1 Introduction
The group of elements commonly referred to as rare earth elements (REEs) comprises the lanthanoids from La to Lu. They are commonly referred to as a group, because they tend to behave coherently in natural systems. Therefore, measured REE concentrations normalized to those of a natural reference material (e.g., chondrite or shale) produce a smooth distribution pattern. Individual REEs, however, may show anomalies that can easily be recognized and quantified. Small anomalies of La, Gd and Lu may occur due to minor differences between the stabilities of chemical REE complexes, while larger anomalies of Ce and Eu result from their redox sensitivity and may be used to trace temperature and/or redox conditions. In natural surface waters, negative Ce anomalies have been observed, as Ce is oxidatively (and hence preferentially) scavenged by Fe–Mn (oxyhydr-)oxides (e.g. Bau and Koschinsky, 2009; Bau et al., 2014; De Carlo et al., 1998; Elderfield et al., 1990; Ohta and Kawabe, 2001). This leaves the dissolved load depleted in Ce relative to the neighbouring REEs, while the Fe and Mn (oxyhydr-)oxide particles are relatively enriched.
The first occurrence of large positive Gd anomalies was reported in 1996 for rivers in Germany (Bau and Dulski, 1996). Since then, anomalously high Gd concentrations were reported for numerous rivers and lakes (Barber et al., 2006; Bau et al., 2006; Elbaz-Poulichet et al., 2002; Klaver et al., 2014; Knappe et al., 2005; Kulaksiz and Bau, 2007, 2011a, 2013; Lawrence and Bariel, 2010; Möller et al., 2000, 2002, 2014; Morteani et al., 2006; Nozaki et al., 2000; Rabiet et al., 2009; Tricca et al., 1999; Verplanck, 2013; Verplanck et al., 2005, 2010), estuaries and coastal waters (Elbaz-Poulichet et al., 2002; Kulaksiz and Bau, 2007; Lawrence, 2010; Nozaki et al., 2000), groundwater (Knappe et al., 2005; Möller et al., 2000; Rabiet et al., 2009; Strauch et al., 2008), and in tap water (Bau and Dulski, 1996; Kulaksiz and Bau, 2011b; Tepe et al., 2014) in densely populated regions with a highly developed health care system. The origin of the anomalous Gd enrichment was traced back to the clear water effluent of wastewater treatment plants (WWTP) (Bau and Dulski, 1996). Since the late 1980s, Gd-based contrast agents are used in magnetic resonance imaging (MRI). These organic Gd complexes are water-soluble and highly stable in order to prevent any interaction of toxic Gd3+ with the body, and to guarantee complete removal via the kidneys within 24–48 h after injection. However, due to their water solubility and high complex stability, these compounds cannot be removed by conventional wastewater treatment procedures and are released into the environment with the clear water effluent of WWTPs (e.g., Bau and Dulski, 1996; Möller et al., 2000; Verplanck et al., 2005). Hence, anthropogenic Gd can be used as a tracer to track wastewater effluents in the environment (Kulaksiz and Bau, 2011b; Lawrence and Bariel, 2010) and as an indicator for the presence of other wastewater-derived substances, such as pharmaceuticals, personal care products, etc. (Strauch et al., 2008).
2 Study site
Lake Paranoá (15°48′S, 47°50′W, 1000 m altitude) is an artificial freshwater reservoir, which was built in 1959 along with the City of Brasilia, the capital of Brazil (Starling, 1998). It is used for power generation and recreational purposes and provides humidity to the city during the dry months (Abbt-Braun et al., 2014; Starling, 1998). To date, this freshwater reservoir has not been used for the extraction of drinking water (Grisolia and Starling, 2001). However, strong population growth in Brasilia puts pressure on groundwater resources and generates increasing demand for potable water, particularly during the dry period from May to September (the warm wet season lasts from October to April). Hence, the lake could soon become a valuable drinking water reservoir (Abbt-Braun et al., 2014). If the lake is to be used as a drinking water reservoir, the freshwater extraction plant is planned to be located toward the east, at the outflow of the lake (Abbt-Braun et al., 2014).
The lake covers an area of 38 km2 and yields a water volume of 498 × 106 m3. The water depth averages about 14 m with a maximum of 40 m and the retention time of the water in the lake is approximately 0.82 years (Starling, 1998, 2002). There are four main tributaries to the lake: the rivers Bananal and Torto entering from the north and the rivers Riacho Fundo and Gama entering from the south (Fig. 1). The drainage basin of the lake covers 1015 km2. The outflow of the lake is located toward the east (Fig. 1). There is little agricultural and industrial activity within the drainage basin, causing only minor contamination of the lake by agricultural or industrial pollutants (Grisolia and Starling, 2001). However, extensive construction and the subsequent urbanization have significantly increased the lake's load with anthropogenic metals, such as Pb (Franz et al., 2013; Gioia et al., 2006).
