1 Introduction
In natural lakes, thermal stratification of the water column leads to the formation of water layers (Wetzel, 1983) allowing stable chemical conditions to develop. In reservoirs, thermal and chemical stratification of the water column is influenced by river inflows (Han et al., 2000) and outflows (Casamitjana et al., 2003; Fontane et al., 1981). Reservoirs are generally built in areas where river inflow is sufficiently high that they can be filled rapidly. The impact of the river inflow depends on its volume and on its penetration depth. This depth is controlled by density differences between the river and the reservoir waters, including differences in temperature and in dissolved and particulate load, as well as by the geometry of the banks and of the reservoir slope at the inflow location. As a river enters a reservoir, it pushes ambient water ahead of itself until buoyancy forces arrest the flow. If the incoming water mixes thoroughly with the upper layer or its density is less than that of the ambient, then the intrusion layer flows across the surface of the reservoir. Atmospheric conditions (temperature, wind, rain) may control the longitudinal dispersion and mixing of this layer with the reservoir water. If the inflow mixed water is denser than the reservoir surface, it will plunge under the surface and create a density current. If the density current is created, it will entrain water from the passing depths of the reservoir water until finding a level of neutral buoyancy to form an underflow in that layer depth, or will continue to the reservoir bottom down the reservoir slope. Mixing of reservoir waters with the inflow occurs in the region of the plunge (initial mixing) and after the flow has formed an intrusion layer. At the end of the reservoir basin, the outflow creates a current at the depth of the sluice, varying according to hydroelectric demand. There are thus two potential layers, one entering upstream at the top of the reservoir and one at the bottom of the water column, at the level of the sluice, with some thickness, sometimes not negligible if compared to the height of the water column. These layers, combined with, and involved in, the thermal and chemical stratification, control the ecological and geochemical behaviour of the reservoir (Bonnet and Poulin, 2002, 2004; Hamilton and Schladow, 1997; Lopes et al., 2010; Rueda et al., 2007; Søballe and Kimmel, 1987).
The Bicaz reservoir (Izvorul Muntelui-Bicaz, here called Bicaz), located in a mountainous area in the East Carpathians, Romania (Fig. 1), was built to supply hydroelectric power. The watershed of the Bistriţa River, its main tributary, comprises numerous manganese and polymetallic sulfide ore deposits. A geochemical study of the Bistriţa River (water and sediments) and the Bicaz reservoir has been undertaken to assess the behaviour of trace elements originating from the weathering of ore deposits.
