1 Introduction
Numerous ultramafic–mafic (UM–M) intrusions occur in various geological contexts and in the three crustal levels (lower, middle and upper) of the Pyrenean segment of the Variscan orogenic belt (Fig. 1) (Barnolas and Chiron, 1996). The origin of these intrusions and associated metamorphic and anatectic episodes is highly debated.
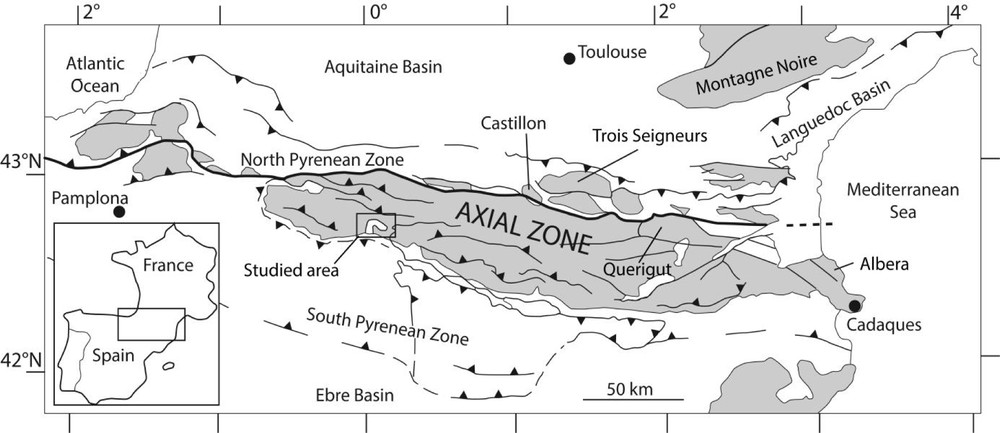
Schematic map of the Variscan segment of the Pyrenees, showing the location of the studied area (after Denèle et al., 2014 modified).
Within the lower crust, these rocks are associated with the granulitic rocks from the North Pyrenean Massifs (Driouch, 1997; Pin, 1989; Roux, 1977; Saint Blanquat, 1989; Vielzeuf, 1984). Pin (1989) suggested that the mafic rocks observed in some North Pyrenean Massifs (Saleix and Castillon) are derived from mantle magmas.
In the upper crust, these UM–M rocks are associated with large granitoid bodies, including the Querigut massif. Several authors (Ben Othman et al., 1984; Cocherie, 1984; Roberts et al., 2000) suggested that most of the granitoids originated from partial melting of crustal protoliths, while mafic rocks were derived from partial melting of the upper mantle.
Within the middle crust, UM–M rocks are associated with amphibolite-facies anatectic domes such as those of Albera (Cocherie, 1984; Driouch, 1997; Vilà et al., 2005), Céret (Cocherie, 1984; Driouch, 1997) and Gavarnie (Debat, 1965; Driouch, 1997; Driouch et al., 1989). Vilà et al. (2005) published the most detailed study and suggest that:
- • the different rock types reflect the fractional crystallization of a basaltic magma extracted from an heterogeneous mantle source;
- • the intrusion of basaltic magmas was contemporaneous with LPHT metamorphism and granitic magmatism;
- • the limited, but systematic, occurrence of broadly coeval basaltic rocks indicates that advective heat transfer from the mantle played a significant role in the LPHT metamorphism and extensive partial melting at various crustal levels.
The main aim of the present paper is to provide new data to extend the discussion initiated by Vilà et al. (2005). The data were acquired during the study of UM, M and intermediate rocks occurring within the metamorphic Gavarnie–Heas Variscan dome in the central Pyrenees (Soula et al., 1986). In this dome, ultramafic, mafic and intermediate rocks occur as:
- • blocks that are several meters in size and;
- • massifs that are several kilometers in size (Gloriettes, Troumouse and Aguila massifs (Debat, 1965; Debat et al., 1996; Driouch, 1997; Pouget et al., 1989)).
The origin and evolution of ultramafic (UM), mafic (M), and intermediate rocks emplaced within the metamorphic and anatectic series are defined in the light of new isotopic data (U–Pb zircon ages, and Sr, Nd isotopic ratios). Finally, the relationships between the evolution of the UM and M rocks, the metamorphism and structural features are defined in the frame of the Variscan orogeny.
2 Geological setting of the Gavarnie–Heas dome
The regional Gavarnie thrust sheet occurs on the southern side of the central Pyrenees (Bresson, 1903), where Variscan metamorphic and anatectic series covered by Upper Cretaceous limestones represent the autochthonous footwall (Fig. 2). These metamorphic and anatectic formations belong to a regional metamorphic dome, the Gavarnie–Heas–Barroude–Plan Larri dome, hereafter referred to as the Gavarnie–Heas dome (GHD) (Soula et al., 1986). The GHD consists of Infra-Silurian sequences affected by a prograde metamorphism (Debat et al., 1996) reaching amphibolite-facies conditions and partial melting in its central part (north of the Gavarnie and Heas valleys). The P–T–t evolution (Debat et al., 1996) defines a clockwise path with:
- • starting of partial melting at ∼ 650 °C and 0.55 GPa;
- • extensive partial melting at ∼ 730 °C and 0.43 GPa;
- • crystallization of first garnet and cordierite granodioritic leucosomes and later cordierite granitic leucosomes, indicating a decrease in temperature and pressure down to ∼ 600 °C and 0.25 GPa.
