1 Introduction
In large-scale humid subtropical regions, aquifer discharge occurs mainly in streams and rivers, ensuring the maintenance of baseflows. Baseflow dynamics provide important aquifer storage information, where groundwater recharge is normally the main source of inflow. Therefore, in accepting that baseflow estimates are equivalent to groundwater recharge, it must be assumed that all groundwater flow discharges into rivers of the basin in question (Healy, 2010). This assumption has already been used in a wide variety of groundwater recharge studies (Cartwright et al., 2014; Lee and Risley, 2002; Risser et al., 2005; Zomlot et al., 2015), which showed results similar to other recharge methods, yet it still must be validated through comparison with other methods in order to provide a reasonable estimate of recharge (Huet et al., 2016). Some studies have shown that baseflow obtained by digital filters could result in higher values due to inclusion of non-strictly groundwater derived discharge (interflow) (Ebrahim and Villholth, 2016; Lee and Risley, 2002).
The understanding of the term ‘baseflow’ sometimes varies between groundwater and surface-water researchers. Some groundwater studies would define baseflow as water discharged from the saturated zone, while in other studies, baseflow may be regarded as all streamflow that is not derived directly from surface runoff (Xu, 2011). However, it is a fact that the hydrographs are a combination of three components originated from groundwater, interflow, and surface discharges (Freeze and Cherry, 1979; Maidment, 1992).
Our study has been focused with special attention to baseflow dynamics, using three different appropriate methods (see Updating presentation of these different methods in the Supplementary Material), all based on surface-water data.
The study is devoted to the Serra Geral Aquifer System (SASG), which is the main source of drinking water for the State of Paraná, Brazil, Southern South America. The aim was to understand the interaction between aquifers and rivers for large-scale subtropical basins by comparing the application of different groundwater recharge estimation methods, and to understand how baseflow is represented through hydrograph separation and hydrological modelling perspectives.
2 Material and methods
2.1 Study area
The area is comprised of two watersheds over SASG, the São Francisco Verdadeiro (SFV) (1506 km2) and the São Francisco Falso (SFF) (506 km2), as shown in Fig. 1.
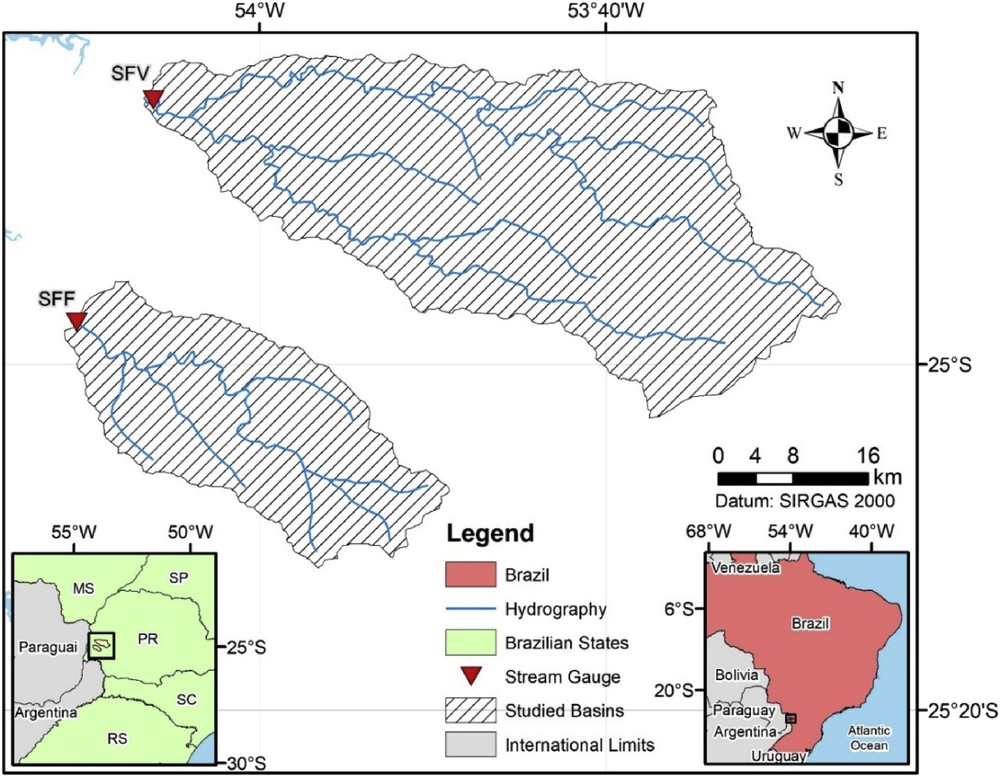
Location of watersheds used in the study.
To understand groundwater recharge and to interpret the results of each method, a conceptual model was formulated. Some physical characteristics such as climate, geology, soils type, topography, hydrology, and land use are factors in determining the occurrence of recharge (Healy, 2010; Reginato and Strieder, 2006). As such, the physical aspects of the watersheds were also taken into account.