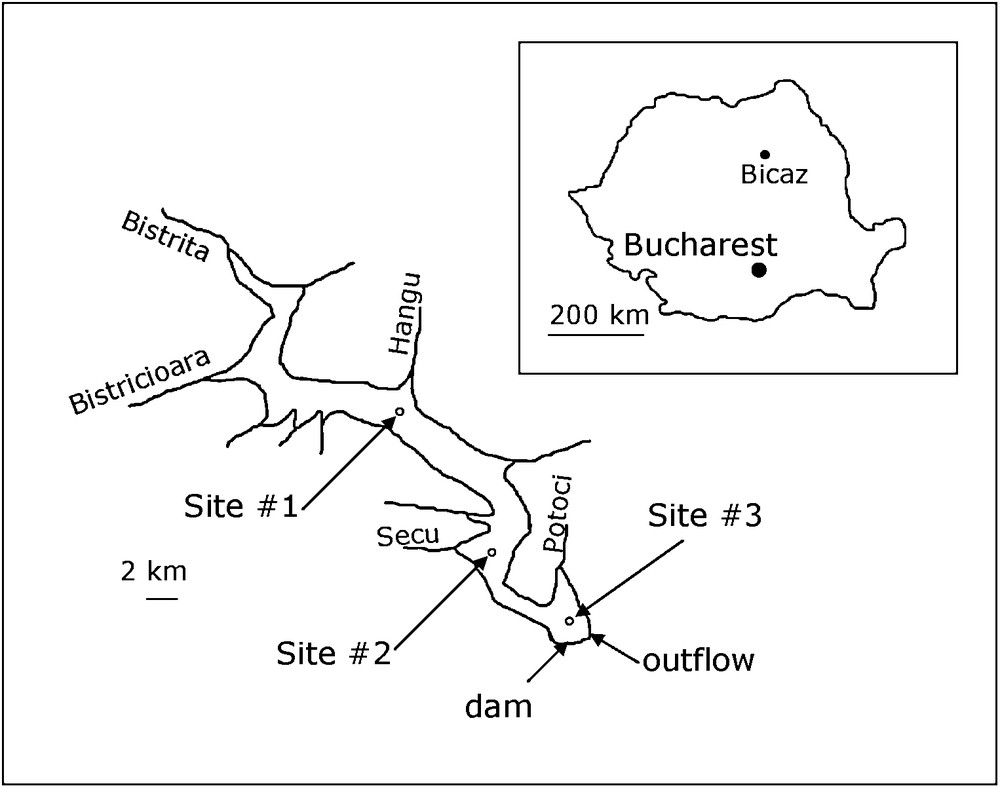
Location of the Bicaz reservoir and the data collection sites.
Localisation du lac de barrage de Bicaz et des points de prélèvement.
The water quality of reservoirs is controlled by the origin and quality of the entering waters (tributaries of the reservoir) and advection, convection and dispersion processes occurring in the water column. In the case of the Bicaz reservoir, the mean annual discharge is greater than the volume of the reservoir (Apetroaei, 2003). This implies that the renewal time of water is less than one year and that water-sediment interaction within the reservoir is low. It also implies that influent waters will create a strong current. There are considerable seasonal variations in discharge, both in volume and in the accompanying dissolved and suspended load. In this mountainous area, there is low discharge in winter (snow precipitation) and high discharge in early spring (snow melt and spring precipitation). During spring, river waters also carry large loads of particulate matter originating from soil erosion. At the mouth of the river, the inflowing waters may produce an intrusion layer in the reservoir, while the outflow generates a current of water whose velocity depends on the rate of withdrawal. Simulation of reservoir hydrodynamics is based on a large data set of meteorological inflow and withdrawal data and requires complex mathematical models (Boegman et al., 2001; Cole and Buchak, 1995; Herczeg and Imboden, 1988; Imberger and Patterson, 1981; Samolyubov et al., 2000). To simulate stratification and transport, these models are usually coupled to temperature (e.g. Bonnet et al., 2000; Rueda et al., 2006), isotopic composition (e.g. Bergonzini et al., 2001; Brigault et al., 1998) or to tracer numerical simulation (Hocking and Patterson, 1994; Rueda et al., 2006). In the water column, the distribution of an element depends on its origin and then on mixing of waters. Furthermore, according to element characteristics, its speciation depends on chemical conditions prevailing in the water. By studying temperature, major element and selected trace element concentrations, the aim of this paper was to assess location and extent of layers, and the thermal stratification characteristics.
2 Geological and hydrological characteristics of the reservoir
The geology of the drainage basin of the Bicaz reservoir is complex. The Bistriţa River crosses three major geological units: a crystalline zone (schists, micaschists, gneisses, amphibolites), a flysch zone (conglomerates, sandstones, clays, marls, limestones), and a magmatic rock zone (pyroclastites, dacites, andesites, microgabbros, diorites, microdiorites). The reservoir basin itself lies within the flysch zone.
