1 Introduction
The geodynamic setting of the Levant Rift system is commonly considered to be a prime example of a large transform fault exposed on land (e.g., Freund, 1970; Garfunkel, 1981), where its post-Eocene age and its transform characteristics are commonly taken for granted (e.g., Gvirtzman and Steinberg, 2012; Hartman et al., 2015). Evidence for left-lateral displacement during the Late Quaternary at a rate of ca. 5 mm/yr (Klinger et al., 2000) is considered representative. However, the tectonic regime of that rift system has been highly debated, and Dubertret (1970), Mart and Horowitz (1981), Picard (1987) and Horowitz (2001) emphasized the extensional structural features of the rift, indicated by its normal boundary faults and large dip-slip throws. The latter interpretation presumed that the rift system was generated primarily by oblique tectonic extension, and that it was initiated in the Late Miocene; it serves as a prime example of early continental break-up (Mart and Dauteuil, 2000; Mart et al., 2005).
The Levant Rift system is a 1100-km-long, north–south-trending series of structural and morphological depressions bounded by structurally uplifted mountainous terrains along its flanks, where large normal faults constrain the mountain-to-valley transitions in many places. The system comprises two segments of distinct tectonic patterns. The southern segment of the rift system extends from the Red Sea through the Gulf of Elat, the Dead Sea and the Sea of Galilee, to the Lebanese Baqa’a (Fig. 1). This rifted segment is built of a series of axial downthrown grabens and uplifted mountainous flanks, with vertical throw of several kilometers, separated from each other by thresholds of subdued structures, where the downthrow of the rifts and the uplift of their flanks are reduced. In addition to vertical displacement, sinistral strike-slip displacement was observed along the southern segment of the system (e.g., Ben-Avraham et al., 2010; Freund et al., 1970; Joffe and Garfunkel, 1987; Mart and Horowitz, 1981). Consequently, the oblique displacement along the Levant Rift system is commonly accepted (e.g., Joffe and Garfunkel, 1987; Mart et al., 2005), even though its quantitative details are debated (e.g., Mart, 2013; Mart and Rabinowitz, 1986; Sobolev et al., 2005).
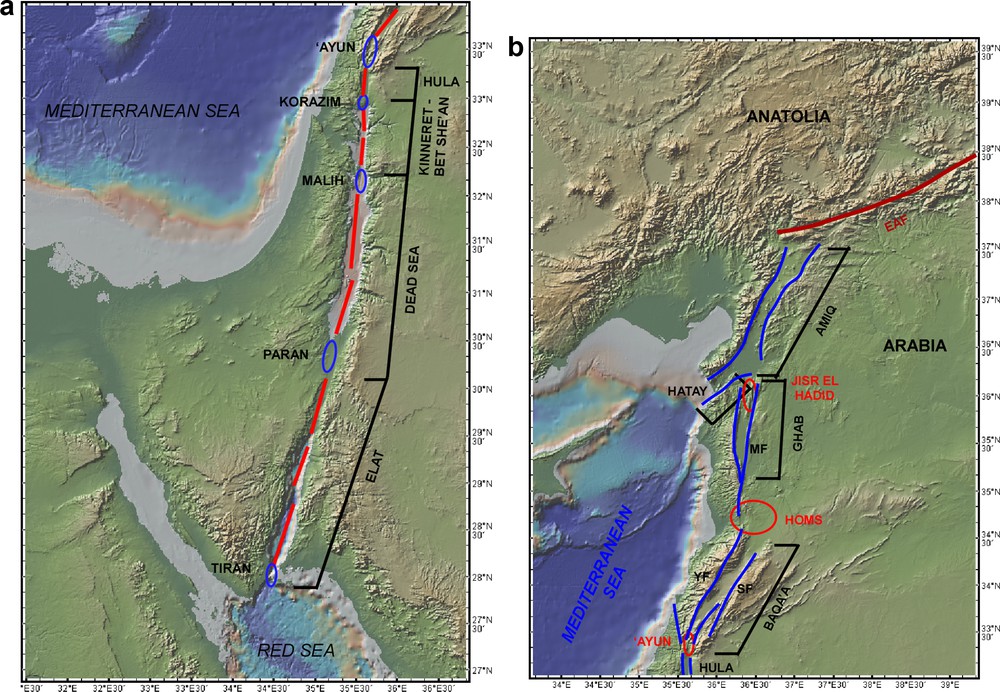
(Color online.) General layout of the Levant Rift System and its two major components, the southern segment with its internal grabens (blue ovals) and thresholds (blue arrows), and the northern Amiq–Ghab–Hatay grabens (red ovals). Tectonically, the southern segment is connected with the Red Sea in the South and the Ghab–Amiq section with the East Anatolian Fault to the northeast. (a) The Elat, Dead Sea and Sea of Galilee graben components of the southern section. (b) The Lebanese Baqa’a (blue oval) and its fault splay (Black line) and the Ghab and Amiq grabens (red ovals). Hatay graben is the SW extension of Amiq graben. Note the linear offset between the Baqa’a and Ghab, and the diversion of the Yammouneh fault from a NNE–SSW trend in the south to a north–south one further northwards.