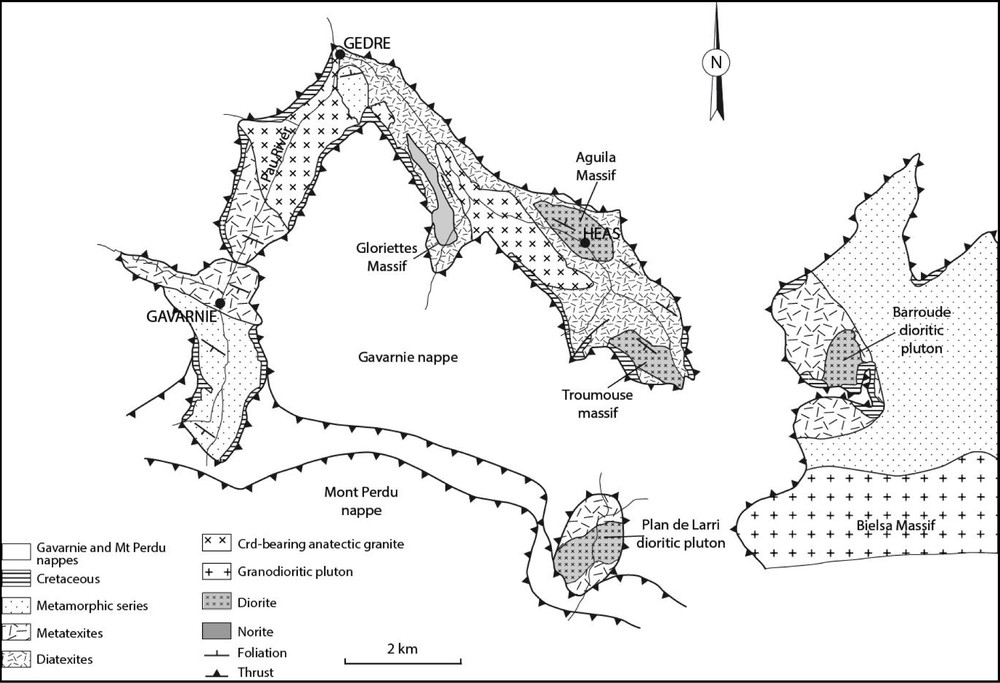
Map of the Gavarnie–Heas dome; location of the Gloriettes, Troumouse and Aguila ultramafic-mafic massifs.
The major foliation defining the domal regional structural pattern corresponds to:
- • a schistosity in the amphibolite-facies metasediments;
- • a layering with alternations of melanosomes and granodioritic leucosomes in the metatexites and diatexites;
- • a “gneissic” foliation in the granitic leucosomes.
Within the metamorphic and anatectic series, UM rocks occur as numerous blocks several meters in size while M and intermediate rocks occur as rare massifs several kilometers in size. The Gloriettes, Troumouse and Aguila massifs located in the Heas valley (Fig. 2) are the three biggest massifs and are the focus of the present study.
The Gloriettes massif is an ∼2-km-long and 0.4-km-wide (Fig. 2) sill interbedded within the N140° trending and ∼W70° dipping migmatites; it mostly consists of norite including ultramafic enclaves. The Aguila massif corresponds to an ∼1.5-km-long and 0.6-km-wide sill trending N120° and dipping ∼NE40° like the surrounding migmatites. The Troumouse massif is an ∼1.5-km-long and 0.6-km-wide body affected by a N140° trending and W70° dipping schistosity. The Aguila and Troumouse massifs mostly consist of mafic to intermediate rocks corresponding to gabbro-diorite and diorite in the geochemical classification TAS (Le Bas et al., 1986); and to diorite in the modal classification of Streckeisen (1976). In the following text, we use the term of diorite. Diorites include ultramafic and norite enclaves decametric to metric in size.
The three massifs are heterogeneously affected by a regional foliation. In the Gloriettes massif, the schistosity only develops in the rim of the massif across several tens of meters in width, while the central part of the massif remains unaffected. The schistosity underlined by metamorphic mineral associations (cummingtonite + anthophyllite + biotite + quartz) superimposed on magmatic mineral associations is parallel to the foliation of the surrounding migmatites. In the Aguila and Troumouse massifs, the schistosity of the mafic and intermediate rocks defined by the orientation of the magmatic components is parallel to the “gneissic” foliation of the surrounding anatectic granite, suggesting that the crystallizations of mafic and anatectic rocks were essentially coeval.
3 Petrography and geochemistry
The compositions of the major minerals are listed as supplementary data in Table A. UM rocks occur as enclaves ranging in size from a meter to several tens of meters mostly within the norites of the Gloriettes massif and more rarely within the Aguila massif. UM rocks always display the same mineral species (olivine, orthopyroxene, amphibole, and spinel), but with distinct modal proportions leading to distinguish peridotites, orthopyroxenites, and hornblendites. These UM consist of rounded olivine (Fo89–94) and orthopyroxene (En84) both included in abundant oikocrystic amphibole (MgO: 19 wt.% and Cr2O3: 4 wt.%).
The UMs of the Gloriettes massif show the following chemical composition: SiO2 (43.6–47.9 wt.%), Al2O3 (7.5–9.6 wt.%), MgO (18–23.9 wt.%), Mg# (79–84), and high Cr (1620–1819 ppm), Ni (126–1298 ppm) and Co (87–144 ppm) contents. The hornblendite occurring within the intermediate rocks in Aguila displays lower Mg# (73) and lower Cr (320 ppm) content, while its Ni (196 ppm) and Co (94 ppm) contents are within the range defined by other UMs. ΣREE range from 34 to 45 ppm, whereas (La/Yb)N range from 2.07 to 6.03, with no Eu anomaly (Fig. 3).