The Bicaz reservoir is long (31.1 km) and narrow (2 km) (Fig. 1). Its main tributaries are the Bistriţa (catchment area 2985 km2) and the Bistricioara (770 km2). Other influents can be ignored. The outflow is located about 45 m below the maximum water level of the reservoir, between the Potoci bay and the dam (Fig. 1). It is made of 4 sluices, disposed horizontally, with a 2.5 m diameter. The depth of the outflow varied from 30 to 50 m (centre of the outflow) depending on the water level during the survey. The Bicaz is a dimictic reservoir (Apopei, 1983). Sampling dates were chosen according to season: at the beginning of summer stratification (June–July), at the end of maximum summer stratification (October), and at the spring overturn (April) (Apopei, 1983). In view of the length of the lake, three sampling sites were selected (Fig. 1): (i) an upper site (#1) opposite Hangu River, about 10 km downstream of the confluence of the Bistriţa with the reservoir, (ii) a middle site (#2) opposite Secu River, and (iii) a lower site (#3) at a point between Potoci River, the dam and the outflow.
From 1973 to 2006, the mean water discharge of the Bistriţa and the Bistricioara Rivers was 1.46 km3/year (National Institute of Hydrology and Water Management of Romania, INHGA, 2008); from 1991 to 2006, the mean water outflow from the reservoir was 1.66 km3/year. Years 2005 and 2006 were particularly rainy with a mean inflow of 1.76 and 2.04 km3/year respectively, and a mean outflow of 2.09 and 2.17 km3/year. In 2005 and 2006, the Bistriţa discharge accounted for a mean of 83% of the total river inflow. In July 2005, the Bistricioara discharge was higher than average, accounting for 33% of the total river inflow. The 2005 and 2006 hydrographs (drawn with monthly mean discharge data) of the reservoir showed two high-water periods per year, in spring and summer, and a low-water period in winter (Fig. 2), with maxima in April–May and August in 2005, and in April and June in 2006. In June 2006, the reservoir overflowed.

Hydrographs of the Bicaz reservoir for years 2005 and 2006 (monthly mean discharges). Arrows indicate the sampling surveys.
Hydrogrammes du lac de barrage de Bicaz pour les années 2005 et 2006 (débits moyens mensuels). Les flèches indiquent les campagnes de prélèvement.
3 Sampling and analytical procedures
Samples were collected from a small motor boat in July 2005, October 2005, April 2006 and June 2006. In July 2005, only site #3 could be sampled (from the surface to a depth of 60 m). The water column was sampled using a vertical water sampler (transparent acrylic tube) linked to a graduated nylon rope. The water column was sampled at depths of 0, 5, 10, 15, 20 m, and then every 10 m to approximately 2 m above the bottom of the reservoir (lowest site elevation). At each sampling site, the water depth was measured with a monobeam echosounder (Cuda™ 168, Eagle). We analyzed major and minor elements and some trace elements (Fe, Ba and Rb are presented here) in filtered and bulk water. Samples were filtered on site with 0.45 μm cellulose acetate membrane filters, which had previously been rinsed with the sample. Aliquots for cation (major, minor and trace elements) and nutrient determination were acidified to pH = 2 with Suprapur grade nitric acid (Merck). Before analysis, samples were stored at about 4 °C in polypropylene bottles. For bulk water samples, acid was added in the laboratory prior to analysis.
Concentrations of species in suspended particles were determined by differences between concentrations measured in filtered and unfiltered water samples. The concentration in particulate matter calculated in this way corresponds to elements present in amorphous phases, in carbonates and to elements adsorbed on stable solids such as clays. Silicate minerals and stable sulphides were not included in the analysis.
Electrical conductivity, pH and temperature were measured on site during sampling. Anion concentrations (Cl, SO4, NO3 and F) were measured by ionic chromatography. Trace elements were analyzed using inductively coupled plasma quadrupole mass-spectrometry (ICP-MS, Perkin Elmer/Sciex Elan 6000).
To gain a clearer understanding of the situation, we also studied the Bistriţa and the Bistricioara before their confluence with the reservoir (Table 1).
Température, conductivité électrique, concentrations en sulfate et en Fe dissous, mesurées dans les rivières, avant la confluence, de juillet 2005 à avril 2006.