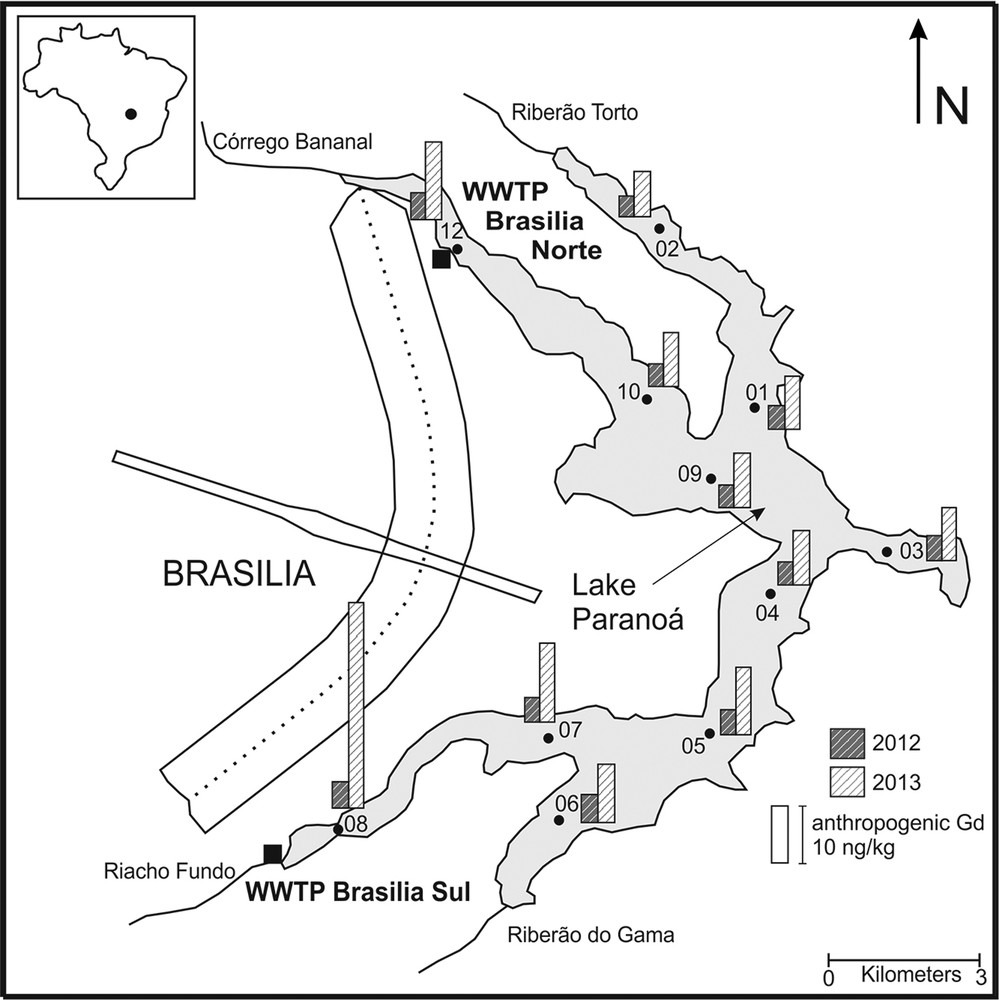
Map of Lake Paranoá, including its tributaries and the sampling locations. Anthropogenic Gd concentrations at each sampling location in 2012 (grey) and 2013 (white) are presented by bars.
In the first decades after the foundation of Brasilia, untreated or inadequately treated sewage was released into the lake, leading to increased nutrient levels and eutrophication (Grisolia and Starling, 2001; Starling, 1998). In 1993, two WWTPs, Brasilia Norte and Brasilia Sul, were built, which remove nutrients, such as N and P, using biological removal processes (Grisolia and Starling, 2001). The WWTP Brasilia Norte, located at in the northern branch of the Bananal tributary, receives domestic sewage from northern Brasilia and processes 479 L/s on average, with a maximum capacity of 911 L/s (CAESB, 2014). The WWTP Brasilia Sul is significantly larger and is located at the confluence of the Riacho Fundo River and the lake (Fig. 1). It services the southern part of the city, where most of the hospitals are located, and processes 1193 L/s on average, with a maximum capacity of 1500 L/s (CAESB, 2014). Since their installation and commissioning, nutrient release into the lake has decreased by 70% (Starling et al., 2002).
Abbt-Braun et al. (2014) have evaluated the raw water quality of Lake Paranoá in order to determine the lake's potential as a drinking water reservoir. They analysed the concentrations of 16 metal(loid)s in unfiltered water samples from Lake Paranoá. The concentrations of all 16 metal(loid)s were all below drinking water limits in Germany and Brazil. In addition, they analysed 50 organic micropollutants of anthropogenic origin (e.g., X-ray contrast media, pharmaceuticals, personal care products, sweeteners, pesticides, and perfluorinated compounds) in water samples taken between 2009 and 2012. In none of these samples, the concentrations of the analysed organic micropollutants exceeded 100 ng/L at the site of future drinking water extraction, except for caffeine and iopromide (a synthetic diagnostic agent). Based on these data, they assigned the lake a good to very good water quality with respect to future drinking water production. However, concentrations of metal(loid)s and organic micropollutants were elevated close to the WWTP Brasilia Sul, identifying the WWTP and the tributary Riacho Fundo as sources of these substances to the lake. As Brasilia's population grows continuously, the use and hence discharge of anthropogenic micropollutants into the lake is likely to increase, which will in the intermediate to long-term affect the quality of the raw water of the reservoir.
3 Materials and methods
For our study, we took water samples at 11 sampling sites in Lake Paranoá in November 2012 (wet season) and July 2013 (dry season) (Fig. 1). The samples were collected in acid-cleaned 1000-mL polyethylene bottles and processed within 24 h.
During sampling in November 2012, the samples were processed unfiltered (i.e. the element concentrations in these samples represent the dissolved + particulate concentrations, i.e. the total concentrations), with the exception of one additional sample taken at site 10, which was 0.2 μm filtered and analysed for its dissolved load (truly dissolved + nanoparticles/colloids < 0.2 μm). In July 2013, two samples were taken at each station, one of which was processed unfiltered for the determination of total concentrations, whereas the other one was filtered through 0.2 μm cellulose acetate membrane filters for the determination of dissolved concentrations. All samples were acidified to a pH between 1.8 and 2.0 with suprapure HCl before a REE pre-concentration procedure was performed (for details on the REE separation/pre-concentration protocol, see Bau and Dulski, 1996; Kulaksiz and Bau, 1996, 2007, 2011a; Shabani et al., 1992). The analysis of REEs and U was performed on a PerkinElmer Elan 6000 inductively coupled mass spectrometer (ICP–MS) at Jacobs University Bremen, Germany. The overall reproducibility of the method is good, the relative standard deviation for two samples taken at the sampling site 10 each year is well below 10% for all REEs (the only exception was La in 2012, for which the relative standard deviation was 15%). Iron and Mn concentrations were determined by inductively coupled plasma optical emission spectroscopy (ICP–OES) with a Spectro Ciros Vision. The lower limit of quantification of Fe and Mn was 0.1 mg/kg.
