1 Introduction
Precipitation is one of the most important variables in the global hydrological cycle, for meteorology, climate and hydrology applications. It also plays a key role in socio-economic activities such as agriculture. The largest impact of future climate changes on the society will likely come from changes in precipitation patterns. Precipitation is characterized by a high variability in space, time and intensity. Convective events can have rain rates above 50 mm/h, last less than 30 min to up to 6 h, with individual cells as small as 4 km in diameter whereas stratiform systems with low rain rates can have life cycles of few days and cover up to 1000 km × 1000 km. In addition, precipitation can have different forms, liquid or frozen (hail, snow). At any time, the area of the globe covered affected by precipitation is only a few percent (around 3%) from very light rain rates to 125 mm/h.
Historically, precipitation has been observed locally by rain gauges, often only as accumulated daily amounts. The global distribution of gauges is quite variable, from relatively dense networks in the developed countries to sparsely distributed gauges in developing regions. Over oceans, gauges are almost non-existent, with only a few of them on islands. The accuracy of gauges depends upon several factors, such as design, precipitation phase (liquid or frozen), wind effects, evaporation/condensation, or surface topography.
Ground-based weather radars are increasingly combined into national and international networks to provide spatial measurements of precipitation (of the order of 100 km from the radar) with high temporal sampling. However, radars are essentially available over land, in regions that are usually well covered by rain gauges. In addition, conversion of the radar reflectivity at a certain altitude and distance from the radar into a quantitative precipitation estimation is difficult. Issues include drop size distribution, melting precipitation, low-level precipitation, attenuation, surface effects, beam blockage, or beam-filling problems.
The needs for precipitation observations were recently assessed by a EUMETSAT expert group (Rizzi et al., 2006) and are presented in Table 1. They cover meteorological applications, both Numerical Weather Prediction (NWP) and NoWCasting (NWC), as well as hydrology and climate, for 2020 onwards. Conventional precipitation measurements, from gauges or, more recently, from radar, will clearly not satisfy these requirements on a global basis.
Les besoins des utilisateurs en terme de précipitations liquides, basés sur les recommandations de EUMETSAT (Rizzi et al., 2006).
Parameter | Application | Accuracy (r.m.s.) | |||
Unit | Threshold | Breakthrough | Objective | ||
Precipitation profile (liquid) | NWP global | % | 100 | 50 | 20 |
NWP regional | % | 100 | 50 | 20 | |
Precipitation rate at surface (liquid) | NWP global | % | 100 | 50 | 20 |
NWP regional | % | 100 | 50 | 20 | |
Climate | mm/h | 10 | 5 | 2 | |
Hydrology (>10 mm/h) | % | 20 | 10 | 5 | |
Hydrology (1–10 mm/h) | % | 40 | 20 | 10 | |
Hydrology (<1 mm/h) | % | 80 | 40 | 20 | |
Precipitation detection (liquid) | NWP global | HR/FAR | 50/50 | 95/10 | 99/2 |
NWP regional | HR/FAR | 50/50 | 95/10 | 99/2 | |
NWC | HR/FAR | 50/50 | 70/40 | 85/20 | |
Precipitation Type | NWP global | Classes | 3 | 4 | 6 |
NWP regional, NWC | Classes | 3 | 4 | 6 | |
Climate | Classes | 3 | 4 | 6 |
Space-borne remote sensing of precipitation is a solution, provided that high spatial resolution, rapid revisiting time and adequate accuracy can be achieved. Very early, the use of visible and infrared satellite data was suggested, “though not indicating precipitation totals directly”, to map the rainfall distribution “more acceptably” than using surface stations (Barrett, 1970). Since then, a large number of satellite sensors have been developed and launched to observe the atmosphere. During the last decade, satellite instruments have been specifically designed for precipitation monitoring, such as the instruments on board the Tropical Rainfall Measurement Mission (TRMM).