Charts: courtesy of https://www.geomapapp.org.
The setting of axial grabens and intermittent thresholds is not unique to the Levant Rift: it was depicted also in the East African Rift (e.g., Bosworth, 1985; Ebinger et al., 1987; Gawthorpe and Hurst, 1993), and in the central and northern Red Sea (Bonatti, 1985; Le Pichon and Francheteau, 1978). And like the Red Sea, the northward diminishing size of the axial grabens was used to suggest the geodynamics of the early stages of continental break-up and the initiation of basaltic accretion in divergent plate boundaries as gradual ascent of punctiform magmatic diapirs (Bonatti, 1985). Analog models showed further that the punctiformic patterns of incipient rifting takes place only when the obliquity of the tectonic extension is 15°–30° from the normal to the axial zone of the rift (Agostini et al., 2009; Mart and Dauteuil, 2000).
The northern part of the southern segment of the Levant Rift system is the Lebanese Baqa’a and its mountainous flanks of Mt. Lebanon and Mt. Anti-Lebanon. The trend of the Baqa’a is NNE–SSW, off the north–south orientation of most of the southern segment of the rift. The Lebanese segment is also distinguished by its anomalous elevation, which rises some 1500 m above the rest of the southern section of the system, and by the distribution of fan-shaped series of fault splay, which constrain the rift and transect its margins (Fig. 1). These faults show both normal and strike-slip offsets (Brew et al., 2001; Walley, 1988, 1998). Well-known among the faults is the 150-km-long Yammouneh Fault that bounds the rifted Baqa’a from the west in its southern section, then transects Mt. Lebanon off the northern Baqa’a, to connect with the Zawiya Fault that bounds the Ghab graben. A sinistral offset of 11 km of Pliocene basalts along the Yammouneh Fault was reported by Dubertret (1970). Marine Middle Miocene deposits along the eastern flanks of the Baqa’a Rift suggest that the uplift of that segment occurred mainly in Plio-Quaternary times (Dubertret, 1975; Homberg et al., 2010; Walley, 1998).
The northern segment of the Levant Rift system includes the Ghab graben, which branches off southwards from the Amiq–Hatay Rift in southern Anatolia. The Amiq–Hatay graben emerged from the East Anatolian Fault (Fig. 1) and was probably generated under a predominantly extensional tectonic stress due to the westwards motion of Anatolia (Boulton and Robertson, 2008; Mart, 2013).
In addition to the regional rift system, the Cenozoic geology of the Levant is characterized by an extensive fluvial system that drained northwestern Arabia to the Mediterranean Sea in the Oligocene, Miocene and the Early Pliocene (Horowitz, 2001, 2002). Abundant deposits of coarse conglomerates and sandstones (e.g., Buchbinder et al., 2005) and geological proof of buried deep canyons (Druckman et al., 1995) are evidence of these rivers, known in Israel as Hazeva Group. Since the general trend of the rivers was from east to west, and the oriental of the rift system is south to north, it is hard to imagine the co-occurrence of these two systems. Their coexistence during the Late Miocene–Early Pliocene, however, illuminates the tectonic regime of the Levant during this critical period.
This paper brings together the geological characteristics of the two segments of the Levant Rift system, to discuss their tectonic similarities and differences, to discuss their inter-relationship with the Hazeva fluvial system and to compare field observations with analog models, in order to suggest the comprehensive geodynamic evolution of the Levant since the Miocene. We shall strive to associate the geodynamic setting of the two segments of the rift system with the tectonics of Anatolia in the north and the Red Sea in the south, to accommodate the emergence of the Levant from the sea and to discuss some of the structural by-products of the closure of the last marine domain of the Tethys Seaway.