REEs behave coherently in natural systems. When normalized to a reference material (here, Post-Archean Australian Shale, PAAS, of McLennan, 1989), smooth patterns are produced and any occurring anomalies can be quantified by extrapolation or interpolation using neighbouring elements. In Lake Paranoá, an anomalous behaviour of Ce and Gd can be observed (Fig. 2). Their anomalies are quantified by interpolation between La and Pr (eq. (1)) and by extrapolation via Eu and Nd (eq. (2)), respectively (Kulaksiz and Bau, 2013):
(1) |
(2) |
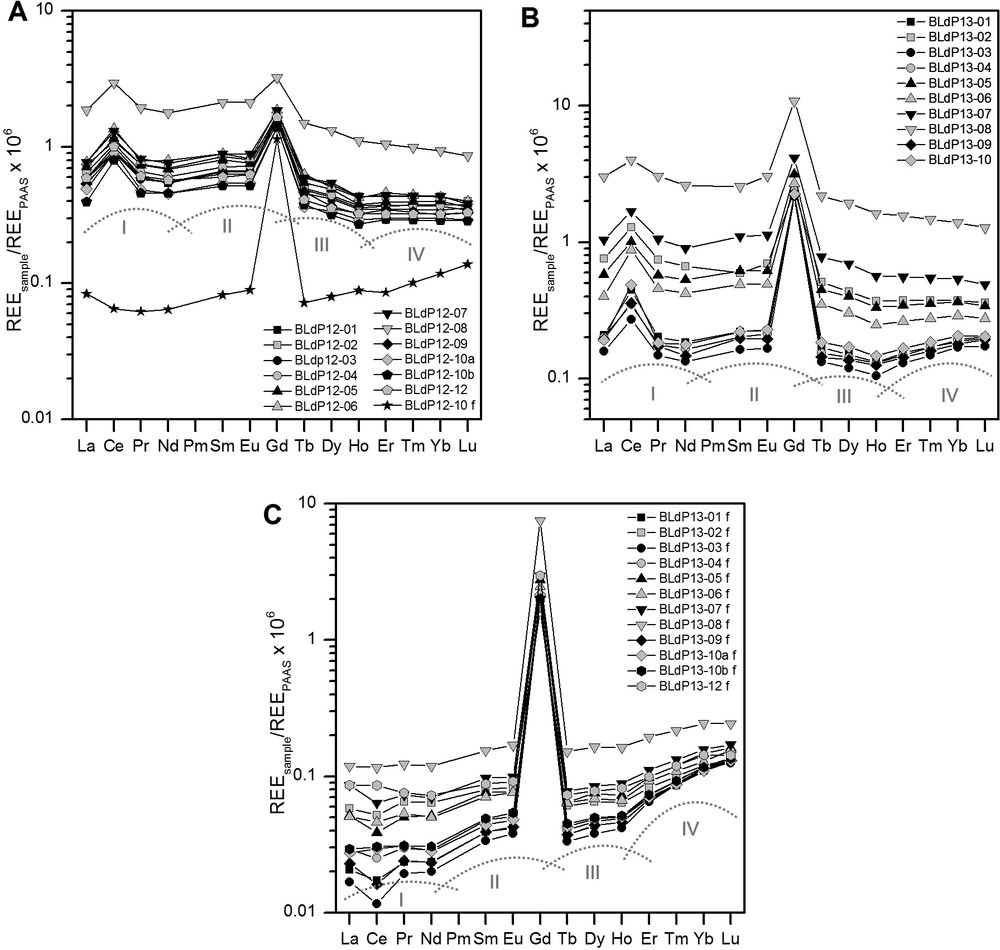
REESN patterns of lake water samples from Lake Paranoá, Brasilia, Brazil. The unfiltered samples and the sole filtered sample taken in 2012 are shown in (A); the unfiltered samples taken in 2013 are shown in (B) and the filtered samples collected in 2013 are shown in (C). All samples show strong positive Gd anomalies and all unfiltered samples show positive Ce anomalies. The four M-shaped tetrads are indicated in grey.
Lanthanum can be used in the calculation of Ce*SN because of the absence of any geogenic or anthropogenic La anomaly in the lake waters (Kulaksiz and Bau, 2011a). The subscript SN denotes normalization to PAAS and the superscript * denotes the natural background value excluding any anomalies. By multiplying the Gd*SN value with the Gd concentrations in PAAS (eq. (3)), the geogenic Gd concentrations can be determined. Hence, the difference between the total Gd load and the geogenic Gd concentration represents the amount of anthropogenic Gd present in a sample (eq. (4)).
(3) |
(4) |
As Tm had been added to the samples as an internal standard to monitor recoveries during the pre-concentration procedure, the geogenic Tm concentrations (needed for the determination of ΣREE) were interpolated as:
(5) |
4 Results
In November 2012, pH values ranged between 7.34 and 7.60, while in July 2013 they were slightly lower and ranged from 7.04 to 7.47 (Table 1). Conductivity was similar during both sampling campaigns, ranging from 95.2 to 108.1 μS/cm in 2012 and from 83.4 to 120.4 μS/cm in 2013 (Table 1). During both years, the highest conductivities were found at the sampling point closest to the discharge site of clear water effluent from WWTP Brasilia Sul (site 8).
Concentrations of REEs, U, Fe and Mn in filtered (f) and unfiltered samples taken from Lake Paranoá in 2012 and 2013. All concentrations are given in ng/kg unless indicated otherwise.