Precipitation estimates from satellites are based upon observations in the visible/infrared or in the microwave. Measurements in the visible and infrared domains provide information only on the top of the cloud that generates the rain and the precipitation estimate is thus indirect. However, these measurements are available from geostationary orbits, with quasi-continuous monitoring of a larger part of the Earth, with up to 15 min sampling. Observations from low orbits have a better spatial resolution but are available usually only twice a day for a given location and a given satellite. Microwave measurements are more directly sensitive to the precipitation but they are so far only available from low orbit satellite. Both passive (radiometer) and active (radar) microwave observations are today available from low orbits for precipitation estimates, with radar observations providing a better horizontal and vertical resolution but over a more limited area.
Fig. 1 shows Hurricane Katrina, August 28, 2005 in the Gulf of Mexico as observed by TRMM with infrared (a), passive microwaves (b), and active microwaves (c–e). Each observation type provides different information on the cloud and rain system that can complement each other.
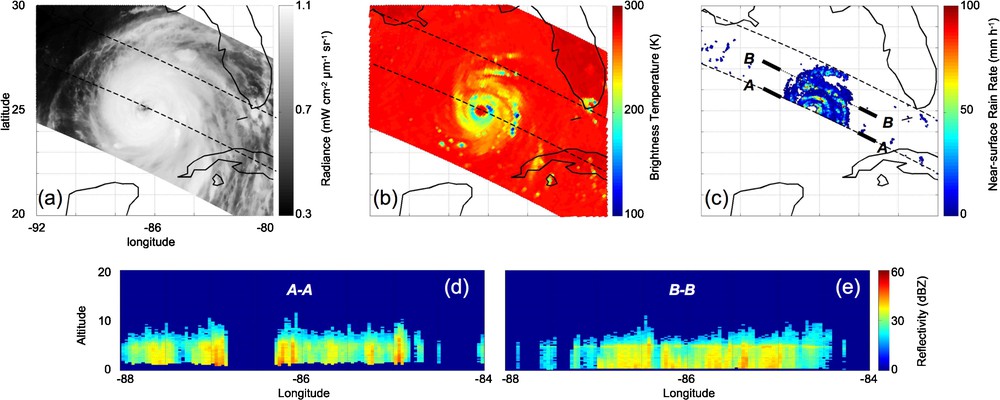
Hurricane Katrina in the Gulf of Mexico August 28, 2005 at 3:20 UTC, as observed by TRMM. (a) 12 μm channel from VIRS; (b) 85 GHz brightness temperature in vertical polarization from TMI; (c) near surface rain rate from PR; (d and e) vertical cross-sections of the PR reflectivity along A–A and B–B transects shown in (c).
L’ouragan Katrina dans le golfe du Mexique le 28 août 2005 à 3:20 UTC, comme l’a observé TRMM. (a) 12 μm de VIRS ; (b) température de brillance à 85 GHz en polarisation verticale de TMI ; (c) taux de pluie à la surface, donné par le radar PR ; (d et e) coupes verticales de la réflectivité radar entre A–A et B–B tels que indiqués en (c).
A recent book “Measuring precipitation from space” (Levizzani et al., 2007) gave an overview of the present and future developments in precipitation monitoring from space. Stephens and Kummerow (2007) also provide a summary of the retrieval methods to remotely sense cloud and precipitation from space. In the present article, a summary of the techniques, results and perspective is given. Section 2 focuses on the IR methods, Section 3 on the passive microwave ones and Section 4 concentrates on the space-borne radar observations. The Global Precipitation Climatology Project and the TRMM mission are summarized in Section 5 and Section 6 presents the perspectives.
2 Precipitation monitoring from visible/infrared satellite observations
Different techniques have been developed to estimate precipitation from visible and/or infrared satellite images. They are all based on the fact that cold/bright clouds are related to convection and are likely to produce rain. The rainfall at the ground is thus indirectly derived from the observations of the cloud top from the satellite, essentially based on the relationship between IR cloud top temperature and the rainfall probability and intensity at the ground. These methods have been applied to a large range of scales and for a variety of applications since the 1970s, including operational meteorology. They benefit from the availability of the visible/infrared observations from geostationary satellite, providing the adequate temporal sampling for the observations of the short duration and high temporal variability of rain.