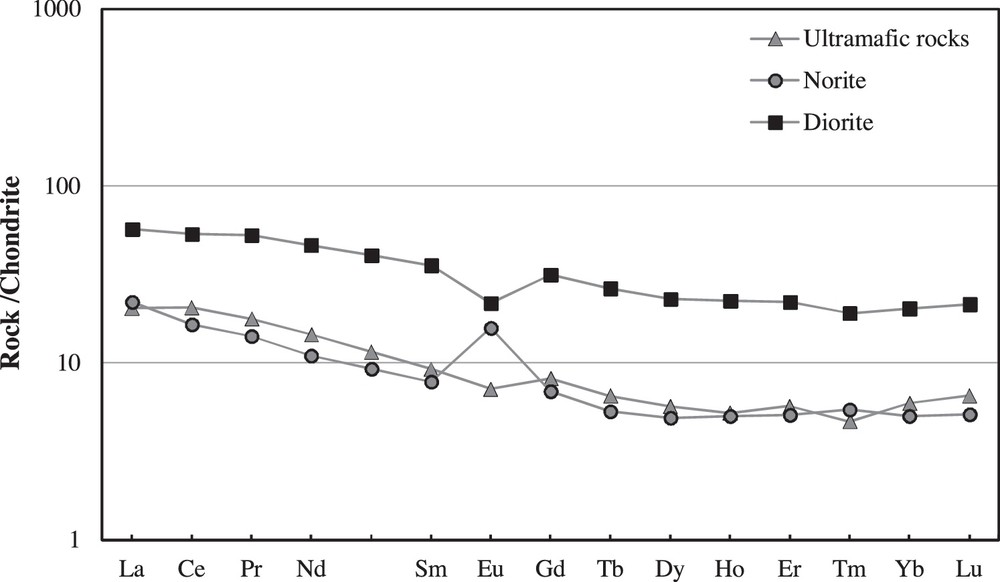
Chondrites normalized (Sun and McDonough, 1989) REE patterns of the UM and M.
The norites from the Gloriettes massif occur as enclaves several meters to several tens of meters in size within the intermediate rocks of the Troumouse and Aguila massifs. The norites consist of rounded orthopyroxene (En70), euhedral randomly oriented plagioclase (An85–90) both included in an oikocrystic magnesio-hornblende with Mg#: 80. The chemical composition of the norites from Gloriettes is homogeneous, with SiO2 (47–49 wt.%), Al2O3 (19–21 wt.%), MgO (7.9–10.4 wt.%), and Mg# (65–72). The Ni and Cr contents of the norites from Gloriettes range from 66 to 80 ppm and from 164 to 380 ppm, respectively. ΣREE range from 27 to 44 ppm and (La/Yb)N from 2.3 to 6.2. Eu anomaly is (Eu/Eu* = ∼1) (Fig. 3). No Eu anomaly is observed.
The norites from Troumouse (sample 10Trou06) are higher in MgO (13.9 wt.%, Mg# reaching 76) and lower in Al2O3 (12.9 wt.%). The Ni and Cr contents of the norites from Troumouse reach 296 ppm and 1100 ppm, respectively. ΣREE is 70 ppm and (La/Yb)N = 3.7. Eu anomaly is positive (Eu/Eu* = ∼2) (Fig. 3).
Mafic to intermediate rocks of the Troumouse and Aguila massifs have a granoblastic texture. These rocks consist of euhedral zoned plagioclase (An49–63), large poikilitic lamellae of magnesio-hornblende with Mg#: 70–80, biotite with Mg#: 52–62 and interstitial quartz of varying abundance. The composition of these rocks from Troumouse massif is SiO2 (49–52 wt.%), Al2O3 (17.4–20.9 wt.%), MgO (4.3–7 wt.%), and Mg# (49–62); the rocks from the Aguila massif are slightly more differentiated with SiO2 (54.4–62.2 wt.%), Al2O3 (16.5–18.8 wt.%), MgO (2.9–5.5 wt.%) and Mg# (40–60). Mafic to intermediate rocks (diorites) have high contents of REE with ΣREE ranging from 80 to 355 ppm and (La/Yb)N from 2–13. The Eu anomaly is negative (Eu/Eu* = ∼0.8) (Fig. 3).
The geochemical relations between the UM, M, and intermediate rocks appear in the Mg# vs. SiO2 diagram (Fig. 4) where the various rock types form a negative correlation line, with a clear hiatus between UMs and norites; this hiatus is also evidenced by the variations in Al2O3. It is coherent with the type of occurrence of the rock types, which are characterized by enclaves of poorly evolved rocks within more evolved rocks. The UMs, Ms and intermediate rocks from the Albera massif (Vilà et al., 2005) and the Querigut massif (Roberts et al., 2000) show the same trend as the UM and M rocks from the Gavarnie–Heas dome.