ng/kg | La | Ce | Pr | Nd | Sm | Eu | Gd | Tb | Dy | Ho | Er | Tm* | Yb | Lu | ΣREE | U | Fe (mg/kg) |
Mn (mg/kg) |
pH | Conductivity (μS/cm) |
Gd/Gd* | Ce/Ce* | |
November 2012 | BLdP12-01 | 22.73 | 74.94 | 5.35 | 18.27 | 3.67 | 0.7 | 7.31 | 0.35 | 1.94 | 0.35 | 0.99 | 0.14 | 0.97 | 0.15 | 137.88 | 3.96 | < 0.1 | < 0.1 | 7.43 | 99.9 | 2.26 | 1.57 |
BLdP12-02 | 27.9 | 87.97 | 6.58 | 23.69 | 4.74 | 0.87 | 7.58 | 0.46 | 2.47 | 0.43 | 1.23 | 0.17 | 1.22 | 0.17 | 165.48 | 3.64 | < 0.1 | < 0.1 | 7.36 | 95.2 | 1.92 | 1.5 | |
BLdP12-03 | 22.21 | 72.09 | 5.11 | 18.72 | 3.68 | 0.72 | 7.63 | 0.37 | 2.01 | 0.35 | 1.05 | 0.15 | 1.06 | 0.16 | 135.32 | 4.21 | < 0.1 | < 0.1 | 7.34 | 101 | 2.3 | 1.56 | |
BLdP12-04 | 24.81 | 84.49 | 5.82 | 20.63 | 3.96 | 0.78 | 7.58 | 0.39 | 2.05 | 0.37 | 1.07 | 0.15 | 1.04 | 0.15 | 153.29 | 9.43 | < 0.1 | < 0.1 | 7.4 | 102 | 2.12 | 1.62 | |
BLdP12-05 | 27.3 | 91.96 | 6.52 | 23.31 | 4.45 | 0.82 | 8.03 | 0.42 | 2.29 | 0.38 | 1.12 | 0.16 | 1.13 | 0.16 | 168.04 | 7.56 | < 0.1 | < 0.1 | 7.54 | 102.7 | 2.2 | 1.59 | |
BLdP12-06 | 29.67 | 108.2 | 6.96 | 27.08 | 4.93 | 0.89 | 8.71 | 0.49 | 2.38 | 0.42 | 1.31 | 0.18 | 1.2 | 0.17 | 192.6 | 5.48 | < 0.1 | < 0.1 | 7.42 | 101.7 | 2.25 | 1.74 | |
BLdP12-07 | 29.47 | 103.72 | 7.21 | 25.89 | 4.93 | 0.96 | 8.6 | 0.46 | 2.55 | 0.43 | 1.26 | 0.18 | 1.23 | 0.17 | 187.04 | 6.41 | < 0.1 | < 0.1 | 7.6 | 102.5 | 1.98 | 1.64 | |
BLdP12-08 | 71.16 | 236.34 | 17.15 | 60.35 | 11.75 | 2.29 | 15.08 | 1.16 | 6.19 | 1.1 | 2.99 | 0.4 | 2.65 | 0.37 | 428.98 | 8.42 | < 0.1 | < 0.1 | 7.43 | 108.1 | 1.44 | 1.56 | |
BLdP12-09 | 20.45 | 72.69 | 5.24 | 18.74 | 3.52 | 0.68 | 6.97 | 0.36 | 1.73 | 0.32 | 0.99 | 0.13 | 0.9 | 0.14 | 132.86 | 5.86 | < 0.1 | < 0.1 | 7.36 | 101.3 | 2.28 | 1.62 | |
BLdP12-10a | 18.8 | 72.2 | 4.28 | 15.45 | 3 | 0.59 | 6.54 | 0.28 | 1.59 | 0.29 | 0.85 | 0.12 | 0.85 | 0.13 | 124.96 | 5.48 | < 0.1 | < 0.1 | 7.42 | 99.2 | 2.42 | 1.86 | |
BLdP12-10b | 15.09 | 63.63 | 4.04 | 15.54 | 2.87 | 0.56 | 6.49 | 0.29 | 1.48 | 0.27 | 0.84 | 0.12 | 0.81 | 0.12 | 112.15 | 5.35 | < 0.1 | < 0.1 | 7.42 | 99.2 | 2.57 | 1.88 | |
BLdP12-12 | 22.56 | 79.97 | 5.4 | 19.4 | 3.34 | 0.68 | 7.69 | 0.32 | 1.66 | 0.32 | 0.92 | 0.13 | 0.91 | 0.14 | 143.43 | 4.9 | < 0.1 | < 0.1 | 7.4 | 99.2 | 2.55 | 1.67 | |
BLdP12-10f | 3.19 | 5.18 | 0.55 | 2.17 | 0.45 | 0.1 | 5.33 | 0.06 | 0.37 | 0.09 | 0.24 | 0.04 | 0.33 | 0.06 | 18.15 | n/a | < 0.1 | < 0.1 | 7.42 | 99.2 | 11.47 | 0.9 | |
July 2013 | BLdP13-01 | 7.94 | 35.51 | 1.78 | 6.27 | 1.22 | 0.24 | 10.34 | 0.13 | 0.7 | 0.13 | 0.44 | 0.07 | 0.53 | 0.09 | 65.39 | 2.55 | < 0.1 | < 0.1 | 7.35 | 85.4 | 9.24 | 2.18 |
BLdP13-02 | 28.87 | 102.47 | 6.58 | 22.59 | 3.29 | 0.75 | 11.16 | 0.39 | 2.03 | 0.37 | 1.06 | 0.15 | 1.05 | 0.16 | 180.93 | 3.82 | < 0.1 | < 0.1 | 7.45 | 83.4 | 3.38 | 1.71 | |
BLdP13-03 | 6.03 | 21.67 | 1.31 | 4.52 | 0.9 | 0.18 | 10.05 | 0.1 | 0.56 | 0.1 | 0.37 | 0.06 | 0.48 | 0.07 | 46.41 | 2.35 | < 0.1 | < 0.1 | 7.41 | 89.96 | 12.04 | 1.78 | |
BLdP13-04 | 7.63 | 27.92 | 1.57 | 5.56 | 1.12 | 0.23 | 10.53 | 0.12 | 0.67 | 0.13 | 0.42 | 0.07 | 0.54 | 0.09 | 56.59 | 2.24 | < 0.1 | < 0.1 | 7.4 | 90.97 | 9.83 | 1.86 | |
BLdP13-05 | 22.16 | 80.36 | 5.06 | 17.96 | 3.43 | 0.67 | 14.72 | 0.35 | 1.88 | 0.33 | 0.98 | 0.14 | 1.03 | 0.15 | 149.23 | 3.61 | < 0.1 | < 0.1 | 7.42 | 93.84 | 4.88 | 1.75 | |
BLdP13-06 | 15.39 | 70.12 | 4.02 | 14.29 | 2.71 | 0.53 | 12.56 | 0.27 | 1.41 | 0.25 | 0.75 | 0.11 | 0.81 | 0.12 | 123.35 | 3.28 | < 0.1 | < 0.1 | 7.43 | 92.1 | 5.2 | 2.06 | |
BLdP13-07 | 39.81 | 133.93 | 9.32 | 30.66 | 6.11 | 1.22 | 19.42 | 0.61 | 3.24 | 0.56 | 1.59 | 0.22 | 1.51 | 0.21 | 248.41 | 4.72 | < 0.1 | < 0.1 | 7.37 | 96.2 | 3.43 | 1.6 | |
