1 Introduction
The water and the energy cycles are together influencing climate. The equilibrium between the incoming solar radiation and the outgoing longwave radiation (OLR) at the top of the atmosphere (TOA) put a large constraint on the system through the radiative equilibrium. The radiative budget is furthermore strongly modulated by the atmospheric water elements like water vapour and clouds (Fig. 1). The radiative-convective equilibrium at play in the deep Tropics further links the radiation cycle to the water cycle through the energy and water vertical transport by deep convection. Finally, evaporation acts as a significant cooling factor of the surface. As a consequence, the surface temperature of the Earth results from the intimate coupling of the water and energy cycle of the atmosphere.

The Earth annual energy budget in Wm−2. From Kiehl and Trenberth, (Kiehl and Trenberth, 1997).
Bilan radiatif annuel de la Terre en Wm−2. Tiré de Kiehl and Trenberth (Kiehl and Trenberth, 1997).
In the Tropics, these cycles are stronger than elsewhere around the globe. The annual excess of incoming solar radiation is not compensated locally by the emission of longwave (LW) radiation and leads to the atmosphere and ocean circulations that export away this excess of energy. Evaporation reaches its maximum over the subtropical oceans, over the trade-winds areas characterized by very low rain amount; water vapour is transported in the low levels towards the meteorological equator where heavy rainfall occurs. The tropical land masses distribution further complexifies the Hadley/Walker cells by inducing the monsoon circulations that transport the moisture from ocean to the land and lead to the annual precipitation. The Tropics are hence characterized by a very active water and energy cycle. In the tropical atmosphere, water is found in vapour phase, in ice crystals in cirrus clouds, in liquid droplets in low levels cloudiness as well as in the form of both ice and water hydrometeors associated with precipitation. All these phases of the H2O molecule interact with the radiation and energy budget of the Earth. Beyond the singularity of the condensation process and phase change within clouds and rainy systems, the interaction between the water vapour and the long wave radiation is also remarkably non linear. An equivalent perturbation in a dry environment will impact more the OLR hence the greenhouse effect than in a moist environment. As a consequence, the analysis of these interactions is complex. In the Tropics where the conventional meteorological network is clearly unfit to the problem, satellite measurements offer a nice opportunity to characterize the water and energy cycle (Roca et al., 2002). The advent of space observations indeed led significant modifications of our understanding of the functioning of the energetic of the monsoon revealing the role of the net radiant energy available to earth–atmosphere system as a fundamental driving force for monsoon rainfall (Srinivasan and Joshi, 2007).
The on-going climate change recalls if needed, the central role of the tropical water and energy cycle in setting the climate sensitivity. Beyond the well known positive feedback due to water vapour, recent investigations on the cloud feedback, suggest that the main reason of departure of the estimation of the climate sensitivity between the multiple models of the fourth assessment report of the International Panel Climate Change (IPCC) rises from their representation of the shortwave (SW) cloud radiative forcing of the low levels subtropical cloudiness (Bony and Dufresne, 2005). Similarly, the recent simulations of the rainfall patterns in the framework of the IPCC scenario at the end of the century by the two French climate models exhibit strong divergence over the monsoons areas (Douville and Terray, 2007) that calls for a better constraint on the model simulation of tropical rainfall. More generally, the observed recent climate trends from satellite are difficult to assess and to confront to the climate model behaviour (Trenberth et al., 2007). In short, stronger constraint from the observational record together with a better understanding of the functioning of the tropical water and energy cycles is required to address the relevance of the model's response to an increase in greenhouse gas concentration. Satellites provide one very unique way to do so and the present paper illustrates some activities oriented towards this scientific objective.
The present article belongs to the Special Issue on Earth observations where the reader will find a number of contributions more or less directly related to the present topic. In particular, the contributions by Kandel and Viollier, Prigent et al., Clerbaux et al., and Tanré et al. bring in discussions on the remote sensing of radiation and rainfall using a variety of platforms. Recent instruments are also introduced there (Interféromètre atmosphérique de sondage infrarouge IASI, Parasol…). As a result, we here focus on uncovered aspects of the remote sensing of the water and energy cycles and we encourage the reader to complete his/her analysis thanks to the whole Special Issue. The article is organized as follows. First, a rapid summary of the history of satellite measurements is presented together with some emphasis on the most recent period. Challenges for the near future about the observations and understanding of the tropical water and energy cycle are then detailed. The emphasis is set on the need for downscaling of the tropical water and energy to the smaller scales, to better understand the role of clouds in the monsoon circulation, to bring more closely end-users and rainfall retrieval experts and to elaborate climatic trends from the 20 plus years of meteorological satellite archive. Needed supporting observations and their usefulness are finally introduced to address the underlined challenges. A short conclusion ends the paper.
2 Past and present
2.1 An historical perspective
The main elements of the water and energy cycle (convective systems, rainfall, water vapour distribution and the radiative budget) were among the first priorities in the history of Earth observations from Space; it was felt especially important to document these variables in the tropical continental and oceanic regions for which the conventional network back then was very scarce.