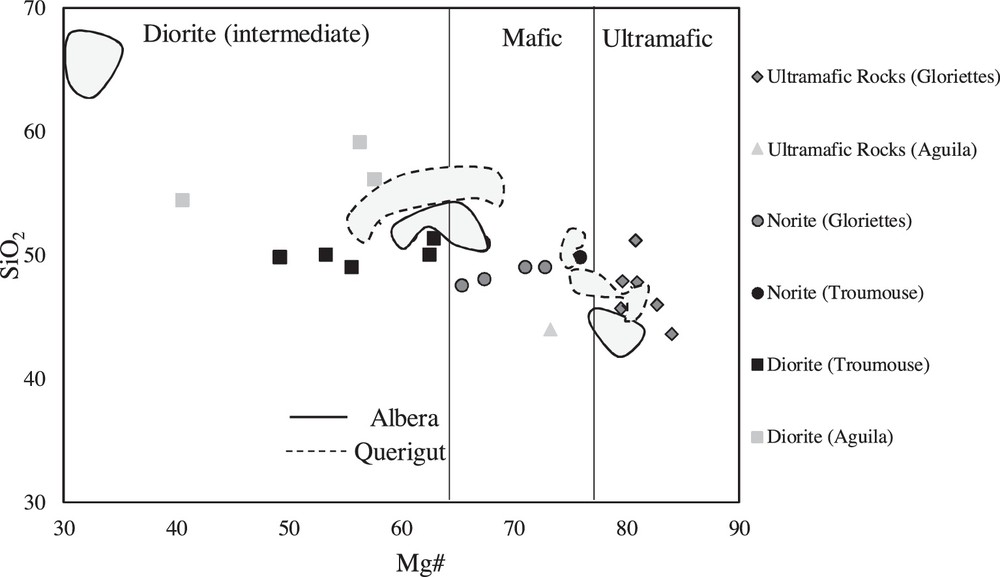
SiO2 vs. Mg# of the investigated rocks compared with those from other Pyrenean UM and M massifs: Albera Massif (data from Vilà et al., 2005), Querigut Massif (data from Roberts et al., 2000).
4 Geochronology and Sr and Nd data
4.1 U–Pb zircon geochronology
The ages of emplacement of the mafic rocks associated with the anatectic domes of the middle crust of the Variscan Pyrenees are poorly constrained. Three intermediate rocks were selected: two from the Aguila massif (10Ag02 and 10Ag03) and one from the Troumouse massif (10Trou02B); see supplementary data, Table B.
Thirty-two zircon grains (60 to 400 μm in size) from the two samples (10Ag02 and 10Ag03) taken from the Aguila massif were analyzed. Their U and Th concentrations range from 60 to 230 ppm and 20 to 120 ppm, respectively. Analyses of 68 U–Th–Pb spots show the same U–Pb age: 295 ± 1 Ma (Fig. 5A).
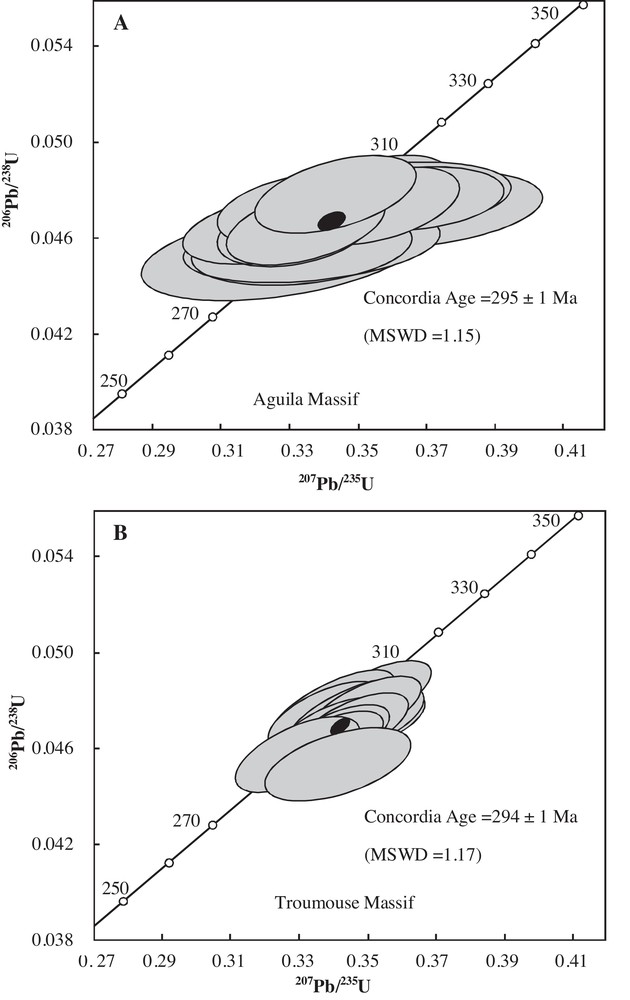
U–Pb concordia diagram for zircons from the diorite of Aguila (A) and Troumouse (B) massifs.
Nine zircon grains (50 to 550 μm in size) from one sample (10Trou02B) taken from the Troumouse massif were analyzed. U and Th concentrations, which are higher than in the Aguila samples, range from 150 to 460 ppm and 60 to 310 ppm, respectively. Analyses of 31 spots show the same U–Pb age: 294 ± 1 Ma (Fig. 5B). In both localities, this age of ca. 294 ± 1 Ma is interpreted as being the age of the diorite crystallization.
4.2 Sr and Nd isotopic data
Sr and Nd isotopic compositions (Table 1) are determined from a selection of 16 samples: eight samples from Troumouse, four from Gloriettes and four from Aguila.
Rb/Sr and Sm/Nd isotopic compositions of the Gavarnie–Heas dome. Repeated La Jolla and NBS 987 international standards give respectively 0.511850 ± 12 and 0.710255 ± 30. Typical blanks are 30 pg for Nd and 150 pg for Sr. The initial isotopic ratios are calculated using an age of 294 Ma.