BLdP13-08 | 115.32 | 318.77 | 26.93 | 88.42 | 14.26 | 3.3 | 50.62 | 1.69 | 9.02 | 1.61 | 4.46 | 0.6 | 3.93 | 0.55 | 639.48 | 9.35 | < 0.1 | < 0.1 | 7.04 | 120.4 | 3.38 | 1.32 | |
BLdP13-09 | 7.42 | 28.38 | 1.52 | 5 | 1.09 | 0.21 | 10.41 | 0.11 | 0.64 | 0.12 | 0.41 | 0.06 | 0.51 | 0.08 | 55.97 | 2.38 | < 0.1 | < 0.1 | 7.35 | 84.7 | 10.44 | 1.95 | |
BLdP13-10 | 7.32 | 38.6 | 1.62 | 6.01 | 1.23 | 0.25 | 10.54 | 0.14 | 0.8 | 0.14 | 0.47 | 0.07 | 0.58 | 0.09 | 67.86 | 2.6 | < 0.1 | < 0.1 | 7.47 | 84.8 | 9.1 | 2.59 | |
BLdP13-01f | 0.79 | 1.37 | 0.21 | 0.8 | 0.22 | 0.05 | 9.28 | 0.03 | 0.2 | 0.05 | 0.19 | 0.03 | 0.31 | 0.06 | 13.58 | 1.77 | < 0.1 | < 0.1 | 7.35 | 85.4 | 39.08 | 0.78 | |
BLdP13-02f | 2.21 | 4.13 | 0.58 | 2.17 | 0.44 | 0.09 | 7.87 | 0.05 | 0.3 | 0.06 | 0.24 | 0.04 | 0.34 | 0.06 | 18.59 | 2.85 | < 0.1 | < 0.1 | 7.45 | 83.4 | 18.51 | 0.84 | |
BLdP13-03f | 0.64 | 0.92 | 0.17 | 0.68 | 0.19 | 0.04 | 9.02 | 0.03 | 0.18 | 0.04 | 0.19 | 0.03 | 0.32 | 0.05 | 12.5 | 1.79 | < 0.1 | < 0.1 | 7.41 | 89.96 | 40.87 | 0.64 | |
BLdP13-04f | 1.12 | 2 | 0.26 | 0.97 | 0.27 | 0.05 | 9.01 | 0.03 | 0.22 | 0.05 | 0.21 | 0.04 | 0.33 | 0.06 | 14.62 | 1.79 | < 0.1 | < 0.1 | 7.4 | 90.97 | 31.66 | 0.85 | |
BLdP13-05f | 1.98 | 3.07 | 0.44 | 1.75 | 0.42 | 0.08 | 12.89 | 0.05 | 0.35 | 0.07 | 0.28 | 0.05 | 0.42 | 0.07 | 21.93 | 2.06 | < 0.1 | < 0.1 | 7.42 | 93.84 | 31.64 | 0.76 | |
BLdP13-06f | 1.93 | 3.67 | 0.47 | 1.7 | 0.39 | 0.08 | 11.47 | 0.05 | 0.32 | 0.07 | 0.26 | 0.04 | 0.36 | 0.07 | 20.9 | 1.52 | < 0.1 | < 0.1 | 7.43 | 92.1 | 28.07 | 0.89 | |
BLdP13-07f | 3.29 | 5.07 | 0.64 | 2.36 | 0.54 | 0.11 | 13.83 | 0.06 | 0.39 | 0.09 | 0.32 | 0.05 | 0.44 | 0.07 | 27.28 | 2.37 | < 0.1 | < 0.1 | 7.37 | 96.2 | 26.48 | 0.8 | |
BLdP13-08f | 4.53 | 9.23 | 1.08 | 4.04 | 0.86 | 0.18 | 35.19 | 0.12 | 0.77 | 0.16 | 0.55 | 0.09 | 0.69 | 0.11 | 57.6 | 3.84 | < 0.1 | < 0.1 | 7.04 | 120.4 | 39.61 | 0.96 | |
BLdP13-09f | 0.88 | 1.29 | 0.21 | 0.79 | 0.22 | 0.05 | 9.68 | 0.03 | 0.2 | 0.05 | 0.19 | 0.04 | 0.32 | 0.06 | 14 | 1.24 | < 0.1 | < 0.1 | 7.35 | 84.7 | 39.97 | 0.69 | |
BLdP13-10af | 1.05 | 2.34 | 0.27 | 0.95 | 0.24 | 0.05 | 10 | 0.03 | 0.23 | 0.05 | 0.2 | 0.04 | 0.31 | 0.06 | 15.83 | 1.61 | < 0.1 | < 0.1 | 7.47 | 84.8 | 37.37 | 1.01 | |
BLdP13-10bf | 1.12 | 2.43 | 0.27 | 1.04 | 0.27 | 0.06 | 9.39 | 0.03 | 0.23 | 0.05 | 0.21 | 0.04 | 0.33 | 0.06 | 15.53 | 1.97 | < 0.1 | < 0.1 | 7.47 | 84.8 | 30.86 | 1.01 | |
BLdP13-12f | 3.29 | 6.82 | 0.67 | 2.46 | 0.49 | 0.1 | 13.9 | 0.06 | 0.37 | 0.08 | 0.28 | 0.05 | 0.4 | 0.06 | 29.03 | 1.87 | < 0.1 | < 0.1 | 7.17 | 88.5 | 30.04 | 1.06 |
We emphasize that in all samples, Fe and Mn concentrations are below the determination limit of 0.1 mg/kg. U concentrations range from 2.2 to 9.4 ng/kg in the unfiltered samples and from 1.2 to 3.8 ng/kg in the filtered samples (Table 1). There is no significant difference between the U concentrations measured in 2012 and those measured in 2013. The U concentrations in Lake Paranoá are well below the 1500 ng/kg limit of U concentrations proposed for drinking water by WHO (2011). Dissolved organic carbon (DOC) ranges from 1.69 to 2.68 mg/kg, which confirms earlier results reported by Abbt-Braun et al. (2014).
Dissolved ΣREE concentrations are 18.2 ng/kg in the single filtered sample from 2012 and range from 12.5 to 57.6 ng/kg in the 2013 samples (Table 1). Total REE concentrations as determined from the unfiltered samples range from 112.2 to 429.0 ng/kg in 2012 and 43.9 to 639.5 ng/kg in 2013. The highest total and dissolved ΣREE concentrations are found in the samples taken closest to the discharge site of WWTP Brasilia Sul (site 8).