The Griffith-Woodley Technique (Griffith et al., 1978) is an empirical scheme to estimate convective rainfall from the Geostationary Operational Environmental Satellite (GOES) infrared images at 11 μm. The method requires a time sequence of cloud area, measured by the satellite at a specific threshold of brightness or temperature to calculate rain volume. The scheme is calibrated with gauge-adjusted radar. It was later extended to mid-latitudes (Griffith, 1987), using outputs from a one-dimensional cloud model. The method was examined by Negri et al. (1984) who concluded that a simpler algorithm without the need for the cloud history could produce similar results. The Convective-Stratiform Technique of Adler and Negri (1988) defines convective cores by locating the local minima in the cloud top temperature fields and assigns rain rate to each one, with the help of a temperature–rain relationship derived from a cloud model. The associated stratiform rainfall region is also identified.
However, these methods encounter difficulties in the detection and quantifications of warm rain processes that are very frequent at mid-latitudes (e.g. Ebert et al. (2007)). Such precipitating clouds are characterized by relatively warm and spatially homogeneous cloud top temperatures, with little differences between the raining and non-raining areas. As a consequence, retrieval techniques based solely on IR cloud top temperature lead to an underestimation of the detected rain areas and rates in these cases. To overcome these problems and improve the rainfall retrieval at mid-latitudes, the use of optical and microphysical cloud parameters derived from multi-spectral visible/infrared data of new generation sensors has been suggested (e.g. Lensky and Rosenfeld (2003) ; Nauss and Kokhanovsky (2006)). The Spinning Enhanced Visible and Infrared Instrument (SEVIRI) on board the Meteosat Second Generation (MSG) offers potential for a better characterization of clouds by means of improved infrared calibration, radiometric performances, multi-spectral information (12 channels), high spatial (3 km at sub-satellite point) and temporal resolution (15 min for the full disk). The enhanced spectral resolution enables the retrieval of optical and microphysical cloud properties that contribute to differentiate various precipitation processes and rainfall intensities within a given area (Thies et al., 2008). Applications of these techniques are particularly valuable for nowcasting, thanks to the high temporal and spatial sampling of the MSG observations.
3 Precipitation estimation from passive microwave observations
Contrarily to visible and IR observations that can only sense the cloud upper layer, passive microwave observations from satellite sound inside the clouds and rain, at least partly (compare panels a and b in Fig. 1). Hydrometeors can cause measurable changes on the microwave radiances observed from satellites, making it possible to quantify clouds and precipitation. Depending on the observed wavelength and the hydrometeor characteristics, microwaves are affected by emission, absorption, and scattering, and the effect strongly increases with frequency. Due to the large imaginary part of the refractive index of liquid water in the microwave range, liquid particles significantly absorb and emit microwave radiation. On the contrary, with their small imaginary refractive index, ice particles only interact by scattering, when the ratio between the particle sizes and the observation wavelength is large enough. The possibility of remotely sensing cloud and precipitation from microwave radiation relies on the understanding of the interaction between the radiation and the hydrometeors. The microwave radiative transfer in scattering atmospheres has been recently reviewed in depth by Battaglia et al. (2006). The main simulation difficulties come from the lack of information on the particle spatial distribution and physical properties (size, shape, refractive index), especially in the ice phase.
At frequencies below 50 GHz, the microwave signal is essentially sensitive to the emission–absorption by liquid clouds and rain and is little affected by the presence of ice clouds aloft. At higher frequencies, scattering effects on frozen particles increase, eventually masking the liquid cloud and rain signal observed from satellite when sufficient ice quantities are present at the top of the cloud.
During the emission–absorption process, liquid particles cause brightness temperatures to increase over a radiatively cold background like the ocean; the induced warming of the signal increases with frequency. Precipitation retrievals over ocean are often based on the emission signals measured at frequencies below 40 GHz, which are the most sensitive to the rain itself (Wilheit et al., 1977; Prabhakara et al., 1992). Over land, the emission signal from the cloud and rain is more difficult to observe, land surface emissivity being high. When frozen particles are large enough with respect to the wavelength, scattering reduces the amount of radiation measured by the satellite. The emission signal originates in the liquid lower part of the cloud and rain, whereas the scattering in the upper part of the cloud modulates the emission signal, especially at high frequencies. The scattering signal at 85.5 GHz has been used to estimate precipitation over ocean and land, for example by Spencer et al. (1989) or Grody (1991). Scattering methods are an indirect measurement of precipitation, relying on the implicit relationship between the presence of large particles aloft and the precipitation below, but have the advantage of being potentially applicable over all surfaces, including land and coasts.