The potential of space observation for meteorological studies appeared as soon as 1948 with the US Air Force program on the Upper Atmosphere Research panel, which operated from White Sand launching pad V-2 and Aerobee rockets. The first photographies of convective clouds (Fig. 2) have been released with a paper of Delbert Crowson untitled “Cloud observations from rockets”. Although satellite platforms were not yet considered, it was the inaugural step on mesoscale convective systems studies. The first US satellite Vanguard 2 (1959) and Tiros 1 (1960) carried cameras for cloudiness observation and the data collected by the TIROS satellites series allowed the first study on a complex convective system (Browning and Ludlam, 1962). These results paved the way for the development of the meteorological space observation system and Applications Technology Satellite (ATS)-1, the first geostationary satellite launched in 1966, carried among other telecommunication devices a camera. In 1970, (Barrett, 1970) proposed a first rainfall monthly estimation from low earth orbiting (LEO) satellite data and showed that the time sampling was not sufficient for a reliable estimation (Frequent observations from geostationary platform only became available later when Geostationary Operational Environmental Satellite [GOES] ran operational in 1974). The GARP Atlantic Tropical Experiment (GATE) was held during summer 1974 and led to, among other results on deep convection organization, milestone references by Stout et al. (Stout et al., 1979) and Arkin (Arkin, 1979) on monthly rain estimates from geostationary images. It is only a few years later that the Global Precipitation Climatology Program started in 1986. The main goal of this programme was to produce global precipitation climatology over 10 years relying heavily on satellite based rainfall estimation.

Photography of convective systems. V-2 rocket 26 Jul. 1948. Courtesy of U.S. Army.
Photographie d’amas convectifs. V-2 rocket 26 Juillet 1948. Courtoisie de l’Armée américaine.
Early attempts to describe the general circulation of the atmosphere did not include the various thermal processes at play in the atmosphere (radiation, condensation fluxes of sensible and latent heat) and the first radiative scheme that introduced the effects of water vapour, carbon dioxide and ozone absorptions of terrestrial radiation was initiated by Manabe and Möller (Manabe and Möller, 1961). The need for a documentation of the stratification of the main radiative gases was then felt and Möller (Möller, 1961) mentioned an “artificial satellite (…) (with) a device to measure the radiation between 6 and 7 μm”. The first of a long series of such devices was the Temperature-Humidity Infrared Radiometer (THIR) that flew onboard the Nimbus-4 satellite through the GARP programme and sounded the mid-tropospheric water vapour content with a dedicated 6.7 μm channel (Allison et al., 1972). The first METEOSAT platform (1977) flew with a so-called “water vapour” channel at 6.3 μm that was mainly used as a tool for the study of the atmospheric motions in cloud free areas (Morel et al., 1978). Some case studies showed the water vapour channel relevance for the dynamics of the mid-latitudes jet streams (Ramond et al., 1981). In the tropical belt, the warm values observed in this channel were associated to a mid-tropospheric subsiding motion, with a convergent flux aloft and a divergence near the surface (Picon and Desbois, 1990).
The radiative budget of the Earth was among the first Earth variables to be investigated from Space. Suomi radiometers dedicated to the measurements of the SW and LW portion of the electromagnetic spectrum were indeed among the first instruments embarked in the first meteorological satellites. From Explorer VII in 1959 to the Nimbus series in the 1970s, the global Earth albedo was hence estimated to be around 29% and the effective radiation temperature around 255 K (Raschke et al., 1973). Regional repartitions were also provided, showing for example a large annual deficit over Sahara. (See also Barlier, [Barlier, 2010] this issue, for a broader historical perspective).
This series of first attempts, trials and failures paved the way to the modern era of monitoring of the water and energy cycle from Space. The more recent efforts greatly inherited, at both the technical and the scientific levels, from this rich innovative period. To a large extent, the scientific and technical questions raised back then are still relevant.
2.2 More recently
Since this pioneering era, progress has been accumulated thanks to a series of dedicated space missions with improved instrumentation and simultaneously with improvements with retrieval techniques from the historical archive as well as through a constant increase in the validation effort. While it is out of the scope of the present work to review all of these, we shall next focus on some illustrative examples of the above, relevant to the elaboration of long time series of observations and their use to better document the water and energy cycles elements and their representation in atmospheric models.