Temperature (°C) | Electric conductivity (μS/cm) | SO42− (μmol/L) | Fediss (mol/L) | |||||
Bistrita | Bistricioara | Bistrita | Bistricioara | Bistrita | Bistricioara | Bistrita | Bistricioara | |
July 2005 | 14.6 | 12.6 | 188 | 250 | 212 | 186 | 4.77E-07 | 3.11E-08 |
October 2005 | 8.7 | 8.8 | 229 | 338 | 311 | 218 | 1.64E-07 | 6.48E-08 |
April 2006 | 4.1 | 3.7 | 151 | 200 | 149 | 160 | 6.16E-07 | 2.58E-06 |
4 Results and discussion
4.1 July 2005
The summer stratification began (Fig. 3a). A distinct layer at a depth of 10 to 20 m (Fig. 3a) was identified by electrical conductivity but not by temperature. The thermal contrast between an intrusion layer and reservoir waters had disappeared but the difference in conductivity was still apparent until site #3 (near the dam), after a course of 30 km.

Profiles of temperature and electrical conductivity measured at the three sites: a: in July 2005; b: in October 2005; c: in April 2006 at sites #1 and #2; d: in April 2006 at site #3; e: in June 2006. The black line indicates the outflow depth.
Profils de température et de conductivité électrique mesurés aux trois sites : a : en juillet 2005 ; b : en octobre 2005 ; c : en avril 2006 aux sites #1 et #2 ; d : en avril 2006 au site #3 ; e : en juin 2006. La ligne noire indique la profondeur de la vanne de soutirage.
4.2 October 2005
In October 2005, the authigenic load of organic matter was maximal, but the water column was still oxic except at the outflow depth (Fig. 4b). This result confirms the mesotrophic characteristics of the reservoir (Mazareanu et al., 1983). The Bistriţa River inputs large quantities of suspended matter, about 291,000 tons/year (Ichim et al., 1976), including 14,000 tons of organic matter, mainly carried in summer (Apetroaei, 2003). In October, the thermocline was at the level of the outflow (Fig. 3b). The river inflow was not identified by temperature and electric conductivity (Fig. 3b). The epilimnion appeared homogeneous at each site but conductivity increased from site #1 to #3. The hypolimnion comprised waters with increased dissolved load.
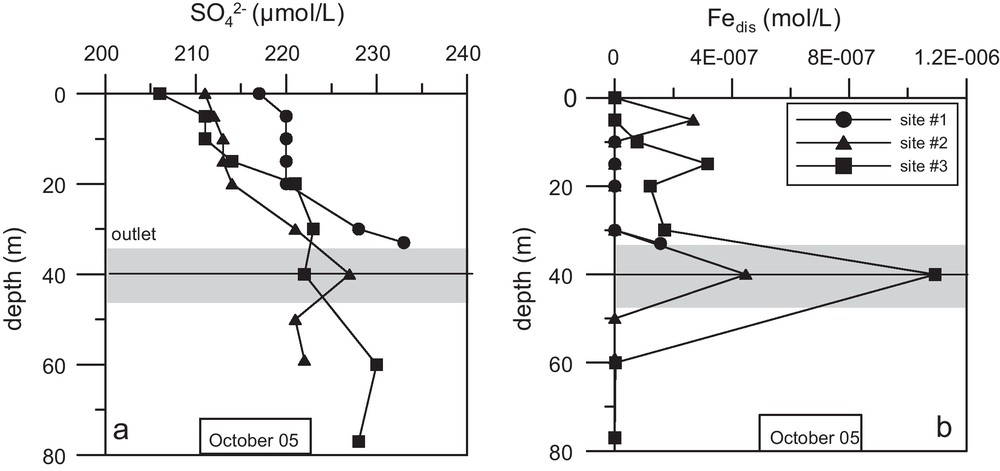
Profiles of: (a) SO4, and (b) Fe in the dissolved phase, at the three sites in October 2005. The black line indicates the outflow depth.