Current microwave instruments used for precipitation monitoring involve the Special Sensor Microwave/Imager (SSM/I) on board the Defense Meteorological Satellite Program (DMSP), the Advanced Microwave Scanning Radiometer (AMSR) on Aqua, and the TRMM Microwave Instrument (TMI). They all have a ∼50° scanning angle and observe in window channels below 90 GHz. Table 2 summarizes the main characteristics of current microwave instruments on board meteorological satellites.
Les instruments micro-ondes aujourd’hui à bord des satellites météorologiques.
Instrument | Platform | Time coverage | Spectral range (GHz) and polarization | Scanning type | Spatial resolution | Main objectives |
SSM/I nsidc.org/data/docs/daac/ssmi_instrument.gd.html | DMSP | Since 1987 | 19VH, 22V, 37VH, 85VH | Conical scanning (53°) | From 60 to 15 km | Integrated H2O, cloud and rain, surface properties |
SSMI/S http://www.osdpd.noaa.gov/PSB/IMAGES/ssmisdoc.htm | DMSP | Since 2003 | 19VH, 22V, 37VH, 50–59H (7 channels), 91V, 150H, 183H (3 channels) | Conical scanning (53°) | From 60 to 15 km | H2O and T profiles, cloud and rain, surface properties |
TMI trmm.gsfc.nasa.gov/overview_dir/tmi.html | TRMM | Since 1997 | 10VH, 19VH, 21V, 37VH, 85VH | Conical scanning (53°) | From 50 to 6 km | Integrated H2O, cloud and rain, surface properties |
AMSR-E aqua.nasa.gov/about/instrument_amsr.php | NASA/Aqua | Since 2002 | 7VH, 11VH, 19VH, 24V, 37VH, 85VH | Conical scanning (54°) | From 50 to 5 km | Integrated H2O, cloud and rain, surface properties |
AMSU-A aqua.nasa.gov/about/instrument_amsu.php | NOAA NASA/Aqua ESA/MetOp | Since 1998 | 23, 33, 50-57 (12 channels), 89 | Cross-track scanner (±50°) | ∼45 km at nadir | T profiles |
AMSU-B http://www2.ncdc.noaa.gov/docs/klm/html/c3/sec3-4.htm | NOAA | Since 1998 | 89, 157, 183 (3 channels) | Cross-track scanner (±50°) | ∼15 km at nadir | H2O profiles |
MHS daac.gsfc.nasa.gov/AIRS/documentation/hsb_instrument_guide.shtml#2. | NASA/Aqua ESA/MetOp | Since 2003 | 150, 183 (3 channels) | Cross-track scanner (±50°) | ∼15 km at nadir | H2O profiles |
The first rain retrieval methods were simple regressions between surface rain rates and their associated simulated or measured brightness temperatures (e.g., Wilheit et al. (1977)). These approaches are still used for long-term climatologies. More recent retrievals (e.g., Kummerow et al. (1996), Kummerow et al. (2001), Bauer et al. (2001), Bauer (2001)) are based on probabilistic techniques, and rely upon data sets of well-documented and physically meaningful situations, usually obtained from the coupling of cloud resolving models and radiative transfer calculations (e.g., Smith et al. (1992), Mugnai et al. (1993) and Kummerow and Giglio (1994)). Iterative techniques based on radiative transfer models have also been developed (e.g., Wentz and Spencer (1998)). Most of these algorithms were originally developed for retrieval of rain over ocean but some of them also have an over-land module that relies on the scattering at 85–89 GHz by the ice clouds above the rain. Inter-comparisons and evaluations of the different rain algorithms are conducted (SPIP, 1998).