2.2.1 Radiative budget
Earth Radiation Budget (ERB) data have been provided by two NASA missions: ERB Experiment (Barkstrom et al., 1985) and Clouds and the Earth's Radiant Energy System (CERES) (Wielicki et al., 1995). This series has been completed by the ScaRaB instrument designed at LMD (Monge et al., 1991; Kandel et al., 1998). The algorithms and data processing for ScaRaB were also developed at LMD (Viollier et al., 1995). Two models were launched in space in January 1994 on the Meteor-7 satellite (Kandel et al., 1998) and in August 1998 (Duvel et al., 2001). A third model of ScaRaB is now planned to be launched in 2010 on the French-Indian Megha-Tropiques (MT) mission (see paragraph 4.2). A new series of ERB instruments is Geostationary ERB (GERB) (Harries et al., 2005) which provides ERB estimates over Africa and surroundings from the geostationary satellites Meteosat-8 and 9 since 2003. POLDER provides also a significant contribution to the estimation of the SW reflected flux (Buriez et al., 2007). This long time series of observations has generated a large amount of analysis studies ranging from the documentation of the tropical inter-annual variability, especially during El Nino (L’Ecuyer and Stephens, 2007), to climatic trends estimation of the tropical radiation budget (Wielicki et al., 2002) (see also paragraph 3.4).
2.2.2 METEOSAT long time series
A significant element of the last decade is the advent of an historical record from meteorological satellites like the operational National Oceanic and Atmospheric Administration (NOAA) satellites and the fleet of geostationary satellites. Of particular interest to the topic, are the METEOSAT series starting a continuous record from 1983 up to nowadays. It provides a long and more or less homogeneous description of the clouds and water vapour in the atmosphere among other parameters. Indeed using the window channel, it is possible to detect and analyse the population of Mesoscale Convective Systems (MCS) observed over West Africa during the monsoon period which contribute to the major part to the annual rainfall there (Mathon et al., 2002). Another relevant example concerns the use of the 6.3 μm channel to document the humidity in the free troposphere although inhomogeneity in the calibration series makes the task harder.
The analysis of the morphology of tropical convective systems has indeed received much attention thanks to the geostationary infrared data. The analysis of these observations indeed led to a large corpus of information on the degree of organization of convection, of occurrence of this type of systems, on the evolution of the cold cloud shield life cycle, all over the inter-tropical belt (Laing and Fritsch, 1997). Statistics on the size, duration, propagation of the systems as well as the link to the thermodynamical and dynamical environment etc… have been acquired. Tropical system morphology is now built up from roughly more than 20 years of continuous observations (Fiolleau et al., 2009). Fig. 3 shows the 24-year average spatial distribution of one class of systems (the one with the longest duration and largest propagation speed). This class of systems is mainly encountered in the Sahelian band and this figure underlines the need to account for the salient features of this type of organized convection to understand the rainfall distribution in this region.

Contribution to the total cold cloudiness by the Class 4 of MCS defined at MCS lasting more than 9 hours and propagating faster than 10 m/s. Summer season averaged over 1983–2006 included. Units: %.
Contribution à la nébulosité froide totale de la classe 4 des amas convectifs définis comme durant plus de 9 heures et se propageant à plus de 10 m/s. Saison d’été moyennée sur la période 1983–2006 incluse. Unités : %.
The 6.3 μm channel is centered on the strong rotation-vibration band of water and is used to monitor the atmospheric water vapour field since the first METEOSAT. Under clear sky conditions and in the Tropics, these radiance data are well related to the Free Tropospheric relative Humidity (FTH). It corresponds to an elaborated vertical average of the relative humidity using the Jacobian of Brightness Temperature as a vertical operator (Brogniez et al., 2004) (see also the seminal paper using GOES data of Soden and Bretherton [Soden and Bretherton, 1993]) over a broad layer of the troposphere. A systematic analysis of the METEOSAT archive has been undertaken and covers the July 1983 to June 2005 period at a 3 hourly time step at a resolution of 0.625°. This archive has been corrected from temporal inhomogeneities due to the different filter functions of successive radiometers and to modifications in the vicarious calibration technique (Brogniez et al., 2006; Picon et al., 2003) and then adjusted on High Resolution Infrared Radiation Sounder (HIRS)-12/NOAA-12 water vapour channel. Comparisons with European Centre for Medium range Weather Forecasts (ECMWF) soundings archive show a small dry bias (−1.23%) and a good temporal stability (σ = 1.70%) over the whole period (Brogniez et al., 2009). This rich long-term database provides a unique opportunity to study the inter-annual variability of FTH over the Africa/tropical Atlantic area. In particular, the very dry conditions encountered over the eastern Mediterranean area during summer has been shown to exhibit large interannual relative variability in FTH related to the mixing of tropical and extratropical air masses that explains a significant part of the interannual variations of FTH there (Brogniez et al., 2009). More generally, in the intertropical belt, the distribution of mid-tropospheric water vapour departs from Gaussian statistics in dry areas. Consequently, arithmetical average is unsuitable to characterize the humidity field variability in these regions and one needs to rely on the distribution of the humidity. The high time resolution of the METEOSAT archive is particularly well adapted to go beyond monthly averages and to document the full distribution of humidity. Preliminary analysis using the relative humidity of the NCEP reanalysis, indeed shows large fluctuations of the dry end of the distribution at the interannual scale (Fig. 4) that are strongly relevant to the long wave radiative budget of the Tropics (Spencer and Braswell, 1997).