2 Geological setting
The geomorphological characteristic shared by many rifts on land and at sea is that the rift is embedded at the crest of a wide ridge, so that the rift and its mountainous flanks form a unified structural feature. Already during his visit to East Africa, Willis (1928) noticed that the East African Rift system comprises two conjugate ranges that nest a central rift, and jointly they form a single geological unit, and he wondered how a compressional ridge and an extensional rift could coexist. Later (Willis, 1938), he noticed similar tectonic settings also in the Levant. Heezen et al. (1959) encountered comparable features in the Mid-Atlantic Ridge and Vine and Matthews (1963) associated the ridge and rift systems of Carlsberg Ridge in the NW Indian Ocean with seafloor spreading. The morphological and structural similarity between the East African Rift and the Levant Rift system was noticed repeatedly (e.g., Gregory, in Picard, 1931; Wdowinsky and Zilberman, 1997).
In the Levant, however, the structural evolution of the rift and its flanks is only the third stage of tectonic uplift since it ascended from the sea in the Early Oligocene (Horowitz, 2002). The first uplift was not associated with rifting, but is linked to the East African mantle plume (Chorowicz, 2005; Moucha and Forte, 2011; Pik et al., 2008). The second regional uplift took place in the Middle Miocene, with the continental break-up of the Red Sea and the significant uplift of its margins. The last geomorphological rise occurred along the Levant Rift system, when mountain ascent and subsequent rifting started in the Late Miocene, and is still presently active (Mart et al., 2005).
The emergence of the Levant from the sea in the Oligocene led to the development of a series of large rivers that flowed from Arabia across the Levant to the contemporaneous Mediterranean Sea (Fig. 2). The rivers crossed a flat terrain that was tilted to the west, carrying in their load cobbles and sand, which were deposited en route as conglomerates and sandstones (Horowitz, 2002). The cobbles, which are predominantly calcareous, with chert and sandstones in places (Fig. 3) were probably eroded from terrains in northern-central Arabia (Sneh, 1982). Additional uplift of western Arabia due to the continental break-up along the Red Sea in the Middle Miocene further enhanced the flow of these rivers (Calvo and Bartov, 2001). But during the last stage of uplift and rifting, which consisted of the structural development of the southern Levant Rift since the Late Miocene, the fluvial system was gradually curtailed. Incipient grabens started to develop concurrently in the present domains of the Gulf of Elat, the Dead Sea, the Sea of Galilee and Lebanon, interrupting with the flow of some of the rivers, but rivers that flowed in between the emerging grabens and their rising flanks continued to supply sediments to the Levant Basin. The few rivers that kept flowing during the Messinian desiccation of the Mediterranean Sea incised deep canyons into their thalwegs due to the marine draw-down, and their erosional capacity, like that of the Nile, was greatly enhanced (e.g., Chumakov, 1973; Druckman et al., 1995; Mart and Ryan, 2008; Ryan and Cita, 1978). These entrenched canyons became deep fjords as the Mediterranean was reconnected to the Atlantic Ocean in the Early Pliocene, and seawater reached the emerging grabens of the Dead Sea and the Sea of Galilee. However, the continued uplift of the flanks of the grabens gradually curtailed the water flow, so that the amount of evaporation from these inland tongues of the ocean exceeded the seawater supply, the salinity rose, and thick layers of salt accumulated in both basins in the Early–Middle Pliocene (Horowitz, 2001; Inbar, 2012; Mart, 2013).

(Color online.) Conceptual reconstruction of the tracks of the fluvial system that flowed from northern Arabia to the Mediterranean Sea in the Late Miocene. Red lines mark the position of the faults bounding the incipient Elat, Dead Sea and Sea of Galilee grabens. The emerging rifts and their elevated flanks enabled the flow of the rivers running in between them at this stage.
Modified after Horowitz (1979) and Zak and Freund (1981).

(Color online.) Mid–Late Miocene fluvial conglomerates on the uplifted flank west of the Dead Sea graben are relicts of the extensive fluvial system that preceded the rift system, and, in places, was active concurrently with the evolving rift. The present topographic elevation is ca. 400 m.