The REESN patterns are shown in Fig. 2. The unfiltered samples from both sampling campaigns show very similar patterns (Fig. 2A and B) with enrichment of the light REEs relative to the heavy ones (LREEs and HREEs, respectively; 2012: LaSN/YbSN = 1.37–1.98; 2013: LaSN/YbSN = 0.93–2.16). Both sample sets display the M-type lanthanide tetrad effect (e.g., Bau, 1996, 1999; Davranche et al., 2004; Masuda et al., 1987), but it is most pronounced for samples with low ΣREE concentrations. All REESN patterns of unfiltered samples show strong positive Ce anomalies and large positive Gd anomalies (Fig. 2A and B). The size of the Ce anomalies shows only minor change from 2012 to 2013, ranging from 1.50 to 1.88 in 2012 and from 1.32 to 2.59 in 2013. Although Ce concentrations are always highest closest to the WWTP Brasilia Sul, the size of the Ce anomaly does not vary systematically throughout the lake. In contrast, the size of the positive Gd anomaly increased from 2012 (GdSN/GdSN*: 1.4 and 2.6) to 2013 (GdSN/GdSN*: 3.4 to 12.0) (Table 1). The same trend can be observed for the Gd concentrations throughout the lake and for the relative amount of anthropogenic Gd present in the water column (Fig. 1). In 2012, total Gd concentrations ranged between 6.49 and 15.08 ng/kg, 30–61% of which were of anthropogenic origin. Concentrations increased to 10.05–50.63 ng/kg in 2013, and the percentage of anthropogenic Gd present in the water column rose to 70–92%. In both years, by far the highest Gd concentrations occur closest to the WWTP Brasilia Sul.
The REESN patterns of the dissolved load display a generally increasing trend from LREEs to HREEs (LaSN/YbSN: 0.15–0.61) with four distinct convex segments indicative of the M-type lanthanide tetrad effect (Fig. 2A and C) (e.g., Bau, 1996, 1999; Davranche et al., 2004; Masuda et al., 1987). While some samples show a small negative Ce anomaly (CeSN/CeSN*: 0.64–1.06), no positive Ce anomalies were observed. All samples show large positive Gd anomalies. At site 10, the Gd anomaly increased from 11.5 in 2012 to 37.4 in 2013 and the dissolved Gd concentration increased from 5.33 ng/kg (91% anthropogenic) to 10.00 ng/kg (97% anthropogenic). The particulate REE pool in a freshwater sample is defined to comprise all the particles that do not pass a 0.45 μm or 0.2 μm filter membrane (Elderfield et al., 1990). The concentrations of REEs in this particulate pool were calculated by subtracting the concentrations of REEs in the dissolved pool (i.e. in the 0.2 μm filtrates) from the total REE concentration (i.e. that of the unfiltered samples). The fraction of REEs bound to particulates decreases with increasing REE atomic number. While on average, 89% of total La is bound to particulates, only 47% of total Lu is present in the particulate pool. One exception to this smooth decreasing trend is Ce, on average 95% of which occurs in the particulate phase. The other exception is Gd, on average only 16% of which are present in the particulate fraction. The highest REE concentrations in the particulate phase were also found at sampling site 8, closest to the WWTP Brasilia Sul.
5 Discussion
The highest ΣREE concentration and the highest concentrations of individual REEs are observed closest to the WWTP Brasilia Sul, indicating that either the tributary Riacho Fundo or the WWTP effluent is the major source of all REEs to the lake water. As there is no significant difference in ΣREE concentrations between the wet (2012) and dry season (2013), it is unlikely that the Riacho Fundo tributary carries high loads of REEs into the lake. Although ΣREE concentrations are highest for both, the filtered and unfiltered sample set at the sampling site closest to WWTP Brasilia Sul, the general REESN patterns of the dissolved as well as the total REEs are rather similar throughout the lake, indicating that while the WWTP effluent is the major source of REEs, mixing processes transport wastewater-derived REEs also to those parts of the lake that do not directly receive any WWTP effluent.
While the REESN patterns of the dissolved load show a depletion of LREEs relative to the HREEs (LaSN/YbSN = 0.15–0.61), this trend is reversed for the REESN patterns of the total load (LaSN/YbSN = 0.93–2.16) (Fig. 2). The difference between the two pools represents the particulate load. Hence, the particulate load must be enriched in LREEs relative to HREEs when normalized to shale (LaSN/YbSN = 1.83–2.77) (Fig. 3). This is corroborated by the amount of REEs present in the particulate fraction. While on average 95% of all Ce is bound to the particulate load, this fraction continuously decreases with increasing the atomic number to an average of 47% for Lu.

REESN patterns of the particulate load of Lake Paranoá. The REE concentrations of the particulate load were determined by subtracting the REE concentrations of the filtered samples from those of the unfiltered samples. All samples show positive Ce anomalies, but no Gd anomalies.
The trends in the REESN patterns of the dissolved and particulate loads result from an interplay of sorption processes at particle surfaces and chemical complexation in solution. The dissolved REE concentrations in river and lake water are dominated by REE complexation with inorganic ligands (F−, Cl−, SO42−, OH−, CO32−, PO43−) (Johannesson et al., 1996; Turner et al., 1981) and organic ligands (e.g. fulvic and humic acids; e.g. Sholkovitz, 1978; Tang and Johannesson, 2003). For the REEs, the gradual decrease in atomic radius with increasing atomic number (“lanthanide contraction”) often causes a tendency of stability constants of complexes with dissolved ligands to increase with increasing atomic numbers (Cantrell and Byrne, 1987; Lee and Byrne, 1993). Sholkovitz (1995) showed that in natural systems free REE3+ ions are readily attached via surface complexation to particle surfaces, most importantly Fe–Mn (oxyhydr-)oxides and organic colloids and particles. Sorption processes, therefore, strongly affect REE concentrations in natural aqueous systems. As the net effect of solution and surface complexation, HREEs partition preferentially into solution, whereas LREEs are preferentially scavenged by particles (Elderfield et al., 1990). Hence, the particulate REE pool is relatively enriched in LREEs and the dissolved REE pool is relatively enriched in HREEs, when compared to the total REE load.