Distribution of relative humidity at 500 hPa using NCEP reanalyses during the boreal winter over the period 1977–2007. (Upper) Fraction of the time with dry air (units are %). (Lower) Composite difference (significant at the 95% level based on a t test) of these dry air fraction between the mean of seven El Niño and six La Niña events (Dry air is characterized by RH lower than 10%).
Distribution de l’humidité relative à 500 hPa à partir des réanalyses du NCEP pendant l’hiver boréal sur la période 1977–2007. (Haut) Fraction du temps avec de l’air sec (les unités sont des %). (Bas) Différence composite (significative au niveau 95 % selon le test T) des fractions d’air sec entre la moyenne de sept événements El Niño et six La Niña. L’air sec est défini avec RH inférieur à 10 %.
2.2.3 TRMM and MSG, AMMA
Another significant event in the last decade relevant to the tropical water and energy budget observations is the success of the Tropical Rainfall Measuring Mission satellite (TRMM) (Kummerow et al., 1998). TRMM flies on an orbit inclined on the equator at 35° and carries on board a suite of instruments among which the first space rain radar. The exploitation of these observations gave a wealth of results. Similarly, the outcome of the Meteosat Second Generation (MSG) (Schmetz et al., 2002) satellites (with enhanced space and time sampling and multispectral capability) brings the water and energy cycle elements observations to a new era. Finally, the Analyse multidisciplinaire de la mousson africaine (AMMA) campaign (Redelsperger et al., 2006), that recently took place in West Africa, helped to gather a large amount of observations dedicated to the water cycle and is also one of the important events of the decade providing a unique ground based observational support for the development of satellite estimates.
The improvement gained from these new observations is particularly obvious in the case of the rainfall estimation. Indeed, the production of global precipitation maps is based on the merge of various rainfall estimators from three data sources: rain gauge ground network, microwave sensors on low orbiting satellites and visible/infrared sensors on geostationary orbiting satellites. The estimation errors of each basic product are due on the one hand to the spatiotemporal sampling and on the other hand to the uncertainties of precipitation intensity retrieval. The infrared geostationary data offer both the best sampling characteristics and the worse instantaneous rate estimation. In contrast with the previous products generation, which was based on simple statistics extracted from infrared data, the current trend is to focus on passive microwave estimators. However, the rain gauge network brings still valuable information. During the AMMA experiment, a new algorithm (EPSAT-SG) has been developed to supply accumulated rainfall estimates over West Africa (Bergès et al., 2009a). This algorithm runs in two steps. During the first step rough scale precipitation estimators are produced from microwave observations and rain gauges. Then, the MSG data are used to downscale this information, the geostationary data are used for a probalistic mapping of rainy area in combination with TRMM radar observations. In the AMMA implementation of the algorithm, only one rough scale precipitation dataset is ingested, the GPCP dataset (Huffman et al., 2007). In order to use fully the multispectral characteristics of the new MSG sensor, the fuzzy mapping is performed through a feed forward neural network. This method has been intercompared with other rainfall estimation algorithms for three Sahelian rainy seasons (Jobard et al., 2009). The results demonstrate a good behaviour of EPSAT-SG, which performs better than the global products on this area. The high temporal sampling (15 min) and radiometric resolution of MSG both contribute to this quantum jump in the quality of the geostationary rainfall estimations. Recent efforts, based on a study that makes use of GOES data, and of a cloud growing rate index shows encouraging results to better characterize the diurnal cycle within the EPSAT-SG framework (Bergès et al., 2009b). More information on the results of the AMMA program are readily accessible in Janicot et al. (Janicot et al., 2008) and Lebel et al. (Lebel et al., 2009).
This enhanced observing system also permitted the elaboration of a better quality atmospheric motion vector (AMV) product used to constrain the tropical circulation in the analysis centres. AMVs are derived from triplets of satellite images and represent the motion of clouds or other atmospheric structures, such as pure water vapour structures (detected in WV absorption channels). In a large majority of cases, AMVs are identical or very close to the wind at the level of the cloud or atmospheric structure. AMVs are particularly useful at low levels in the Tropics: they can be associated to the monsoon flow, where they show the humidity flux in the lower layers towards the large convective systems generating precipitation although the extraction of these vectors is difficult over land (Szantai and Sèze, 2008).
The enhanced tropical sampling due to the TRMM orbit has generally increased the quality of most of the rainfall products. The latest release of GPCP indeed benefits from the TMI derived rain rates and is useful to analyze General Circulation Model simulations. Fig. 5 shows a comparison between GPCP and the LMDz model (Hourdin et al., 2006). The model is run in low resolution in a nudged mode (Coindreau et al., 2006) using the National Centers for Environmental Prediction (NCEP) Global Forecast System (GFS) analysis to guide the model. Such configurations concur to a realistic synoptic variability representation in the model, which helps its validation against observed dataset. The results indicate that the model captures well the large-scale structure of the Inter-Tropical Convergence Zone (ITCZ) during the monsoon 2006 with a slight wet bias in the northern part of the Sahel. While the range of rainfall encountered over the region is well reproduced and so is the Guinean maximum, the model fails to reproduce the observed Mt Cameroon extreme rainfall. Such preliminary comparisons are useful to orient the development of the model and to evaluate its usefulness in the Tropics.