Sample | Rb (ppm) |
Sr (ppm) |
87Rb/86Sr | (87Sr/86Sr)m ± 2 σ | (87Sr/86Sr)i (294) |
Sm (ppm) |
Nd (ppm) |
147Sm/144Nd | (143Nd/144Nd)m ± 2 σ | (143Nd/144Nd)i | ɛNd (294) |
Ultramafic | |||||||||||
10GLO05 | 23.4 | 78.7 | 0.832532 | 0.712621 ± 9 | 0.70898 | 1.62 | 6.7 | 0.146213 | 0.512133 ± 7 | 0.51184 | −8.85 |
10GLO06 | 22.6 | 37.7 | 1.679063 | 0.715871 ± 8 | 0.70854 | 2.3 | 8.8 | 0.158046 | 0.512068 ± 6 | 0.51175 | −10.58 |
10Ag04 | 31.1 | 128.5 | 0.677625 | 0.711988 ± 10 | 0.70903 | 1.9 | 9.1 | 0.126257 | 0.51211 ± 5 | 0.51186 | −8.52 |
Norite | |||||||||||
10GLO07 | 43.7 | 239 | 0.511959 | 0.712433 ± 9 | 0.7102 | 1.15 | 5.1 | 0.136352 | 0.512035 ± 6 | 0.51176 | −10.38 |
10GLO08 | 58 | 235 | 0.691078 | 0.712786 ± 8 | 0.70977 | 0.95 | 4.4 | 0.130559 | 0.512051 ± 6 | 0.51179 | −9.84 |
10Trou06 | 76.8 | 193 | 1.114243 | 0.713002 ± 7 | 0.70813 | 3.3 | 13.5 | 0.147824 | 0.512319 ± 8 | 0.51202 | −5.28 |
Diorite | |||||||||||
10Trou02B | 148.5 | 448 | 0.927921 | 0.710369 ± 8 | 0.70631 | 4.68 | 17.8 | 0.158996 | 0.512275 ± 6 | 0.51196 | −6.58 |
10Trou03 | 79.6 | 532 | 0.418862 | 0.71054± 7 | 0.70871 | 4.57 | 27.2 | 0.101601 | 0.512185 ± 5 | 0.51198 | −6.09 |
10Trou04 | 90 | 295 | 0.854213 | 0.712304 ± 8 | 0.70857 | 4.85 | 20 | 0.146645 | 0.512225 ± 6 | 0.51193 | −7.07 |
10Trou05 | 107.5 | 427 | 0.704799 | 0.710888 ± 8 | 0.70781 | 3.77 | 20.3 | 0.112304 | 0.512164 ± 6 | 0.51194 | −6.91 |
10Trou08 | 179 | 301 | 1.666187 | 0.719055 ± 9 | 0.71178 | 5.14 | 20.5 | 0.15162 | 0.512143 ± 8 | 0.51184 | −8.87 |
10Ag01 | 193 | 255 | 2.120726 | 0.719755 ± 9 | 0.71049 | 10.35 | 57.3 | 0.109224 | 0.512016 ± 8 | 0.5118 | −9.68 |
10Ag02 | 111 | 255 | 1.219046 | 0.714425 ± 10 | 0.7091 | 5.22 | 21.4 | 0.147503 | 0.512134 ± 7 | 0.51184 | −8.88 |
10Ag03 | 138.5 | 267 | 1.452886 | 0.715714 ± 9 | 0.70937 | 4.96 | 23.9 | 0.125495 | 0.512112 ± 5 | 0.51186 | −8.45 |
The isotopic signatures for Sr range from 0.710369 to 0.719755 and plot roughly on the calculated 294-Ma isochron (Fig. 6). Data from the Querigut massif including those from differentiated rocks (Roberts et al., 2000) define the same trend as the data from the present study, whereas data from the Albera massif, including also those from differentiated rocks (Vilà et al., 2005), clearly plot outside this trend (Fig. 6).
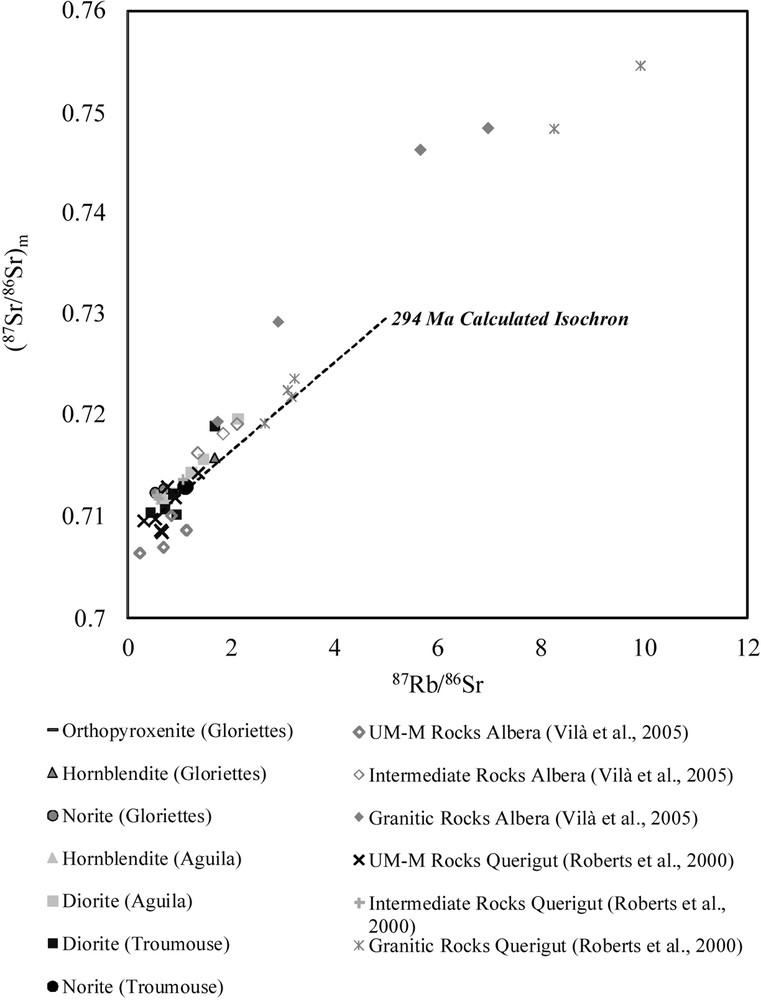
Sr isochron diagram for the measured samples plus litterature data from Vilà et al. (2005) and Roberts et al. (2000). The 294-Ma theoretical isochron is superimposed upon the data.