At frequencies above 100 GHz, the measurements are not expected to directly sense the rain profile close to the ground: cloud opacity in the precipitating areas limits the amount of up-welling radiation from the rain. The Advanced Microwave Sounding Units (AMSU) on board the NOAA polar orbitors are temperature (AMSU-A) and humidity (AMSU-B) cross-track sounders. They observe in the O2 band around 55 GHz (AMSU-A) and around the H2O 183 GHz line (AMSU-B) (see Table 2). Correlations between precipitation and observations arise from indirect relationships between the cloud, the atmospheric profile and the precipitation below. Rain rates have been estimated from AMSU-B observations over ocean and land, from simple scattering index based on the differential scattering in the clouds at 89 and 150 GHz (e.g., Ferraro et al. (2005)). Neural network schemes are developed to retrieve precipitation rates from AMSU-A and -B observations, using training database generated from collocated observations from AMSU and radar (Staelin and Chen, 2000) or from simulations derived from the coupling of mesoscale cloud models and radiative transfer codes (Defer et al., 2008).
The estimation of snowfall is a recent field of challenging research, with the difficulties first to model accurately the scattering by snow and second to discriminate between the falling snow and the strongly variable emissivity of the snow on the ground. Physical approaches are attempted to retrieve snowfall rate over land using observations above 100 GHz (e.g., Kim et al. (2008)).
Until recently, only microwave satellite observations under clear sky conditions were assimilated in Numerical Weather Prediction schemes in operational meteorological centers. An elaborated variational assimilation scheme has been tested at European Center for Medium-range Weather Forecast (ECMW) (Moreau et al., 2003) and is currently implemented in operational mode (Bauer et al., 2006a, b).
4 Active microwaves for precipitation measurements
The use of active microwave observations from satellites for precipitation retrieval started in November 1997 with the launched of the TRMM mission with the first space-borne Precipitation Radar (PR) (Kummerow et al., 2000). The TRMM mission with its suite of instruments will be briefly presented in the next section. The PR transmits energy at 13.8 GHz and measures the power reflected by the rain particles and the Earth's surface. It has a 215 km swath, a horizontal footprint diameter of 4.3 km and a pulse range corresponding to a 250 m vertical resolution. The instrument is regularly calibrated to better than 0.5 dBZ and has shown an excellent stability for the last 10 years. Although its swath is relatively narrow and it suffers from the same uncertainties for precipitation retrieval as do ground-based radars, it captures the three-dimensional rain structure with horizontal and spatial resolutions that were never reached before (see panels d and e in Fig. 1). Different precipitation algorithms have been derived (e.g., Iguchi et al. (2000)). To obtain accurate three-dimensional rain structure, it is necessary to correct for the attenuation effects before radar reflectivities are converted into rainfall rates, because the signal at 13.8 GHz suffers from attenuation. The rainfall rate R is estimated then from the reflectivity Ze using a power law (R = aZeb) in which the parameters can be a function of rain type, height of the freezing level, or storm top. The presence or absence of the bright band is also accounted for. The critical steps in the algorithms include attenuation correction, selection of the drop size distribution model and the correction for non-uniform beam-filling effects.
Unique information has been obtained for the first time from the TRMM precipitation radar, such as latent heat profiles at a global scale (Tao et al., 2006), evidence for the suppression of rainfall by smoke-contaminated clouds (Rosenfeld, 2000). In addition, it played a critical calibration role, in the development of passive microwave rainfall retrieval, as well as in the cross-comparison of precipitation ground-based radars.
Cloud particles measured in non-precipitating water and ice clouds are rather weak scatterers of microwave radiation. The need to detect the weak cloud signals imposes constraints on the minimum detectable reflectivity as well as on the frequency of operation (the higher the frequency, the more sensitive to the scattering). The CloudSat Cloud Profiling Radar (CPR), launched in August 2006, operates at high frequency (94 GHz) and makes it possible to investigate the vertical structure of both clouds and rain (Stephens et al., 2002, 2008). It observes the Earth with a fixed angle close to nadir. The vertical resolution is ∼500 m and the spatial resolution is 1.4 km. The minimum sensitivity (−30 dBZ) required from CloudSat radar represents almost a five orders of magnitude more sensitivity than the TRMM PR. In orbit calibrations are performed regularly, in addition to pre-launched measurements. The CloudSat spacecraft flies in formation as part of a constellation of satellites (the A-Train). At 94 GHz, the attenuation by gases (essentially water vapor), liquid water droplets, and precipitation-size particles is not negligible, and can be exploited, especially to detect and estimate precipitation intensity.