Profils (a) du sulfate et (b) du Fe dissous, aux trios sites en octobre 2005. La ligne noire indique la profondeur de la vanne.
Profiles of sulphate and total dissolved iron (Fig. 4) evidenced a distinct layer at a depth of 30 to 50 m. This layer had a high concentration of sulphate (Fig. 4a), becoming less marked at site #3, while dissolved iron (Fig. 4b) showed a sharp increase from site #1 to #3. This increase in Fediss suggests an anoxic layer in which oxygen consumption is due to the oxidation of detritic organic matter from rivers. At site #3, the concentration of Fediss is about 10-fold that of the Bistriţa River (Table 1). The increase in sulphate concentration measured at site #1 and #2 corroborates the Bistriţa input (Table 1). The decrease observed at site #3 may indicate some sulphate reduction.
During this period, the main chemical composition of the rivers (electrical conductivity, concentration in major constituents) was different from that of the reservoir (Table 1), but the low river discharge (Fig. 2) prevented the use of these parameters to identify mixing. Due to the characteristics of the Bistriţa watershed and the river large load of organic matter, an intrusion layer can be identified from sulphate contents and redox sensitive elements. Our results show that river waters dropped to the outflow depth, creating an anoxic layer between the epi- and the hypolimnion. Some of the influent waters were mixed in the upper part of the water column, explaining the sulphate concentration gradient recorded from site #1 to #3 at a depth of between 5 and 20 m (Fig. 4a), and the dissolution and settling of particulate Fe from site #1 to #3 (Fig. 4b). This mixing was also recorded by the electric conductivity gradient from site #1 to site #3 in the epilimnion. The high density of river waters was caused by its temperature (Table 1).
4.3 April 2006
From February to April, the surface of the reservoir was partially covered with ice (personal observation). The presence of ice, a low-density layer, prevents exchange of thermal energy between the water and the atmosphere. This insulating layer induces a winter thermal stratification with temperatures between 0 and 4 °C from the top to the bottom of the water column.
In April, the river inflow formed a diluted and a “warm” surface layer (Fig. 3c) whose chemical signature deepened from site #1 (10 m depth) to site #2 (15 m depth). After site #2, no signature of river waters was recorded (Fig. 3d). No particular layer was revealed at the outflow depth by temperature, conductivity (Figs. 3c and d) or concentration of major constituents. Trace elements including Ba or Rb indicated input and mixing of river waters with reservoir waters at site #3 (Fig. 5a and b). This mixing was weakly observed by conductivity (Fig. 3d). Barium and rubidium were carried by rivers in both particulate and dissolved forms (Fig. 5). During this period, it seems that the outflow depth acted as a mixing limit between the top of the water column (from 0 to 30 m) and the bottom. Below the outflow depth, the decrease observed in dissolved Ba and dissolved Rb (from a depth of 40 m to the bottom of the reservoir) may have been caused by precipitation of Ba- and Rb-bearing particles or by adsorption of Ba and Rb on settling particles. The total concentration of these two elements was constant below the outflow depth.