The isotopic signatures corrected using an age of 294 Ma are depicted in an ɛNd–(87Sr/86Sr)i diagram (Table 1). Age-corrected data from the Albera and Querigut massifs are superimposed on the same diagram (Fig. 7). The samples from the Troumouse massif show consistent negative values for ɛNd, whatever the type of rock considered (–5.3 to –7.6, average –6.6), except one diorite sample (10Trou08) that gives a more negative value (ɛNd = –8.9). On the other hand, these samples display very scattered (87Sr/86Sr)i ratios (0.70631 to 0.70871), and 0.71178 for the diorite sample (10Trou08).
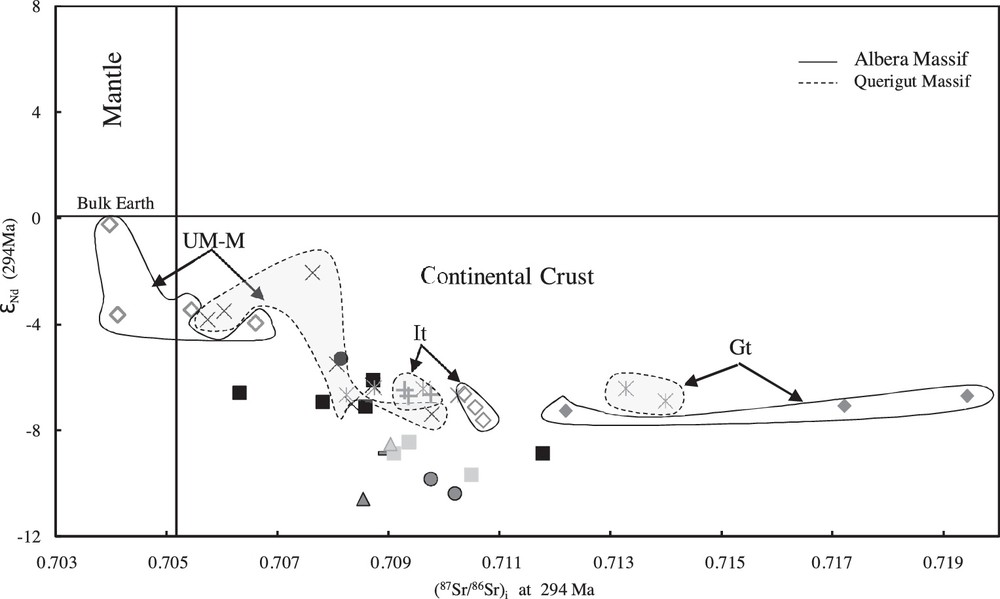
Plot of ɛNd vs. the initial (87Sr/86Sr)i ratio both corrected from an age of 294 Ma. Representative data from the other Variscan UM–M rocks from the Pyrenees are from Vilà et al., 2005 for the Albera Massif and from Roberts et al., 2000 for the Querigut Massif. UM–M: Ultramafic and mafic rocks; It: intermediate rocks; Gt: granitic rocks (symbols in Fig. 6).
Initial signatures for the Gloriettes and Aguila massifs display more negative values for ɛNd than those from the samples from the Troumouse massif, with ɛNd ranging from –8.5 to –10.6 and (87Sr/86Sr)i ratios from 0.70854 to 0.71049. Within each massif, there is no isotopic gap as a function of the rock type, but when we compare one massif with another, the samples from Troumouse clearly display the higher Nd radiogenic signature (ɛNd = –5.3 to–8.9). However, all the analyzed samples plot within the continental crust domain and display no evidence of a mantle component.
5 Discussion
5.1 Comparison with other occurrences of UM and M rocks emplaced within the Variscan middle and upper crust from the Pyrenees
UM and M rocks from the GHD dome display strong similarities with UM and M rocks from the Albera, and Querigut massifs:
- • similar relationships between the massifs and their surrounding migmatitic metasediments for the Albera massif;
- • similar type of occurrence with more primitive rocks included within more evolved rocks;
- • similar petrographic suite with peridotites, norites and diorites and mineralogical feature (lack of Cpx);
- • similar concentrations of major and minor chemical elements in whole rocks (Fig. 8);
- • similar relationships with regional metamorphism and structures.
