N) and Qunf Cave (17°N) clearly indicates that the termination of the humid period on the Arabian Peninsula was not a single event but rather time-transgressive, because of the gradual southward migration of the ITCZ and the associated monsoon rain belt. The timing of the Middle to Late Holocene humid–arid transition is thus dependent on the geographical position of the monsoon proxy record. An abrupt humid–arid transition may occur in a particular record as soon as the mean latitudinal position of the ITCZ and the associated summer monsoon rainfall belt are displaced south of the study site, as indicated by the abrupt isotopic shift at ≈ 6.3 ka B.P. in the composite Hoti Cave stalagmite record from northern Oman. Our results from Oman thus suggest that the termination of the humid period is asynchronous across the southern Arabian Peninsula.
The Holocene Q5 and H5 δ18O-profiles show distinct decadal- to multidecadal-scale variability in monsoon precipitation. During the Early Holocene, two distinct ≈ 100-year long minima in monsoon precipitation are apparent at 9.2 kyr and 8.2 kyr B.P. (so-called “8.2 kyr cold event” [1]), both of which coincide with intervals of distinctly lower temperatures in Greenland [36] and central Europe [1,18]. Modern instrumental data as well as paleoclimate archives from the Asian monsoon domain [4] support this association between North Atlantic temperatures and monsoon intensity. It is believed that colder temperatures and a greater extent of snow cover impedes the warming of Eurasia and thereby reduces the pressure gradient that ultimately drives the ISM. While during the Early Holocene decadal to multidecadal changes in monsoon precipitation are closely related to the atmospheric dynamics in the North-Atlantic realm, short-term fluctuations in IOM precipitation also occurred after 8 kyr B.P. A comparison between the detrended Q5 record [15] and 14C production record, a proxy for solar output [5], shows a strong similarity. Intervals of weak (strong) solar activity correlate with periods of low (high) monsoon precipitation (Fig. 6). However, the visible correlation becomes less clear in the Late Holocene when the Δ14Cres record shows only small amplitude variations in solar activity. Results of spectral analysis further reinforce our interpretation that second-order variations in IOM precipitation were triggered by changes in solar activity, as indicated by statistically significant major solar cycles centred at ≈ 220 (de Vries), ≈ 140, ≈ 90 (Gleissberg cycle) and 13–10 (sunspot cycle) years in the Q5 δ18OC paleoprecipitation record [15]. Additionally, the cross-spectral analysis between both records confirms the correspondence of statistically significant solar cycles at 205, 132, 105–90 years. Such a close sun–monsoon connection on decadal to multidecadal time scales is confirmed by other monsoon records, such as the Dongge Cave record from China [44]. Whether variations in solar output affect the IOM indirectly, via internal forcing mechanisms, or directly via external forcing mechanisms is not fully resolved yet.
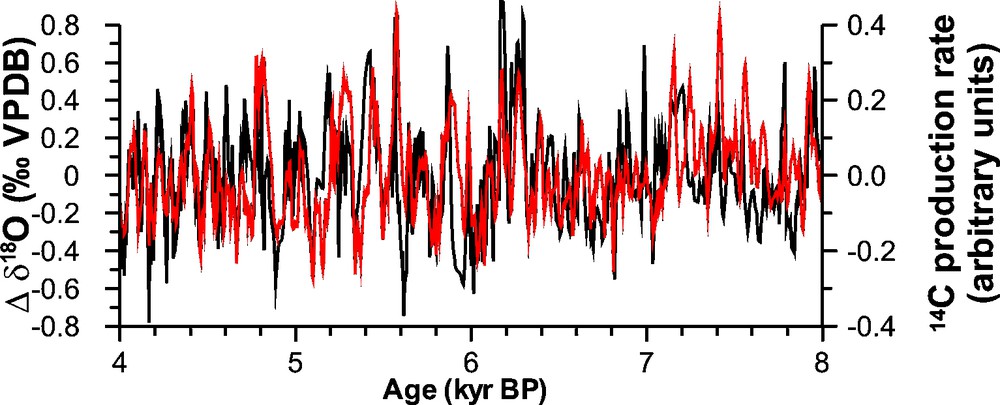
Comparisons of the detrended Q5 (Qunf Cave) δ18O-profile with solar variability on centennial to millennial time scales [15]. The 14C production rate (data kindly provided by J. Beer) is used as solar signal. Intervals of enhanced monsoon precipitation (more negative δ18O) coincide with intervals of higher solar activity (negative values).
Fig. 6. Comparaison de l’évolution des valeurs de δ18O de la stalagmite Q5 (Qunf) avec la variabilité solaire à l’échelle de la centaine au millier d’années [15]. La production en carbone 14 est utilisée comme signal solaire. Les intervalles de précipitation de mousson (valeurs les plus négatives de l’δ18O) coïncident avec les intervalles d’intense activité solaire (valeurs négatives).
7 Conclusions
Th–U dated speleothems from caves in Oman provide detailed information on the timing of pluvial periods over the last 330,000 years B.P. [8,9,14–18,28]. Based on stalagmites from Hoti Cave in northern Oman at least five pluvial periods occurred at around 6.3 to 10.5, 78 to 82, 120 to 130, 180 to 200 and 300 to 330 kyr B.P., with little or no growth during the intervening periods. All of these periods coincide with peak interglacials, when orbitally-controlled summer insolation and glacial boundary conditions were at maximum and minimum respectively. δ18OC and δDFI values indicate that precipitation originated from a southern moisture source as a result of an intensified monsoon and more northerly position of the ITCZ. During the Early Holocene (10.5–9.5 kyr B.P.), the mean latitudinal position of the summer ITCZ advanced rapidly northward. However, convection within the ITCZ and ISM intensity is, despite orbitally-induced maximum solar insolation, suppressed by glacial boundary conditions (e.g., enhanced Eurasian snow cover). Short-term (10–102 yr) changes in ISM intensity correlate well with high-latitude temperature variations recorded in Greenland ice cores, with reduced (enhanced) convection and lower (higher) ISM intensity being associated with cooler (warmer) high-latitude air temperatures. From 7.8 kyr B.P. to present, the mean summer ITCZ continuously migrated southward and ISM intensity and precipitation decreased gradually in response to solar insolation.