The REESN patterns of the dissolved and total REE pool display four convex segments, i.e. the M-type lanthanide tetrad effect (Fig. 2). Most of the fractionation of isovalent REEs in natural systems can be explained by lanthanide contraction. However, the REEs with a quarter-filled, half-filled or full 4f orbital have been shown to behave slightly anomalous, as their electron configuration causes the stabilities of their chemical complexes to deviate from the general trend defined by their REE neighbours (Bau, 1996, 1997; Kawabe, 1992). The lanthanide tetrad effect has been found in seawater, and in other natural waters that had interacted with particles, such as Fe oxyhydroxides, although in these studies the W-type lanthanide tetrad effect had been observed in the solution and the M-type tetrad effect in the precipitate (Bau, 1998, 1999; Davranche et al., 2004; Kawabe et al., 1998, 1999; Masuda and Ikeuchi, 1979; Masuda et al., 1987). As both the dissolved and total REE load of Lake Paranoá are characterized by the same M-type tetrad effect, Fe (oxyhydr-)oxide colloids and nanoparticles that are important carriers of REEs in freshwater, with a REE pattern with M-type tetrad effect, could be the cause of the observed REE distribution. The M-type tetrad effect is also observed in the unfiltered samples, albeit not as pronounced as in the filtered ones. This suggests that the particulate REE pool is different from the colloidal/nanoparticulate pool. While the particulate load is more similar to average upper continental crust (although more enriched in LREEs), the colloidal load appears to be the carrier of the M-type lanthanide tetrad effect. This is supported by the fact that the tetrad effect is most pronounced in the samples with the lowest ΣREE concentrations, in which the signal from the nanoparticle/colloid-bound REEs is not obliterated by the high REE concentration of the particulate pool. The M-type lanthanide tetrad effect is visible in samples from both 2012 and 2013, indicating that whatever caused this effect did not change within the sampling interval.
Most of the filtered samples (i.e. the dissolved REE pool) show a small negative Ce anomaly, which is common for pristine rivers and lakes, because a significant fraction of Ce gets oxidatively bound to particles and is thereby removed from the dissolved REE pool. In contrast to the filtered water, the REESN patterns of the unfiltered waters samples (i.e. the total REE pool) show a pronounced positive Ce anomaly, indicating oxidative scavenging of Ce onto particles in the lake's water. These particles may be inorganic Mn or Fe (oxyhydr-)oxides or organic particles, which all are known to oxidatively scavenge Ce (Bau, 1999; Bau et al., 1997; De Carlo et al., 1998; Freslon et al., 2014; Pourret et al., 2008; Steinmann and Stille, 2008; Stille et al., 2006). The positive Ce anomaly, quantified by Ce/Ce*, ranges from 1.32 to 2.59 and does not change significantly between 2012 and 2013, indicating that the supply of excess Ce to the lake remained constant throughout the sampling period. However, it is still unclear where the excess Ce comes from. The differences in size and direction of the Ce anomalies in the dissolved and total REE pools render it unlikely that this Ce decoupling occurred within the lake, as preferential scavenging of Ce should have resulted in much larger negative Ce anomalies in the respective dissolved pool than are actually observed. This rather suggests that these particles had already developed a positive Ce anomaly before they entered the lake. Although the total Ce concentration is highest closest to WWTP Brasilia Sul, the size of the Ce anomaly shows only little variation throughout the lake. This could indicate that the excess Ce enters the lake with the clear water effluent of the WWTP Brasilia Sul. However, there have been no reports demonstrating that processes within a WWTP could facilitate the release of excess Ce to the environment. There are some fertilizers used in Brazil that carry high loads of Ce, which could potentially be washed out and carried into the lake by the tributaries (Turra et al., 2011). However, REESN patterns of fertilizers commonly applied in Brazil do not show any anomalous behaviour of Ce (Smidt et al., 2011). Even if the applied fertilizers carry excess amounts of Ce, they are unlikely to be the sole supplier of the excess Ce in Lake Paranoá, because there is only little agricultural activity within the drainage basin of the lake, implying that the amounts of fertilizers utilized would not be enough to cause the observed Ce anomalies (Grisolia and Starling, 2001). Hissler et al. (2015) also observed positive Ce anomalies in the particulate fraction, but no Ce anomaly in the dissolved load, in a small creek discharging into the Alzette River in Luxembourg. They attributed this positive Ce anomaly to anthropogenic Ce input from local industry. As industrial activity is low in the catchment of Lake Paranoá, it is unlikely that the industrial use of Ce causes the observed positive Ce anomalies in Lake Paranoá. Anthropogenic Ce nanoparticles as potential contaminants can be excluded because they would produce a positive Ce anomaly in the dissolved REE pool as well. Kronberg et al. (1979) analysed sixteen soil samples collected close to Brasilia, thirteen of which show a significant positive Ce anomaly when normalized to shale (Ce/Ce* = 1.56–4.41). Erosion and aeolian (in the dry season) and/or fluvial (in the wet season) transport of such lateritic dust into the lake could cause the positive Ce anomaly. The fact that a strong Ce anomaly can only be observed in the particulate REE pool further supports the hypothesis that the REE signatures of the particulate and colloidal pools are distinctly different.
The REE budget of Lake Paranoá is influenced by the input of anthropogenic Gd that enters the lake with the clear water effluents from both WWTPs. The highest Gd concentrations in both years in the unfiltered as well as in the filtered samples are found close to the WWTP Brasilia Sul, the larger of the two WWTPs in Brasilia and the one that processes the sewage from the majority of Brasilia's hospitals. This suggests that most of the anthropogenic Gd enters the lake with the clear water effluent of WWTP Brasilia Sul. However, elevated Gd concentrations are also found close to the WWTP Brasilia Norte, although these are not as high as those at Brasilia Sul. The spatial distribution confirms that the anthropogenic Gd enters the lake with the discharge of the WWTPs. This further confirms findings by Abbt-Braun et al. (2014), who show that WWTP Brasilia Sul is the major source of anthropogenic micropollutants to the lake. The calculated concentrations of anthropogenic Gd present in both the dissolved and particulate load are in close agreement (relative standard deviation below 15%), indicating that the anthropogenic Gd is water-soluble and not bound to particles, corroborating the interpretation that the anthropogenic Gd is derived from the discharge of the highly soluble and very stable anthropogenic Gd complexes used as contrast agents in MRI scans (e.g. Bau and Dulski, 1996; Möller et al., 2000; Verplanck et al., 2005). While Gd concentrations are highest close to the WWTPs, pronounced Gd anomalies can be found throughout the lake. In spite of the short retention time of the lake water of only 299 days, the rather small variation of the size of the Gd anomalies, quantified by Gd/Gd*, indicates that the water is well mixed.