Model Simulation (left) and satellite estimations (right) of the seasonal mean rain rate during the AMMA campaign in summer 2006. Units are mm/h.
Simulation de modèle (gauche) et estimations satellite (droite) du taux de pluie saisonnier moyen pendant la campagne AMMA en été 2006. Les unités sont des mm/h.
These past and on-going studies illustrate the kind of questions related to our current understanding of the water and energy cycle and are likely to be relevant in the next decade as well. A few milestones to address these broad objectives are listed next.
3 The challenges for the near future
A number of challenges concern the science of water and energy cycle in the Tropics for the immediate future. We focus below on a limited number of topics for which we expect to provide some contributions in the coming years.
3.1 Down to the convective systems scale
Most of our knowledge on the life cycle of the tropical systems from a physical point of view (rainfall distribution, latent heating and radiation cooling rate vertical distribution, water transport, shear etc.) mainly arises from individual observational campaigns heavily based on ground radar observations and Cloud Resolving Model modelling (Houze, 2004). While this large body of observations and simulations permitted the building of useful conceptual models of tropical system life cycle, it nevertheless does not reach any integrated perspective statistically significant yet. As discussed above, the morphology of the cloud systems has received a good understanding thanks to geostationary platforms over the last decades. The advent and long duration of the TRMM mission completes this by now offering the possibility to bridge the numerous individual case study physical observations and the climatological morphological approach (Zipser et al., 2006). The merging of these two datasets (from geostationary and from LEO satellites) in order to provide a dynamical composite of the systems life cycle composed of morphology from GEO and physical parameters from LEO is now at hand (Futyan and Del Genio, 2007). Fig. 6 shows such an example. This figure reveals two different conceptual models of MCS for ocean and land with a distinct initiation phase over land characterized by substantially more convective rainfall than at the end of the life cycle. Such cloud objects approaches can be extended to the radiation field as well (Xu et al., 2005) but it requires a higher accuracy in the flux estimates at the smaller scales than in the past. New methodological developments are showing encouraging results (Viollier et al., 2009) that should help in this downscaling effort. The composite approach can also be extended to the moist environment of the systems that plays an important controlling role on the life cycle of the organized systems (Roca et al., 2005a).
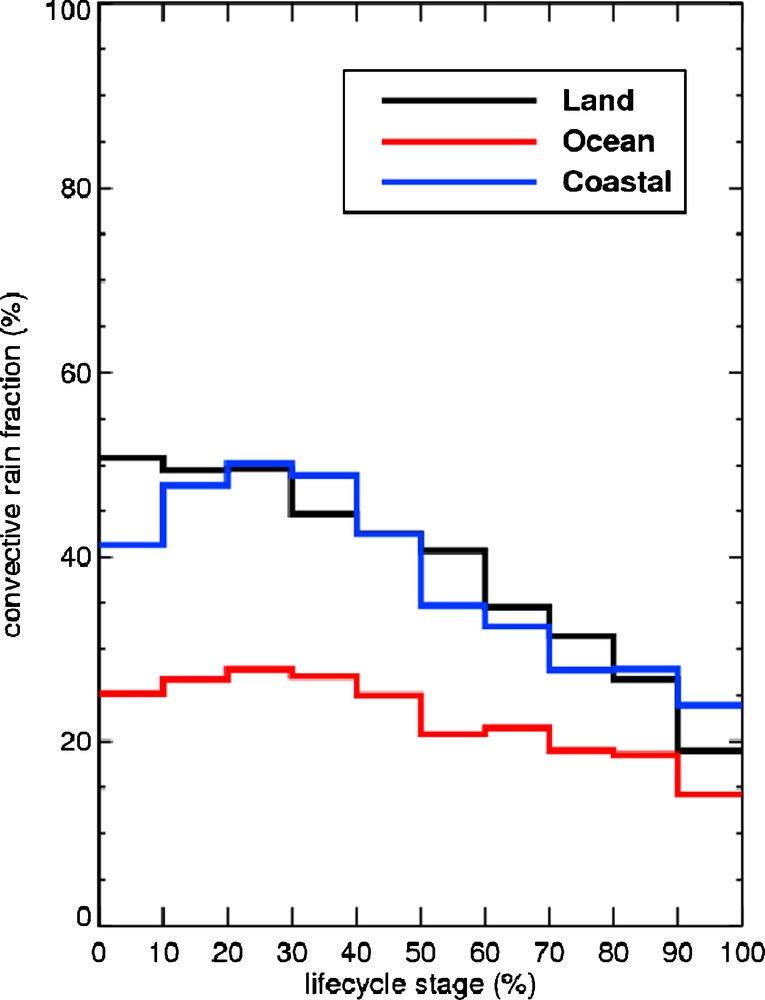
Convective rain fraction of the systems as a function of the life cycle stage over the West African and Atlantic Ocean region. Eight summers of data have been used. Convective rain fraction is estimated thanks to the TMI observations and the Viltard et al. algorithm (Viltard et al., 2006).