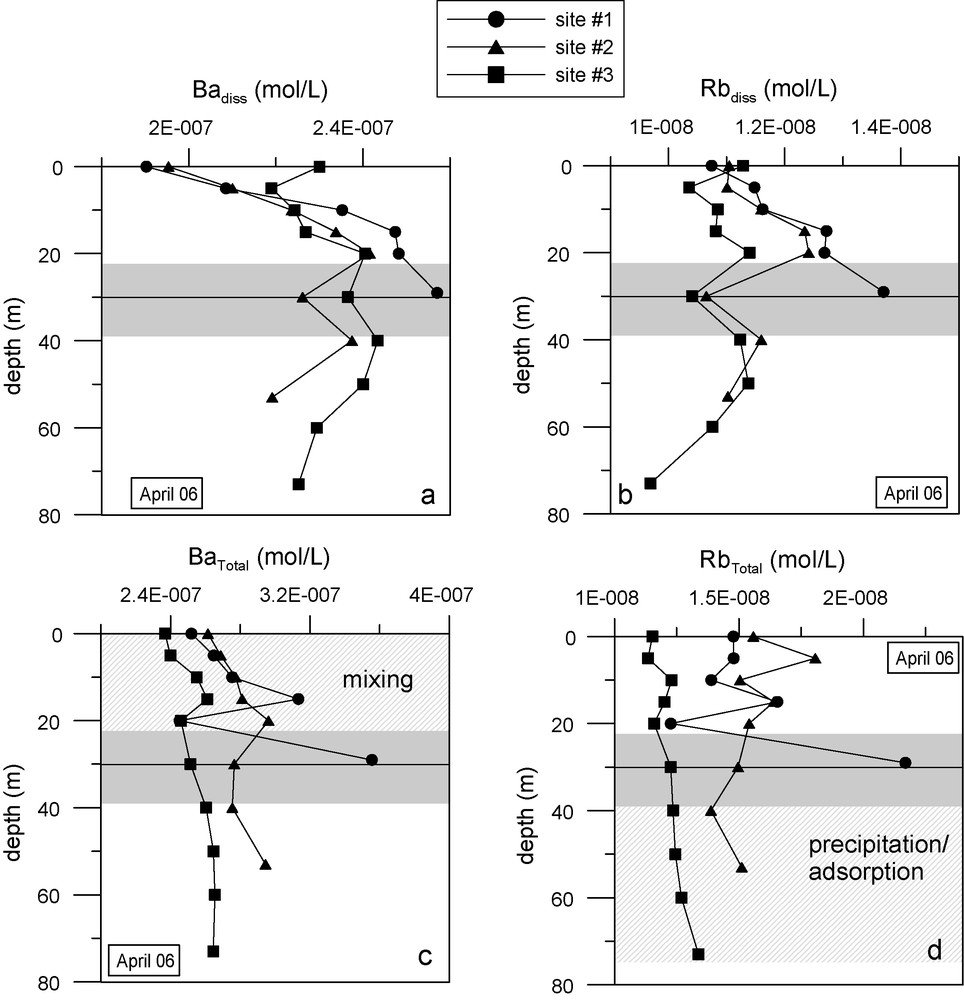
Profiles of: (a) dissolved Ba, (b) dissolved Rb, (c) total Ba, and (d) total Rb, measured at the three sites in April 2006. The black line indicates the outflow depth.
Profils (a) du Ba dissous, (b) Rb dissous, (c) Ba total, (d) Rb total, mesurés aux trois sites en avril 2006. La ligne noire indique la profondeur de la vanne.
4.4 June 2006
In June, the river inflow created a distinct layer at a depth of 10 to 20 m (Fig. 3e), which was identified by electrical conductivity but not by temperature. The thermal contrast between river and reservoir waters should be less marked, at this period, and has disappeared by site #1. By contrast, the difference in conductivity was still apparent until site #3 (near the dam), after a course of 30 km. In July 2005, at site #3, the same distribution than in June 2006 was observed for temperature and conductivity (Figs. 3a and e). But the intrusion layer displayed more mineralized waters than the reservoir in July 2005, and less mineralized waters in June 2006. The outflow formed a distinct current in June 2006, at a depth of 40 to 60 m (Fig. 3e), identified by temperature and electrical conductivity.
4.5 Location of layers and thermal stratification
Fig. 6 summarizes the location of layers from October 2005 to June 2006.

Diagram of location of specific layers. In October 2005: a rapid drop of river waters forming a deep current at the outflow depth, merged with the thermocline. In April 2006: an intrusion layer becoming less marked at the downstream site and a weak deep current that restricts exchanges between the top and the bottom of the water column. In June 2006: a stable intrusion layer and a deep current.