The present fluvial flow from the Levant to the Mediterranean Sea is negligible (e.g., Negev, 1969), because the north–south-trending Levant Rifts and their mountainous flanks separate the Mediterranean from the fluvial domains of northern Arabia. However, the large rivers that drained northern Arabia to the Mediterranean Sea from the Early Miocene to the Early Pliocene (Horowitz, 1979; Zak and Freund, 1981; Fig. 2) left behind extensive deposits of sandstones and coarse conglomerates, known in Israel as Hazeva Group (Fig. 3). The thickness of the Hazeva exceeds 2000 m in some places, and even though the greatest thickness was measured in boreholes drilled in the rift floor south of the Dead Sea, it was suggested that the extensive deposition of the Hazeva sediments outside the rift pre-dated the rifting (Calvo and Bartov, 2001), and the original thickness of Hazeva Group was comparable to the thickness of more than 2.5 km that was measured in Arava 1 exploration Borehole, located ca. 30 km south of the Dead Sea (Calvo, 2012; Fleischer and Warshawsky, 2002). Indeed Horowitz (1979) suggested that prior to the rifting, the landscape of the Levant was that of a large and flat plain dipping gently westwards and transected by large rivers.
The gradual structural uplift of the mountainous flanks of the grabens caused the dwindling of the fluvial system to its final demise in the Pleistocene, so that the erosion caused by the uplift of the mountainous flanks of the rift system left behind only minor relicts of the extensive fluvial sediments, but some of these are located presently at mountaintops (Fig. 4). See also Horowitz (1979).

(Color online.) Sediments of a Miocene river channel on the crest of the Hatira Ridge at the western flank of the Dead Sea graben, west of the southern Dead Sea, looking westwards, at a topographic elevation of ca. 500 m. The orange line marks the base of the Miocene conglomerates outcrop.
Evidence that some of the large Hazeva rivers continued to flow during the Late Miocene and even the Early Pliocene was discovered in the coastal plain of southern Israel, where Druckman et al. (1995) encountered the Afiq and Ashdod deep canyons, that were incised into their thalwegs during the Messinian draw-down, and the Afiq defunct valley is nearly 10 km wide and 1 km deep. An isochron map of the Pliocene-to-recent interval shows that both Afiq and Ashdod rivers continued to carry sediments in the Early Pliocene, as well as the predecessor stream of the present Dalia Valley in northern Israel (Fig. 5). Comparable features were reported from the continental slope of North America (Vachtman et al., 2013).

(Color online.) Pliocene-to-recent isochron map of the SE Mediterranean, showing anomalous sediment accumulation in the upper continental slope off Afiq, Ashdod and Dalia rivers, suggesting that these Hazeva rivers still transported large quantities of sediment to the Mediterranean in the Early Pliocene. Insert: location map.
The Miocene fluvial system of the Levant co-existed with the emerging southern segment of the Levant Rift system in the Late Miocene–Early Pleistocene, where the scattered incipient grabens along the axial zone of the regional rift enabled the Hazeva rivers, flowing in the threshold zones, to continue their regimen. However, the gradual growth of the rift heralded the demise of the entire fluvial system. The last of the Hazeva rivers that crossed the rift and its margins and flowed from NW Arabia to the Mediterranean Sea was the Edom paleoriver (Fig. 2), that was dated to the Early Pleistocene (Avny et al., 2000; Guralnik et al., 2010).
A structural indication regarding the geodynamic regime of the northern section of the Levant Rift system is derived from GPS measurements of tectonic motion in the Middle East, which show that the displacement along Ghab and Amiq grabens and their flanks is to the northwest. Such trend could imply that Arabia might be pressing against the northern segment of the Levant Rift system and that the northern rifts should have developed under a compressional tectonic domain (Burke and Sengör, 1986). However, the regional display of the GPS measurements (e.g., Le Pichon and Kreemer, 2010; Reilinger et al., 2010) show that parts of Anatolia located west of the East Anatolian Fault are affected by the westwards pull that is discernible all over Anatolia, and increases towards the Aegean Domain (Chorowicz et al., 1999; Mart, 2013). It seems plausible that the domain of the northern Levant Rift is affected by differential extension trending westwards as well.