Fraction de pluie convective des amas convectifs en fonction de leur cycle de vie sur l’Afrique de l’Ouest et l’océan Atlantique. Huit étés de données ont été utilisés. Cette fraction convective provient des observations de TMI et de l’algorithme de Viltard et al. (2006).
3.2 The cloud radiative forcing in the monsoon
In the Tropics, the deep convective clouds have long been associated with a quasi-null cloud radiative forcing at the TOA (Kiehl and Ramanathan, 1990) with important consequences on the understanding of the cloud feedback on climate (Stephens, 2005). Explanation for this near cancellation ranges from the deep and thick cloud bounded by the tropopause for which near cancellation is found (Kiehl, 1994) to the averaged effects of multiple cloud types (Cess et al., 2001). Rajeevan and Srinivasan (Rajeevan and Srinivasan, 2000) emphasized the fact that near cancellation occurs there because the upper clouds over the Warm Pool exhibit large optical depths but with moderate fractional coverage. On the other hand, they underlined that the strong negative cloud radiative forcing observed over the Bay of Bengal during the monsoon that would act as a negative feedback onto the monsoon (Sharma et al., 1998) is due to the large optical depth and the large cloud fraction observed there (Wu and Kau, 2007) suggesting a strong role of the cloud SW radiative properties. The role of the moist environment of the deep clouds in the region has also been investigated. Roca et al., (Roca et al., 2005b) showed that the large amount of water vapour encountered in the Bay of Bengal area damps the long wave forcing of the deep clouds, leading to large and unopposed negative SW response, giving the net negative forcing, emphasizing the LW part of the spectrum. These studies recall the variety of factors influencing the observed radiative budget in the deep convective cloud regions of the Tropics. The relative role and the contribution of each of these elements need to be quantified in order to clarify the leading process at play from the scale of the individual convective systems up to the seasonal/interannual scale.
3.3 Precipitation estimation from satellite
Challenges around the precipitation estimation from space are numerous, ranging from new active instrumentation development to the optimized combination of multiple complementary observations, enhanced sampling through constellation deployment… Here we would like to emphasize a slightly different perspective. Indeed, the large variability of rain in space and time makes it hard to catch with any measurement device. Moreover, satellite and ground measurements are different by nature: a measure from satellite integrates rain in space (pixels) but a rain-gauge integrates rain in time (accumulation) (Bell and Kundu, 2003) making their comparison a delicate exercise. Fig. 7 shows a Hovmüller diagram of the rainfall estimation given by three different satellite products and ground validation datasets of a westward-propagating MCS passing over a one-degree square in the region of Kopargo (Benin) in July 2006 during the AMMA experiment. At this scale qualitative inter-comparison shows large differences among satellite and ground estimates as well as with the ground estimates and illustrates the difficulty of the validation effort. Methodological work needs to be further carried on in order to fully characterize the flaw in the satellite product and better exploit the ground based systems results.

Latitude-averaged Hovmüller diagrams over a (1°)2 box (1.5°E 2.4°E; 9.0°N 10.0°N) showing a westward-propagating MCS on July 28th 2006. From left to right, the Hovmüller diagram (a) is the top of atmosphere temperature (MSG 10.8um channel), the Hovmüller (b) is a sub-product of EPSAT-SG estimating rainfall probability (MSG resolution). Then (c) to (e) are the estimates of EPSAT-SG (Bergès et al., 2009b) at MSG resolution, GSMAP-MVK (Ushio et al., 2006) at 0.1° × 1 h resolution and TMPA (Huffman et al., 2007) at 0.25° × 3 hour resolution. Ground validation datasets used for (f) are rain-gauge interpolated fields (data courtesy of M. Gosset from LTHE), (g) used radar-estimated rain rates at 1.5 km of altitude (data courtesy of N. Viltard from CETP).
Diagramme de Hovmüller construit sur une boîte de (1°)2 (1.5°E 2.4°E; 9.0°N 10.0°N) montrant un amas convectif se propageant vers l’ouest le 28 juillet 2006. De gauche à droite, le diagramme représente (a) la température de brillance à 10.8 μm de MSG, (b) un sous-produit de EPSAT-SG correspondant à la probabilité de pluie (résolution MSG). De (c) à (e), il s’agit de la pluie au sol, estimée par EPSAT-SG (Bergès et al., 2009b) à la résolution MSG, GSMAP-MVK (Ushio et al., 2006) à la résolution de 0.1° × 1 heure et TMPA (Huffman et al., 2007) à la résolution de 0.25° × 3 heures. Les données sol de validation utilisées (f) sont celles des pluviographes (données par courtoisie de M. Gosset du LTHE) et (g) d’estimations radar à 1.5 km d’altitude (données par courtoisie de N. Viltard du CETP).