Croquis de la position des couches particulières. En octobre 2005 : un plongement rapide des eaux de rivière qui forment un courant profond à la hauteur de la vanne, confondu avec la thermocline. En avril 2006 : une couche d’intrusion qui s’estompe avant le site aval et un faible courant profond qui limite les échanges entre le haut et le bas de la colonne d’eau. En juin 2006 : une couche d’intrusion stable jusqu’au site aval et un courant profond.
In October 2005, the river inflow (from the Bistriţa and the Bistricioara) rapidly deepened and supplied the deep current located at the outflow depth. This deep current was evidenced at each season, but displayed different characteristics. It acted as a mixing barrier between top and bottom waters of the reservoir. In April 2006, the river inflow is recorded at site #1 and #2 but not at site #3. In June 2006, the river inflow formed an intrusion layer still recorded at site #3. This implies that the velocity of the river inflow was sufficiently high during this period to prevent the exchange (vertical advection and diffusion) between intrusion layer and reservoir waters. It is conceivable that the persistence of the intrusion layer recorded at the lower site is linked to the deep current, allowing a superposition of horizontally moving layers.
The most contrasted profiles were recorded in June 2006 at the three sites, with four layers. The most homogeneous one was recorded at the lower site in April 2006 where the deep current did not change the concentration of major constituents.
Consequently, the composition of bottom waters was mainly regulated by the input of settling particles, water-sediment interaction and spring and autumn mixing.
5 Conclusion
Data from the 2005 and 2006 surveys demonstrate that the water column of the Bicaz reservoir exhibits winter and summer stratification. The summer stratification may be delayed due to the high river inflow of June–July. A deep current, induced by the withdrawal of water, prevents the diffusion of heat to the bottom of the water column and thus the thermocline (October) is less deep (Fontane et al., 1981).
An intrusion layer occurred, originating from the tributary rivers, together with a deep current produced by withdrawal. The deep current was recordable at every season, whereas the intrusion layer was not recorded in October (Fig. 6) because the river inflow supplied mainly the deep current. In early summer (the reservoir overflowed in June 2006), the persistence of the river inflow layer up to the dam may have originated from different causes generally observed in reservoirs: (i) the shape of the confluence (a large bay due to complete replenishment; Wetzel, 1983), (ii) the large flow of incoming river waters (Han et al., 2000), or (iii) a “lack” of mixing between the lighter current (surface) and the denser current (outflow depth) (Odulo et al., 1997). Water transit time may vary considerably according to depth and season: some waters may transit fast (in the deep current) and some more slowly (below the deep current). This contrasted water movement may have a significant impact on biogeochemical processes (Casamitjana et al., 2003; Rueda et al., 2007).
Because chemical elements display different behaviours, their distribution in the water column registers different processes. For trace elements, as stable isotopes, the variability of their abundance permits identification of significant differences of small quantities. But the chemical reactivity, e.g. affinity to Fe oxide, solubility dependent on redox status, produces complementary information. In the Bicaz reservoir case, the use of temperature alone would not identify the location and extent of layers at every season. In October, temperature data could not detect the outflow current while chemical data exposed small input and mixing with river waters in the epilimnion and the presence of an anoxic layer at the outflow depth. Supplementing the examination of mixing conditions with data on major and trace elements can provide additional insights in the study of reservoirs.
Acknowledgements
The authors would like to thank the reviewers, Dr. W.E. Fleenor, University of California, and Dr. M. Poulin, École des Mines de Paris, for their helpful and constructive comments. Neculai A. Apetroaei (Laboratorul de Acvacultura si Ecologie Acvatica, Facultatea de Biologie, Universitatea “Al. I. Cuza” Iasi, Romania) provided invaluable assistance in sampling and data collection. The GéEAC (Université de Tours, France) partially supported this study.