3 Analog modeling of oblique rifting
The abundant structural evidence for both normal and strike-slip faulting along the Levant Rift system suggests that the prevailing tectonic interpretation of the system as a “transform” (sic) is ambiguous. The grabens of the southern section of the Levant Rift system were considered to be pull-apart basins, formed by left-lateral displacement (Freund et al., 1970; Quennell, 1958), but the understanding that the rift is configured not only by strike-slip faulting but by normal faulting as well (e.g., Joffe and Garfunkel, 1987; Mart and Horowitz, 1981) cast doubt on that interpretation. Furthermore, the complex temporal co-occurrence between the westwards flowing Neogene river system of the Levant and the northwards trending regional rift and its elevated flanks was seldom discussed, but the apparent coexistence of the two dominant systems seems essential to clarifying the Levant tectonic setup. Consequently, laboratory experiments of analog structural models of oblique rifting were applied to investigate the evolution of the Levant Rift and fluvial systems, following the methodologies of Withjack and Jamison (1986), Davy and Cobbold (1991), and Tron and Brun (1991).
Oblique rifting comprises the composite interaction of lateral shear and extensional stresses that jointly affect brittle lithospheric material, with the possibility of additional structural contribution of diapiric ascent of underlying ductile matter along the axial zone of block separation. While the extension of the brittle crust produces normal faulting and the lateral shear creates strike-slip faulting, the combination of the two processes in oblique rifts produces series of structures of uplifted flanks, downfaulted grabens, and some rotation of the downfaulted blocks (Agostini et al., 2009). Such an assemblage of structures is drastically different from either normal or strike-slip faulted features.
The structures discerned in the analog models of oblique extension are determined by the angle of deviation of the extension vector from the normal to the plane of initial structural separation (Mart and Dauteuil, 2000). The analog experiments show that where these deviations are in the range of 30°, several small grabens develop simultaneously along an axial zone, separated by small transform faults (Agostini et al., 2009), which resemble the ENE–WSW escarpments in the axial zone of the central Red Sea (Bäcker et al., 1975; Le Pichon and Francheteau, 1978). As the deformation continues, the small rifts propagate along their long axis (Fig. 6). Where the scatter of the emerging grabens is nearly linear, they interconnect eventually, but if the initial grabens were offset considerably, a persistent transform fault connects the edges of the propagating grabens, where the length of that fault does not change with the continuation of the extension, as predicted by Wilson (1965).
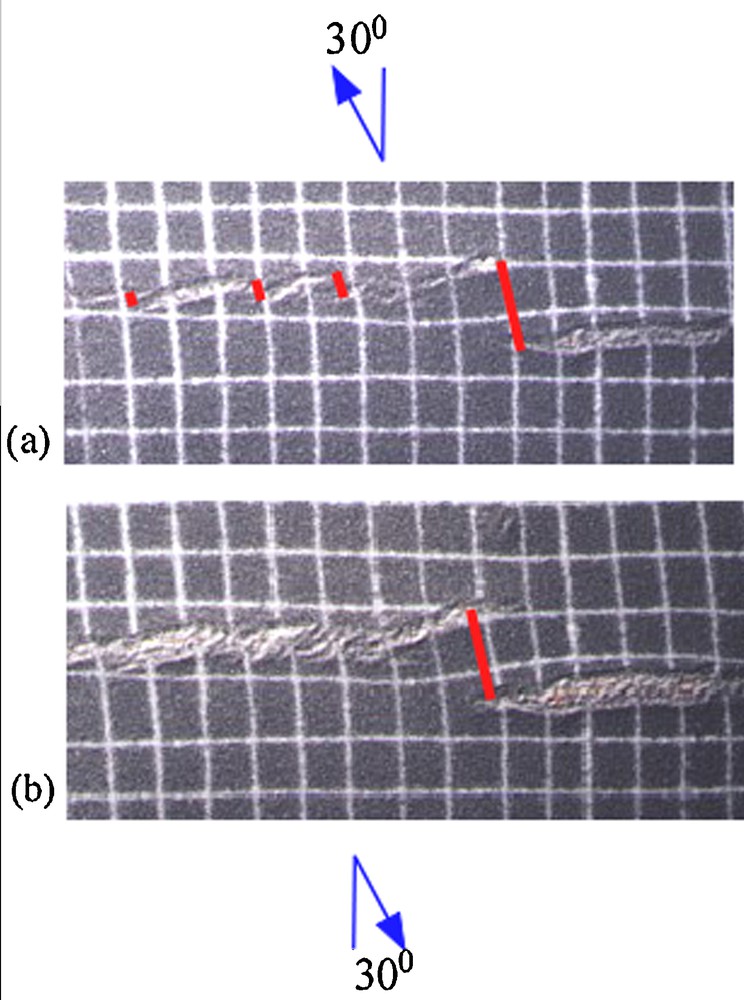
(Color online.) Analog models of oblique extension showing that changes in the obliquity of the extension led to variable structural products. Dark sand layers overly a layer of red silicone that floats on honey. The white squares on the sand are ∼2 cm across. Axes of the oblique extension are marked by red arrows. (a) Small grabens, set en échelon, form simultaneously at the early stage of rift formation under obliquity of 30°, with incipient transform faults connecting the edges of the grabens (yellow lines). (b) During the mature stage of 30° oblique extension, some of the early grabens merge, encasing the initial transform faults, but where the axial displacement of the grabens is large, the transform fault persists. Note that the length of the persistent transform faults remains constant.