The EarthCARE mission (Lajas et al., 2008) is to include a similar radar to CloudSat but with additional capabilities for providing Doppler motion measurements.
5 Combination programs
No existing technique so far provides all the spatial, temporal and accuracy features requested by the user community for precipitation monitoring. Table 3 summarizes the benefits and limitations of the various satellite techniques. Different solutions have been developed to overcome the limitations of a given technique. For example, to resolve the sampling problem due to the low orbits of the current microwave sensors, passive microwave observations and IR geostationary measurements have been combined: the microwave rain retrieval is used as a reference and the IR images provide the almost continuous measurement to follow the evolution of the systems (e.g., Sorooshian et al. (2002) ; Tapiador et al. (2004)). In this section, two international programs dedicated to precipitation monitoring and combining different techniques are briefly presented, along with their results. The Global Precipitation Climatology Project is an organized effort to merge multi-instrument observations from various satellite platforms and rain gauge measurements to provide a long time record of global precipitation. At a mission level, the TRMM combines five precipitation sensitive instruments on board the same platform.
Avantages et limitations des différentes mesures de précipitation.
Rain gauge | Point measurement, type-dependent accuracy, no ancillary information on rain type etc. available, measures rain accumulation (possibly DSD), operational. |
Surface radar | Limited volume measurement, measures Z (possibly polarization, Doppler-spectrum), operational, accuracy of RR-retrieval depends on rain gauge calibration. |
Satellite visible/infrared | Available from geostationary satellite with good temporal sampling, high spatial resolution Indirect estimates |
Satellite passive microwave radiometer | Wide swath measurement, calibration good, measures TB that is sensitive to T, q, hydrometeors, (surface emissivity,) channel selection rather flexible, operational. |
Satellite radar | Profile/narrow swath measurement, calibration rather good, measures Z, experimental. |
5.1 The Global Precipitation Climatology Project (GPCP)
The goal of GPCP is to produce a climatology of global precipitation, to improve understanding of seasonal to inter-annual variability of the global hydrological cycle, determine the atmospheric latent heating rates, and provide an observational data set for model validation and initialization. It merges microwave and infrared satellite observations as well as rain gauge data and takes advantage of the strengths offered by each type of measurement: unbiased estimates where rain gauge data are available, physically-based microwave rain rates estimated from low orbit satellites, and high temporal resolution indirect estimates from visible/infrared sensors on geostationary satellites (Huffman et al., 1995; Adler et al., 2003). GPCP now provides an ongoing record of almost 30 years of global monthly precipitation with a 2.5° × 2.5° spatial resolution. For the recent years, 1° × 1° daily products are also available. Detailed analyses have been conducted to inter-calibrate and merge the different types of measurements, and great care was taken to provide the most consistent and complete precipitation analyses that the satellite and in situ data allow. However, the impact of changes in sensor characteristics along with the decrease in rain gauge measurements during the span of the project should not be underestimated. Careful estimates of the precipitation uncertainties are provided. An extensive description and assessment of GPCP have been recently published (Gruber and Levizzani, 2008). More information is available from http://precip.gsfc.nasa.gov/. The mean annual GPCP precipitation over 1979–2002 is shown in Fig. 2 (from Gruber and Levizzani (2008)).

Mean annual GPCP precipitation over 1979–2002 in mm day−1 (Gruber and Levizzani, 2008).
Precipitations moyennes données par GPCP entre 1979–2002 en mm j−1 (Gruber and Levizzani, 2008).
The GPCP datasets have provided an opportunity to study the global precipitation patterns and their variability in ways that were not possible before. For instance, Fig. 3 shows the interseasonal variability of the precipitation over the globe for land and sea separately (from Gruber and Levizzani (2008)). A 5.5% precipitation increase is observed over ocean and a 1% decrease over land during the 1979–2005 year time period of GPCP, but these trends have to be taken with caution (Gu et al., 2007). Despite the long time record now available, analysis of the global precipitation trend is still difficult, with strong contaminations of the precipitation inter-annual variability by the El Nino Southern Oscillation (ENSO) signatures.