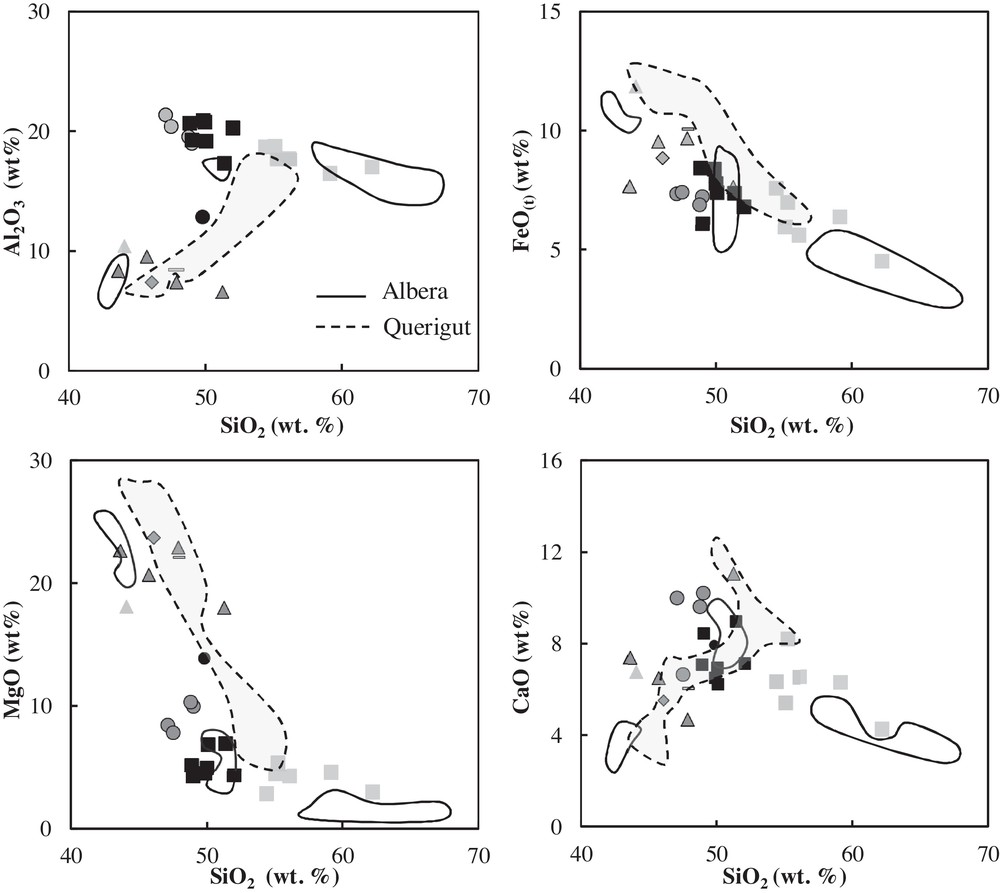
Major element Harker diagrams. The compositions of UM–M rocks from the Gavarnie–Heas dome are compared with the compositions of UM–M rocks from the Albera (Vilà et al., 2005) and Querigut massifs (Roberts et al., 2000).
On the other hand, the initial Nd and Sr isotopic compositions of the samples of the GHD dome are systematically enriched compared to those of similar rocks studied by Roberts et al. (2000) and Vilà et al. (2005). The spread of (87Sr/86Sr)i is less in our samples than in those cited in the literature, but that of ɛNd is clearly lower and plot within the continental crust domain, displaying no evidence of a mantle component.
Moreover, with regard to similar mafic rocks derived from mantle-originated magmas occurring in some North Pyrenean massifs (Saleix massif, with low (87Sr/86Sr)i ratios, between 0.70349 and 0.70676, and high ɛNd values, ranging from –5.3 to +2.2; Pin, 1989), there is a notable contrast between our data and these values.
5.2 Timing of the formation of the UM–M rocks from the Gavarnie–Heas Dome
To date, no U–Pb ages are available for the UM and M rocks occurring within the middle crust in the Axial Zone of the Variscan Pyrenees. Prior to the present study, Cocherie, 1984, obtained an age based on Rb–Sr whole-rock isochron at 282 ± 5 Ma (MSDW = 3.3) from the study of 14 samples of magmatic rocks taken from the Albera massif. Vilà et al. (2005, page 114) proposed the same age for the same massif, based on an Rb/Sr isochron, but did not totally exclude an age of 300 Ma by comparison with the age of the Querigut granitic massif (Roberts et al., 2000).
Our new La–ICPMS zircon U–Pb isotopic analyses revealed an age of 294 ± 1 Ma for the diorite crystallization in the Aguila and Troumouse massifs. This age is similar to that of the norite in the North Pyrenean Massif of Treilles (293 ± 14 Ma; Pin, 1989), and is clearly younger than the ages (307–301 Ma) obtained by U–Pb zircon dating of the granitoid plutons intruding the upper crust in the Variscan Pyrenees (Querigut, Carança, Mont Louis, Cauterets massifs; Denèle et al., 2014; Roberts et al., 2000). An age of 294 Ma indicates that the evolution of the structural-metamorphic GHD (retrograde metamorphism and crystallization of anatectic leucosomes with a simultaneous decrease in temperature and pressure, uplift and acquisition of the final shape of the dome) continued during the Permian.
5.3 Genetic links among the different lithologies and model of magmatic evolution
The Gavarnie–Heas UM and M rocks have unmatching petrographical, in one side, and geochemical, in the other side, characteristics. Indeed, the mineralogy of UM rocks is clearly ultramafic, with the occurrence of forsteritic olivine and enstatite, whereas these rocks display magmatic melt trace element contents (Fig. 3). Moreover, UM rocks show a cumulative origin, underlined by its high MgO (∼ 22 wt.%), Cr (∼ 1700 ppm) and Ni (425–1298 ppm) contents. Norite can be related to either mafic or intermediate magmas. They have relatively high SiO2 (48–50 wt. %) but also high Mg# (65–76), while displaying magmatic melt trace element patterns (ΣREE range from 27–70 ppm) and (La/Yb)N = 2.3–6. Norites share petrological characteristics of two types of melts: mantle-like and crustal-like, while having crustal isotopic signatures.