Modified after Mart and Dauteuil, 2000.
The structures developed in the experiments of 30° oblique extension resemble the morphology and the geological setting of the southern segment of the Levant Rift system in the distribution of grabens of non-uniform dimensions along the axial zone, with thresholds between them. The gradual propagation of the grabens diminished the thresholds and enhanced the continuity of the regional rift (Agostini et al., 2009). If streams could have been introduced into the model, they would have continued to flow in the threshold zones between the propagating grabens until the grabens merged.
4 Discussion
The tectonics of the Levant Rift system has been debated since Picard (1931) considered it to be an extensional structure and Dubertret (1932) advocated that the rift is a product of large strike-slip faulting. Quennell (1958, 1984), Freund et al. (1970), Joffe and Garfunkel (1987), Klinger et al. (2000) and most of the tectonic studies of the Levant since 1970 supported the concept of large sinistral offset, whereas Schulman and Rosenthal (1968), Horowitz (1979, 2001) and Mart et al. (2005) advocated than the rift is primarily and extensional feature. In view of this prolonged and persistent debate, the present discussion will focus on the contribution of the investigation of the internal grabens, their effect on the Miocene–Pliocene fluvial system, and the contribution of the analog models of the internal structure of the rift to deciphering the geodynamic regime of the Levant since the Oligocene.
The internal grabens of the Levant Rift were considered to be pull-apart basins, the products of strike-slip displacement, while Lebanon Mountains were considered a restraining bend (e.g., Freund et al., 1970; Garfunkel, 1981; Quennell, 1958). The concept of pull-apart basin implies that all the geological series inside the basin must be younger than the basin; consequently, the occurrence of Cretaceous strata (Kashai and Croker, 1987) in the Miocenic Dead Sea graben (Joffe and Garfunkel, 1987), for example, is oxymoronic. On the other hand, measurements of crustal thickness in the Dead Sea graben encountered Moho at ca. 25 km, indicating crustal thinning (Ginzburg and Makris, 1979), probably due to the ascent of mantle diapirs under the rift (Rabinowitz et al., 1996), and thinned crust was measured under the zone of the Sea of Galilee as well (Ginzburg and Folkman, 1980). An alternative explanation to the evolution of the Dead Sea Graben was suggested by Ben-Avraham et al. (2010), who modeled numerically that the graben was formed by the drop-down of an isolated lithospheric block into the mantle. Unfortunately this conceptual model was not supported by data.
Correlation was encountered between the structural characteristics of the southern segment of the Levant Rift and the Red Sea. Seismic reflection investigations of the northern part of the Red Sea encountered a series of axial grabens there, and Bonatti (1985) suggested that such grabens were derived from the ascent of mantle diapirs along the axial zone, which produced the grabens by pushing the crust apart as a precursor of continental break-up. Northeast-trending escarpments that connect the edges of some of these grabens were considered to represent emerging transform faults (Bäcker et al., 1975; Le Pichon and Francheteau, 1978).
The prominence and subsequent demise of the Oligo-Miocene fluvial system of the Middle East is critical to reconstructing the geological history of the Levant Rift system. The paleogeographic and geological data indicate that several of the rivers that transected the region during the Oligo-Miocene became defunct due to the incipient structural development of the rift and its elevated flanks in the Late Miocene, but the rivers that persisted to flow in between the emerging grabens during the Messinian and the Early Pliocene were reconfigured by the Mediterranean draw-down, so that their channels deepened considerably. Druckman et al. (1995) discerned two Late Miocene canyons, one of them 10 km wide and 1 km deep, crossing the present southern coastal plain of Israel. The occurrence of Messinian evaporitic sediments inside these canyons established their age and their correlation with the Messinian desiccation of the Mediterranean Sea. Thus there is plausible similarity between the Hazeva canyons in the Latest Miocene and the deep contemporaneous entrenchment of the Mediterranean rivers, such as the Nile (Chumakov, 1973) and the Rhone (Fontannes, 1882).