While the dissolved and total concentrations of the geogenic REEs are similar in both years, the amount of anthropogenic Gd increased substantially. The anthropogenic Gd anomaly in the unfiltered samples increased from 1.9–2.6 in 2012 to 3.4–12.0 in 2013, representing an increase of up to 460% within just eight months. When looking at the dissolved pool, the anthropogenic Gd anomaly increased from 11.5 in 2012 to 37.4 in 2013 (site 10), representing an increase of 330%.
As the anthropogenic Gd originates from applications during MRI scans, this increasing trend is in agreement with the increasing numbers of MRI applications in the world. The MRI scans performed in the federal district of Brasilia increased from 4218 in 2012 to 5163 in 2013 and are likely to increase even further (Secretaria de Estado de Saudé do Distrito Federal, 2015). Hence, the use of Gd-bearing contrast agents has increased and, therefore, the amount of Gd contrast agents discharged into the lake. During an MRI scan, 0.1 mmol Gd is injected per kg of body weight (Carr et al., 1984). This amounts to 1.0–1.3 mg of Gd injected during one scan, assuming a body weight between 60 and 80 kg for an average Brazilian individual. Multiplying this by the number of MRI scans performed in the federal district of Brasilia shows that the amounts of Gd used during MRI scans increased from 4.0–5.3 kg in 2012 to 4.9–6.5 kg in 2013. This closely matches the 6.1 kg of anthropogenic Gd present in the lake in 2013, which was calculated by multiplying the average anthropogenic Gd concentration with the lake's water volume, further supporting the conclusion that the anthropogenic Gd originates from the application of Gd-bearing contrast agents during MRI scans. As even more MRI scans will be performed in the future, the lake's load with anthropogenic Gd is likely to increase even further, as until now, there are no widely available low-cost methods to remove this anthropogenic Gd during sewage treatment in WWTPs.
Anthropogenic Gd is only one of many anthropogenic micropollutants in the hydrosphere. The lake's load with various anthropogenic micropollutants, including X-ray contrast media, pharmaceuticals, personal care products, pesticides or perfluorinated compounds, was classified to be relatively low by Abbt-Braun et al. (2014), who analysed water samples taken between 2009 and 2012. However, the strong increase in anthropogenic Gd, which can be used as a tracer for wastewater-derived substances, between November 2012 and July 2013, suggests that similar anthropogenic micropollutants, such as the ones analysed by Abbt-Braun et al. (2014) may have also increased significantly and are likely to increase even further, as Brasilia's population is continuously growing.
The ecotoxicological effects of REEs are still poorly understood (Gonzalez et al., 2014). However, the mobility and bioavailability of REEs, which in turn are controlled by factors including pH, temperature and the types of (in-)organic ligands present, have been shown to be controlling parameters when investigating the toxicity of REEs. In addition, the type of organism exposed to elevated REE concentrations is also crucial when determining the level at which toxic effects start to occur (Gonzalez et al., 2014). So far, no studies have been performed to assess the long-term health effects of consumption of drinking water containing anthropogenic Gd. As anthropogenic Gd can be used as a tracer for other wastewater-derived substances, such as pharmaceuticals, personal care products and food additives (e.g., Kulaksiz and Bau, 2011b; Strauch et al., 2008; Tepe et al., 2014), the potential detrimental health effects of such substances should also be taken into consideration before using Lake Paranoá as a drinking water reservoir. However, even at the strongly elevated concentrations observed, the Gd itself is currently not yet a health threat.
6 Conclusions
The REE distribution in the 0.2-μm filtered (dissolved) and in the unfiltered (total REEs) waters from Lake Paranoá, Brasilia, showed that there are distinct differences between the two. As most of the particle-reactive REEs are bound to particles and/or their surfaces, the REE concentrations of the unfiltered water samples are notably higher than those of the filtered ones. While the unfiltered water samples show LREE enrichment, the filtered water samples are enriched in HREEs. Filtered as well as unfiltered samples from 2012 and 2013 display the M-type lanthanide tetrad effect, which is more evident in the filtered than in the unfiltered samples. This suggests that the REE signature of the dissolved load may be controlled by a nanoparticulate/colloidal fraction showing an M-type lanthanide tetrad effect. This effect is less pronounced in the unfiltered samples, suggesting that this nanoparticulate/colloidal signal recognizable in the unfiltered sample is obscured by the particulate REEs. In contrast to the nanoparticulate/colloidal fraction, the REESN pattern of the particulate load is rather flat, but shows a positive Ce anomaly. The size of the Ce anomaly did not vary significantly between 2012 and 2013. While the cause of the positive Ce anomaly is still unclear, erosion and transport of Ce enriched soils/dust into the lake is the most likely source of the excess Ce.
This study is the first one to confirm the presence of anthropogenic Gd, which is used as a contrast agent during MRI, in surface waters in Brazil. The anthropogenic Gd anomaly, typically quantified as Gd/Gd*, increased between November 2012 and July 2013 by up to 460%. As the MRI market in Brazil is rapidly expanding and expected to grow even further, the discharge of anthropogenic Gd into the lake will most likely increase within the near future as well. Because the ecotoxicological effects of REEs are still poorly understood, it is important to perform further studies on the bioavailability and possible health effects of both, the anthropogenic Gd as well as the excess Ce.
Since anthropogenic Gd is a tracer for other wastewater-derived substances, such as pharmaceuticals, personal care products and food additives, monitoring the REE distribution in Lake Paranoá offers a rather inexpensive yet robust way of monitoring the presence of such xenobiotics in the lake water if the lake may be used as a drinking water reservoir in the future.
Acknowledgements
We thank Nathalie Tepe, Jule Mawick and Daniela Meißner for their help in the Geochemistry Laboratory at Jacobs University Bremen, and Barbara Alcantara Ferreira Lima, Jeane Grasyelle and Kamila Morgano for their support in the Laboratório Geochronologia in Brasilia. The research leading to these results has received funding from the European Union Seventh Framework Programme FP7/2007-2013 under grant agreement No. 295091 to MB and ED. The document reflects the view of the authors only. The European Union is not liable for any use that may be made of the information contained therein.