Diorites correspond to evolved magmas displaying intermediate chemical compositions (between basic and acid). Field relationships (norite enclaves within diorites) as well as chemical and isotopic features (clearly evidencing a continental crust origin for the later) allow us to propose that both diorites and norites are members of the same magmatic trend.
In summary, we have, on one side, UM–M rocks that can be considered as relics from a basaltic melt, strongly amphibole-metasomatized, and, on the other side, rocks that can be considered to derive from evolved, more silica-rich magmas.
Two different scenarios can be proposed for the genesis and emplacement of those lithologies:
- • evolution of mantle melts associated with crustal contamination (Assimilation ± Fractional Crystallization; AFC);
- • evolution of melt originating from a heterogeneous deep continental crust.
In the first scenario (AFC) melts, originated from the mantle, evolve within the continental crust and assimilate crustal material in order to explain, for example, their isotopic signatures (DePaolo, 1981; Taylor, 1980; Taylor and Sheppard, 1986). UM are early relics of mantle melts, despite displaying systematic crustal isotopic signatures, and norites and diorites are evolved mantle melts trapped at different levels during their fractional crystallization histories. This model is compatible with the high Mg# (65–76) of norite, which is in good agreement with the composition of evolved, mafic mantle melts; diorites can be the final product of such process. On the other hand, this model does not explain:
- • the unambiguous crustal isotopic signatures of the UM rocks, which is the opposite of what is expected in the AFC process;
- • the absence of contamination trends like in the studies of Vilà et al. (2005) and Roberts et al. (2000).
Indeed, in the present study, UM samples do not correspond to the isotopic pole, contrary to their equivalent in the Albera and Querigut massifs.
In the second scenario (melts products of a heterogeneous deep continental crust), the source of the melts is either heterogeneous at a small local scale or at a regional scale (Melting, Assimilation, Storage and Homogenization (MASH); Annen et al., 2006; Hildreth and Moorbath, 1988). The slightly heterogeneous, crustal isotopic signatures may be the final mixing products of melts coming from isotopically distinct regions, or the result of the melting of intrinsically heterogeneous sources. If the UM rocks cannot be generated by this melting process (they are too basic in terms of composition), they can be considered as relics of the melting process (mafic layers or blobs later impregnated by amphibolitic crustal melts). This process may explain the occurrence of poikilitic amphiboles enclosing ultramafic relics of Opx and Ol. Norites may result from melts produced by the melting of a hybrid source, more mafic than amphibolitic, or be also residues of a partial melting process, such as UM. Diorites are intermediate melts product of partial melting of a bi-modal continental crust source.
This model is in agreement with the crustal isotopic signatures and the occurrence of poikilitic amphiboles in the UM rocks. Poikilitic amphiboles are associated with metasomatic processes, where UM relics are percolated by hydrous crustal melts, enriched in trace elements and radiogenic isotopes. The association of the two components may explain the strong crustal isotopic signatures of these rocks. Finally, this model is compatible with the occurrence of dioritic magma. On the other hand, it does not explain the genesis of norites, both enriched in SiO2 and MgO, with a clear continental crust isotopic signature.
Finally, none model integrates all constraints. However, the strong crustal characteristics of all UM and M rock types from the GHD, lead us to favor the second model (MASH)-like.
6 Conclusion
In the central Pyrenees the early stages of the Variscan orogeny are dated to 319–316 Ma (Westphalian) by the deposition of flysch series (Culm) in foreland basins (Delvolvé, 1996). Tectonic phenomena (north–south shortening) are accompanied by the development of a thermal anomaly leading to partial melting of the mantle, granulitic metamorphism and locally partial melting within the lower crust as well as amphibolite-facies metamorphism and anatexis within the middle crust (Guitard et al., 1996; Vilà et al., 2005; Wickham and Oxburgh, 1986). Melts originated from a heterogeneous deep continental crust comprising metasediments and previously emplaced basic rocks move to the middle crust to crystallize the UM–M and intermediate rock types and to the upper crust to crystallize the granodiorites. The age of the emplacement of basic rocks in the lower crust is unknown. However, in the central Pyrenees, north of the GHD, flows of meta-basalts and meta-andesites are interbedded in the Upper Ordovician series (Calvet et al., 1988). These rocks could hypothetically represent volcanic equivalent of basic series first emplaced in the deep continental crust.
The new radiometric ages obtained from the diorite (294 Ma) of the GHD indicate that the Variscan Pyrenean orogeny continued during the Early Permian i.e. after the emplacement and crystallization of the large granodioritic massifs (∼ 300 Ma) (Denèle et al., 2014). In the Axial Zone of the Pyrenees partial melting within the deep crust, emplacement and crystallization of UM–M and intermediate rocks, metamorphism and anatexis within the middle crust span at least 30 Ma (between 320 and 290 Ma).
Acknowledgements
We are grateful to M. Seyler and G. Ceuleneer and to the reviewers P. Barbey and M. Vilà for their constructive comments and to M. Chaussidon for his editorial work and constructive discussions. We finally thank A.-M. Cousin for her assistance with drawing the figures.