Global averages of monthly precipitation (mm day−1) for ocean (blue), total (black) and land (green). Heavy lines indicate running mean (Gruber and Levizzani, 2008).
Moyennes globales mensuelles des précipitations (mm j−1) au-dessus de la mer (bleu), au total (en noir) et au-dessus des terres (vert). Les traits pleins indiquent la moyenne courante (Gruber and Levizzani, 2008).
5.2 The Tropical Rainfall Measurement Mission
TRMM is a collaborative mission between NASA and the Japanese spatial agency (JAXA). It is on a low non-sunsynchronous orbit (350 km initially, now at 400 km) that covers the diurnal cycle within the 35°S–35°N region. Two thirds of global rainfall occurs in these tropical–sub-tropical areas, with the latent heating resulting from precipitation controlling the large-scale general circulation. It initially carried five rain-measuring instruments, described in Kummerow et al. (1998). In addition to the microwave radiometer (TMI) and the precipitation radar (PR) that have already been described (Sections 3 and 4), it contains a 5-channel Visible and InfraRed Scanner (VIRS) and a Lightning Imaging Sensor (LIS) used to optically detect the lightning flash rate. A last instrument, the Cloud and Earth's Radiant Energy System (CERES) was to investigate the Earth's radiation budget and the impact of clouds on net radiant energy, but it failed shortly after the launch. The sensors observe simultaneously for the first time different aspects of the precipitation systems, leading to very valuable information on the cloud and rain processes.
Many applications make use of the observation combinations. The PR is especially important to measure precipitation over land, where passive microwave measurements have difficulty because of a poor contrast between the generally radiometrically warm precipitation signals and the highly emissive land surface. The swath width of the TMI is more than three times that of the PR (∼780 km versus ∼220 km) making it possible to measure precipitation over a wide-swath track with TMI, while the PR measures the detailed physics of precipitation along the narrow-swath radar track in coincidence with the inner-swath radiometer track, and possibly calibrates the TMI estimates (Viltard et al., 2006). Combination of PR and TMI data with the Lightning Imaging Sensor (LIS) also resulted in a better understanding of global precipitation systems (Cecil et al., 2005; Nesbitt et al., 2000).
A review of the TRMM mission, benefits, and outcomes is provided in the TRMM Senior Review Proposal (TRMM, 2007) and a list of publications derived from TRMM is available at http://trmm.gsfc.nasa.gov. The TRMM contributions cover a large range of domains, including climatology studies (rain, latent heating profiles, lightnings, cloud systems, along with their diurnal cycles), hydrological applications and operational use in Numerical Weather Prediction centers. Fig. 4 shows an example of comparison of latitudinal distribution of zonaly averaged annual precipitation, from observational analyses (TRMM, GPCP, CMAP) and nine Global Circulation Models (from Dai (2006)). The observation analyses are rather close whereas the model simulations tend to differ significantly, especially in the Tropics.

Latitudinal distribution of zonally averaged annual precipitation, for observational analyses (TRMM, GPCP, CMAP) and nine Global Circulation Models (Dai, 2006).
Distribution latitudinale moyenne des précipitations annuelles, donnée par les observations (TRMM, GPCP, CMAP) et par neuf modèles de circulation (Dai, 2006).
The TRMM mission was initially planned for 3 years. It has now provided data for over a decade and the mission could be extended up to 2012.
6 Future challenges
During the last decade, satellite observations allowed significant advances in our understanding of the distribution and quantification of precipitation, especially with the contribution of the TRMM mission. Observations at different wavelengths (visible, infrared and microwaves), potentially in passive and active modes (microwave radiometers and precipitation radar), and from both low and geostationary orbits were analyzed and eventually coupled to produce records of precipitations estimates over the globe, for long time period, and with up to an hourly sampling.