There is therefore ground to presume that the deep Messinian canyons served as conduits of seawater to the inland when the marine regime was resumed. The occurrence of thick Pliocene salt deposits in the Dead Sea and the Sea of Galilee grabens was probably initiated when the structural uplift of the western flanks of these two grabens gradually affected the hydrological balance of the marine inlets, so that the quantity of the incoming seawater was less than the evaporation from the inland embayments. The Pliocene age of the salt in both Dead Sea and Sea of Galilee basins (Horowitz, 2001; Inbar, 2012; Karcz and Zak, 1987) and the occurrence of Hazeva deposits on mountaintops in Israel (Fig. 4) indicate that the structural development of the Levant Rift system occurred mostly during the Plio-Quaternary.
The Levant Rift system is commonly described as a hybrid of transform and strike-slip fault, proposing that the sinistral offset increased with time, in spite of it being the tectonic link between the Red Sea and the East Anatolian Fault. Quennell (1958) suggested that large valleys flowing into the southern segment of the rift faced each other in the past, and according to his correlation, they are located at present 107 km sinistrally apart from their original position. Quennell (1958) and Joffe and Garfunkel (1987) also presumed that the 107-km length of the rhomb-shaped (or pull-apart) basin of the northern Dead Sea supported their estimate of left-lateral displacement along the Levant fault. Freund et al. (1970) carried the concept further and correlated between outcrops of similar depositional facies of various stratigraphic periods from the Triassic to the Pleistocene, and suggested two stages in the 107-km lateral offset, a pre-Miocene 67-km stage, and a Neogene–Quaternary phase of 40 km. Freund (1970) also suggested that the 250-km separation between Africa and Arabia along the Red Sea emerging oceanic ridge splits towards the north, about 150 km comprise the slab divergence of the Suez Rift, and some 100 km were transformed into lateral displacement along the Dead Sea Rift. Garfunkel (1981) developed this concept further to suggest that the Levant Rift is a leaky transform fault, and dated the initiation of the sinistral displacement of 105 km to a time-span starting in the Middle Miocene.
While the normal faults that constrain the grabens of the Levant Rift indicate large vertical offset along these faults, there is also ample evidence of sinistral displacement as well. Left-hand horizontal offset along the rift is likely to drag loose blocks along the flanks of the rift due to friction, and evidence for local anticlockwise rotation was encountered along the western border of the Jordan Rift in the Galilee in the North (Ron et al., 1984) and near Timna in the South (Mart and Horowitz, 1981). Measurement of sinistral displacement along the southern segment of the Levant Rift was observed in the walls of the Le Chastellet (or Ateret) Crusaders’ castle in the Korazim threshold zone, which were displaced some 2 m sinistrally by two earthquakes that occurred in 1201 and 1547 (Ellenblum et al., 1998). Offset of landforms south of the Dead Sea led Klinger et al. (2000) to suggest a displacement rate of 4 mm/yr.
Questionable is the correlation of sites of similar sedimentary facies across the southern segment of the Levant Rift (Freund et al., 1970), because the general SSW–NNE orientation of the Triassic, Jurassic and Cretaceous isofacial zones (Druckman, 1977; Flexer and Honigstein, 1984; Hirsch and Picard, 1988) was not considered when offsets were estimated (Horowitz, 1979). Furthermore, Dubertret (1970) insisted that the lateral faulting along the Yammouneh Fault is only 11 km, so that the offsets suggested by Quennell (1958) and Freund et al. (1970) are incompatible with the geology of Syria and Lebanon. It seems therefore that the structural interpretation of the Levant Rift as a transform fault is ambiguous. While Freund (1974) distinguished specifically between transform and transcurrent faults, advocates of large sinistral offset along the Levant Rift system present evidence of strike-slip faulting, and consider the deformation zone to be a transform fault (e.g., Garfunkel, 1981; Girdler, 1990). The commonly used term “Dead Sea Transform” (e.g., Garfunkel et al., 2014) is ambiguous, evading the determination of the largest geological structure of the Levant to be transform fault, transform rift, or strike-slip fault.