A second challenge concerning rainfall estimation from space lies in the better adequation between the end-users requests and the various satellite products. From an atmospheric physics perspective, rainfall is the result of the phase changes that occurred in the column and an indirect estimation of the associated latent heating of the atmosphere. The vertical distribution of the heating is the variable that forces the circulation (Tao et al., 2006). From a hydrological perspective, interest is set on the surface rainfall that acts as the input and the forcing of the continental water cycle (de Rosnay et al., 2007). These two major applications (not-mentioning meteorology and agriculture) have different constraints on the resolution and quality of the retrieval. Hence, more exchanges between the users and the satellite community are needed in order to optimize the retrieval development according to its final use and for instance, to avoid that a product suitable for hydrology is used unsatisfactorily by atmospheric community and vice-versa.
3.4 Observations of trends in the water and energy cycle
Given that the record of measurements for many elements of the water cycle (clouds, water vapour) and the radiative budget at the TOA in the Tropics dates back to the early 1980s and carries on up to nowadays, it is tempting to use those to derive climatic trends to infer any signature of climate change onto these cycles. Indeed, climate models are able to provide responses to scenario of greenhouse gas increase, which are interesting to confront, as much as possible, to the observational record. The satellite capabilities are well suited for such large-scale endeavour. Caution should nevertheless be exerted when going for such exercises. Previous attempts lead to endless discussions about the reality of the trends, like the discussions on the Advanced Microwave Sounding Unit (AMSU) temperature records. Recently, trends have been found in the tropical mean radiative budget (Wielicki et al., 2002) and found to be artificially due to a slow altitude drift of the satellite embarking the ERB non-scanning instrument (Wong et al., 2006). Similarly climate change-like trends were extracted from the HIRS record of UTH (Bates and Jackson, 2001) and were shown to be incorrect (McCarthy and Toumi, 2004). More recently investigations have proven the International Satellite Cloud Climatology Project (ISCCP) cloud records to be unfit for the long-term analysis of the climate (Evan et al., 2007). Hence, one of the important challenges facing the satellite community is to provide clean and exploitable geophysical records to document the recent evolution of climate and stick to good scientific practices. Recent careful investigations of the GPCP records recall that more time is needed before any potential of acceleration of the tropical water cycle stands out from the record noise (Gruber and Levizzani, 2008) but that the dataset is already useful to document some decadal variability (Gu et al., 2007) in the Tropics. Decadal variations and trends are indeed hardly detectable. In the case of the radiation budget, and in order to minimise the impact of the calibration uncertainty, Ben Rehouma et al. (Ben Rehouma et al., 2007) has inter-compared several data sources and focused on local anomalies in the trends. Over a part of the South East Atlantic including the marine low cloud area off Angola, they found a decrease of the yearly means of net flux estimated to 2.2, 3 and 6 Wm−2 respectively for three different datasets. The above examples recall that such exercise is intrinsically difficult. More methodological developments are needed to assess these temporal scales. Beyond enhanced work on the calibration of the sensors, extensive coupling between satellite and ground based measurements might be one way to focus on the robust features of climate change of the water and energy cycle (Held and Soden, 2006).
4 Supporting observations
Most of these challenges can only be achieved if both, the historical relevant archive is continuously well exploited and calibrated and continued with similar measurements and confronted to the results of the new observing capability. A few of these aspects are briefly discussed next.
4.1 The meteorological and research fleet
The meteorological fleet has been shown to provide useful (although difficult to manipulate) long time series on some key elements of the atmospheric water cycle. The new generation of operational satellites will continue these observations and provide complementary ones. MSG and MetOP are planned to observe continuously up to around 2018. Of particular interest are the infrared spectrometer (lASI and Atmospheric Infra Red Sounder/AIRS) that provide vertical profiles of water vapour relevant to the water and energy cycle (Gettelman et al., 2006; Pougatchev et al., 2009). Recent initiative at the European level will provide the suitable support for the long and complex processing of these data; and in particular the FTH series will be extended from the first to the second METEOSAT generation (Schulz and co-authors, 2008). In France, the Service d’archive de traitement météorologique des observations spatiales (SATMOS) (Cloché and Roca, 2007) and the more recent joint CNES-CNRS ICARE centre in Lille provide sound basis for the implication of the French laboratories in this heavy task.
The recent effort to fly a train dedicated to the global observations of the atmosphere using both active and passive sensors contributed to the enhancement of the remote sensing of the Tropics and of the water and energy cycles (Stephens and coauthors, 2002). For instance, Chung et al. (Chung et al., 2008) revealed new features of the deep tropical convective turrets while Bouniol et al. (Bouniol et al., 2008) highlighted, using airborne measurements during AMMA, the need to carefully handle the multiple scattering before using the satellite data. These better understood new observations will strongly contribute to deciphering the functioning of the tropical clouds.