However, despite the large variety of satellite observations now available, the requirements of the different communities in terms of precipitation estimates (Table 1) are still not met. Main challenges concern the need for high temporal sampling, high spatial resolution, and the possibility to detect light rain rates and quantify falling snow. Assimilation of precipitation estimates into Numerical Weather Prediction scheme is only in its infancy and now-casting of precipitation during extreme weather events will not happen soon. The International Precipitation Working Group (Turk and Bauer, 2006) is sponsored by the World Meteorological Organization (WMO) and focuses on the development of satellite precipitation measurements for operational and research applications. It encourages the synergies between the different observation types for more accurate instantaneous rainfall estimations at all latitudes. It has a key role in the development of future sensors and missions.
The main international project in the field of precipitation is the Global Precipitation Measurement (GPM) mission, planned for a launch in 2013 (Smith et al., 2007). It aims at improving and extending the TRMM measurements to higher latitudes (up to 65°), with a more frequent sampling (every 3 h), a higher sensitivity to light and heavy rainfalls (from 0.1 cm to more than 20 cm/h), and a possibility to discriminate between rain and snow. GPM is a constellation of satellites (up to height) with a core satellite equipped with a precipitation radar and microwave radiometers, and the other satellites having mainly passive microwave sensors on board. The core satellite, jointly developed by NASA and JAXA, will fly both a Dual-frequency Precipitation Radar (DPR) and a high-resolution, multi-channel passive microwave rain radiometer, the GPM Microwave Imager (GMI). The radar is planned to operate at 13.6 GHz and 35.5, to infer information on both rain and cloud, including size of the precipitation particles, and information on solid precipitation. At high frequencies, higher order of scattering may be significant in the radar echo, with consequences that will have to be accounted for in the processing (e.g., increase of the optical thickness, decrease of the mean free path). These effects will have to be taken into account into the observations processing. Fig. 5 illustrates the GPM concept with the core and the constellation satellites. A mission such as the French-Indian Megha-Tropiques (Desbois et al., 2007) could be part of the GPM constellation.

The GPM concept, with the core and the constellation instruments (Gruber and Levizzani, 2008).
Le concept GPM, avec le satellite principal et les instruments secondaires (Gruber and Levizzani, 2008).
High sampling intervals of precipitation are particularly crucial in the follow-up of severe weather events. Current visible and infrared observations from geostationary orbit do not provide accurate quantification of the rain rates. So far, both passive and active microwave observations are only available from low-orbit satellite.
Geostationary orbits provide good time sampling but deploying passive microwave sensors in high orbit with the adequate spatial resolution imposes the use of shorter wavelengths or the use of interferometric technique. The spatial resolution is proportional to λ/D (with λ the wavelength and D the antenna diameter). To obtain spatial resolution of 50 km at 50 GHz, an antenna of 4 m is required. Millimeter/sub-millimeter radiometers are thus suggested to observe precipitation with an adequate spatial resolution while keeping reasonable antenna size. The potential of millimeter/sub-millimeter observations for precipitation monitoring has been evaluated (Staelin and Surussavadee, 2007; Defer et al., 2008), showing very good promises for rain rates above 1 mm/h over both ocean and land. For instance, the Geostationary Observatory for Microwave Atmospheric Sounding (GOMAS) project has been submitted to the European Space Agency, with channels between 50 and 425 GHz and ∼3 m antenna, providing a spatial resolution of ∼10 km at 400 GHz (Bizzarri et al., 2005). The other solution consists in using a synthesized aperture. Such a challenging solution is currently studied at JPL, the GEOSTAR project, with a 2D spatial interferometric system at 50 GHz, with three arms, each one with roughly 100 receivers at each observed frequency (Lambrigtsen et al., 2004).
Active microwave observations from geostationary orbits are also planned. Nexrad-In-Space is studied at NASA. It is a geostationary satellite Doppler weather radar at 35 GHz equipped with a 35-m deployable antenna, to provide vertical and horizontal velocities and precipitation intensities, with a 12-km resolution over a region of approximately 5200 km in diameter (Tripoli et al., 2007).
Acknowledgments
The author wants to thank Eric Defer for providing Fig. 1 and for proofreading the manuscript.