Investigations of the northern section of the Levant Rift system, namely the Ghab and Amiq grabens, show evidence of an active tectonic regime of oblique rifting there, which includes both normal faulting and sinistral offset. Estimates of the lateral displacement of Plio-Quaternary structures are less than 20 km, and evidence of earlier offsets is dubious (Dubertret, 1970; Karabacak et al., 2010; Searle et al., 2010, 2011; Walley, 1998; Westaway, 2004, 2011). The rate of displacement, which can be tentatively gleaned from the sinistral offset of dated historic structures, is not very different from that inferred from GPS measurements, i.e. approximately 5 mm/year. A Roman aqueduct in the northern Amiq graben was displaced 13.6 m sinistrally by an earthquake dated to 30–70 AD, suggesting an offset rate of ca. 6.5 mm/year (Searle et al., 2011), somewhat faster than the measurements of the displacement along the southern segment of the rift system.
The northwestwards direction of the present geodynamic motion along the eastern side of the northern section of the Levant Rifts, discerned from GPS measurements (McClusky et al., 2000), led Westaway (2004) to suggest that the tectonic regime along the northern Levant Rift is transpressional, in spite of field evidence indicating transtensional regime (Boulton and Robertson, 2008; Searle et al., 2010). However, geodynamic analyses show that the northern section of the domain of the Levant Rift system is constrained primarily by the intensive westwards motion of Anatolia (Adiyaman et al., 1998; Le Pichon and Kreemer, 2010; Reilinger et al., 2010; Sengör et al., 2005). Mart (2013) argued that the rifting of the northern segment of the Levant Rift system, as well as the East Anatolian Fault, was generated by this Anatolian geodynamic process.
5 Conclusions
The Hazeva rivers configured the Levant hydrography in the Oligocene and the Early–Middle Miocene, and their gradual demise in the Late Miocene and the Pliocene depict the geodynamic processes that led to drastic variations in the regional landscape. The westwards-tilted plateau, where the fluvial system that drained northern Arabia prevailed in that time, was interrupted by the migration of the northern edge of the Red Sea continental break-up system eastwards, from the Suez to the Elat trend in the Late Miocene. Four grabens started to develop simultaneously in a linear axial zone that stretched northwards from the Red Sea, where the Elat, Dead Sea, Sea of Galilee and Lebanon eventually developed. The gradual subsidence of these structural grabens along the axial zone of the emerging rift and the uplift of their flanks interrupted the flow of the Hazeva rivers. However, rivers that flowed in the thresholds between the grabens continued to flow, a few of them prevailed to the Pliocene, and one survived to the Middle Pleistocene. Experiments in the frame of analog models suggest that the change in the landscape was caused when oblique rifting began to reconfigure the southern segment of the Levant Rift.
The few Hazeva rivers that existed in the Messinian were affected by the Mediterranean draw-down, and incised deep canyons into their thalwegs. When the Mediterranean water returned in the Early Pliocene, these canyons became fjords that led seawater to the Dead Sea and Sea of Galilee grabens of the rift system. However, as the grabens deepened and their flanks ascended further, the amount of seawater supply along the fjords decreased. The salinity of the water in the grabens increased and thick salt deposits were formed in the Pliocene basins of the Dead Sea and Sea of Galilee. The salt, which was dated by pollen in the Dead Sea and by interfingering basaltic flows in Kinneret graben, enabled the dating of the transition from the marine environment in the Dead Sea and Kinneret grabens to continent, and thus constrain the timing of the ascent of the flanks and the subsidence of the median valley of the Jordan Rift and Lebanese Baqa’a. The Amiq and Ghab grabens were formed in the Late Miocene, together with the growing offsets along the Anatolian faults.
We suggest that the Levant Rift system is the tectonic product of two unrelated geodynamic processes, the northward propagation of the Red Sea and the westwards migration of Anatolia. These processes configured the Levant and adjacent terrains since the Late Miocene and are still active.
Acknowledgements
This paper is the product of a prolonged research and numerous collaborations. The cooperation with Aharon Horowitz of Tel Aviv University, Olivier Dauteuil, and Jean-Pierre Brun of the University of Rennes-1 as well as with William Ryan of Columbia University is deeply appreciated. The reviews of Einat Aharonov and Yann Klinger were very constructive.