4.2 The Megha-Tropiques mission
The MT mission (Desbois et al., 2007) is an Indo-French mission built by the Centre National d’Études Spatiales (CNES) and the Indian Space Research Organisation (ISRO) due to be launched in 2010. Megha means cloud in Sanskrit and Tropiques is the French for Tropics. The major innovation of MT is to bring together a suite of complementary instruments on a dedicated orbit that strongly improves the sampling of the water cycle elements. Indeed, the low inclination on the equator (20°) combined to the elevated height of the orbit (865 km) provides unique observing capabilities with up to six over-passes per day in the best case (Fig. 8). The scientific objective of the mission concerns:
- • atmospheric energy budget in the inter-tropical zone and at system scale (radiation, latent heat);
- • life cycle of Meso-scale Convective Complexes in the Tropics (over oceans and continents);
- • monitoring and assimilation for cyclones, monsoons, Meso-scale Convective Systems forecasting.

One week of the Megha-Tropiques orbit.
Une semaine de l’orbite de Megha-Tropiques.
These scientific objectives are achieved thanks to the following payload:
- • SCARAB: wide band instrument for inferring LW and SW outgoing fluxes at the TOA (cross track scanning, 40 km resolution at nadir);
- • SAPHIR: microwave sounder for water vapour sounding: six channels in the WV absorption band at 183.31 GHz. (cross track, 10 km);
- • MADRAS: microwave imager for precipitation: channels at 18, 23, 37, 89 and 157 GHz, H and V polarisations. (conical swath, < 10 km to 40 km).
The MT system does not fly an imager as the use of the geostationary platforms was envisioned to provide the needed infrared and visible information. Hence, the MT mission heavily relies upon the geostationary data for its full exploitation. The products related to precipitating cloudy conditions are derived from the MADRAS instrument: surface rain, cloud liquid water content profile, cloud ice water content profile, liquid and ice precipitation profile. The water vapour profiles are derived from a multi-instrumental approach (using MADRAS/SAPHIR/Geostationary instruments) for clear and cloudy conditions (non-precipitating) over lands and oceans. From this approach, other parameters are derived such as the total column water vapour, precipitable water, and surface temperature over land etc… The radiative fluxes derived from ScaRaB are the outgoing short wave and long wave fluxes. With the application of the angular distribution models (ADM), the radiometric calibration remains the most critical issue in these flux estimations. Many efforts were dedicated to both issues. The internal calibration module of ScaRaB-3 has been improved: the SW filter is now removable, which allows inter-calibrating of the main channels during the calibration modes. For the ADM issue, new neural network techniques have been developed in order to be consistent with the most accurate CERES models (Viollier et al., 2009). These instantaneous products are also averaged in space and time to obtain the daily and monthly regional means. The geostationary observations will be combined to MT observations for the tracking of MCS. Tracked systems will be matched to overpasses of the MT satellite, and then a composite of the evolution of precipitation will be performed during the life cycle of the systems as exemplified in section 3. The MT observations combined with the geostationary data will lead to a more efficient space-time sampling, useful for precipitation estimate. The objective is then to derive precipitation products from different sensors (geostationary sensors, microwave sensors onboard MT) and consequently to use in combination with MT all the available geostationary data on the entire tropical belt.
MT is involved in the Global Precipitation Mission (Hou et al., 2009), a constellation of multiple microwave radiometers to be calibrated thanks to a dual-frequency rain radar on board a core satellite. The combined MT products will be extended to these multiple platforms to benefit from the expected enhanced sampling.
5 Conclusions
In the Tropics, the non-linear interactions between the water and the energy cycle are fully expressing their complexities. The process at play spans a wide range of scales, from individual cloud systems to climatic trends, which makes its study, a challenge. Satellites provide the best tool to observe the water and energy cycle in the intertropical belt but their manipulation should be carried out with caution. While most of the geophysical parameters are directly related to the measured radiances, some are only very indirectly related to the observations. Hence, a careful investigation of the quality of the retrieval through extensive validation efforts is required to qualify the products at the suited scale. The application of the satellite products (e.g., rainfall for hydrology) requires a dedicated effort to bridge the interdisciplinary gap. Long-term trends are difficult to obtain from a series of individual spacecrafts and the inter-calibration effort already initiated should be improved and continued before archives can be used to confidently reveal the evolution of the water and energy cycle. The new generation of meteorological satellites, together with the experimental research fleet opens a new and exciting era that will contribute to a better understanding of the water and energy cycle in the Tropics.
Acknowledgments
The congenial support of the Climserv team and of K. Ramage is acknowledged. Thanks are given to the Groupe Modèle du LMD for many fruitful discussions on the modelling of the tropical circulations and the water and energy cycle. The authors also want to thank R. Kandel for insightful discussions on the radiation budget and climate change. These acknowledgments extend naturally to the French science team of the MT project. Special thanks to Michel Desbois for having initiated most of this scientific activity over the last 30 years.