2.1.1 Relevant physical characteristics to understand groundwater recharge occurrence
According to Köppen's climate classification (Kottek et al., 2006), the watersheds have a humid, temperate climate with hot summers (Cfa). Annual evapotranspiration is between 1000 and 1200 mm, while average annual rainfall is around 1719 mm, as shown in Fig. 2A. Monthly rainfall does not vary considerably over the year, and given the hydrogeological conditions, recharge can occur throughout all year. Evapotranspiration values vary according to the seasons. Higher values were observed in summer, which indicates that recharge is less favored during this period (Iapar, 2017; Pinto et al., 2014).

Annual rainfall (A). Slopes (B). Land use (C). Soil type (D) (Binacional, 2014; Embrapa, 2007; Ladeira Neto, 2013; Pinto et al., 2014).
Aquifer volcanic rocks represent one of the largest basalt areas on the planet; basalt-andesites are also found and contrast with rhyolites, rhyodacites, and latites (Nardy et al., 2002; White, 1906; Wildner, 2004). The rock aquifer system's thickness is approximately 1000 m (Athayde, 2008) and covers the entire study area. Located below this system is the Guaraní Aquifer System (SAG). Some studies show the possibility of occurrence of SAG discharge zones into the studied rivers, especially around an altitude of 238 m (Athayde, 2008; Fernandes et al., 2006; Portela Filho, 2003; Souza, 2004).
Topography plays an important role in the distribution of groundwater recharge. Steep slopes tend to have low infiltration rates and high runoff rates (Healy, 2010). Both of the studied watersheds have different characteristics. SFV watershed is mostly located over Cascavel Plateau, a medium dissection region, where tops are elongated and flattened, convex slopes are less than 12%, and valleys are V-shaped. The SFF watershed is entirely located over São Francisco Plateau, where dissection is also medium, tops are only elongated, convex slopes are less than 30%, and valleys are V-shaped (Mineropar, 2006). Both watersheds have their springs and outlet in similar altitudes, around 660 and 230 m, respectively. However, the SFV's main river is 133 km long, while SFF presents only 61 km. The SFF watershed also contains slopes much higher than those observed in the SFV watershed (Weber et al., 2004), as shown in Fig. 2B.
Vegetation and land use can have effects on the recharge processes as well. Vegetated land surface typically has higher rates of evapotranspiration (and, hence, less water available for recharge). Excess irrigation also could constitute significant amounts of recharge, whilst urban areas contain impervious areas that can inhibit recharge (Healy, 2010). The SFV watershed is predominantly devoted to agriculture (72%) in flatter areas, with portions of forests (13%), fields in steeply sloped areas (11%), and a small portion of urban areas (4%). The SFF watershed has a predominance of fields (40%) in steeply sloped areas and agriculture (39%) in flatter areas, with portions of forests (20%), and a small portion of urban areas (1%), as shown in Fig. 2C.
Both watersheds present different soil characteristics. The SFV has a predominance of Latosols (48%) with depths of 2 m and Nitosols (41%) with depths between 1 and 2 m, both located in the less dissected area where the elongated and flattened tops occur. Small portions of Neosols (10%) are also found in areas with greater slopes of depths lower than 50 cm. The SFF has a predominance of Nitosols (47%), with depths between 1 and 2 m closer to the drainage areas, and Neosols (33%) with depths lower than 50 cm located mainly over tops and more dissected regions, as shown in Fig. 2D (Embrapa, 2007, 2012). Latosols and Nitosols of the (deeper) SFV have a higher water storage capacity and lower generation of surface runoff, whereas the SFF Neosols have a low storage capacity and the capacity to generate high surface runoff (Fan et al., 2015). Therefore, the SFV soils display greater favorability to the occurrence of groundwater recharge.
The SFV and SFF rivers are perennial, indicating that they are a natural outflow for local aquifers. Distal periods of river recession were analyzed and showed the SFV river to have greater baseflow contributions during these periods (a constant recession lasting 79 days) than the SFF river (29 days of constant recession), which ensures a greater capacity to regulate the SFV rivers.
2.2 Groundwater recharge estimate methods
2.2.1 Soil-water budget
The soil-water budget method for estimating groundwater recharge is derived from a control volume such as a watershed, aquifer or soil, in which all components are measured or estimated, then recharge is attributed to the residue (Healy, 2010). The water balance equation obeys the principle of mass conservation, or continuity principle, where the difference between inputs and outputs is equal to the variations in the system storage (Feitosa et al., 2008). In this case, a simplified water-budget equation was used to represent the soil control volume, as shown in Eq. (1).
(1) |
In Eq. (1), P is the rainfall (mm), ETR is the actual evapotranspiration (mm), ES is the surface runoff (mm), R is the groundwater recharge (mm), and ΔS is the soil storage variation (mm).
This equation (Feitosa et al., 2008) considers the main components of the water balance. However, despite their simplicity, such terms are not always easily or adequately quantified. The uncertainties in estimations using water balance in humid regions are small compared to the total groundwater recharge, being suitable for time scales of one year or more, mainly due to soil moisture balance (de Vries and Simmers, 2002; Hirata and Wahnfried, 2005). A scale of years was employed in this case, and groundwater recharge values were obtained for the period from 2000 to 2014; since the method provides an average recharge value, a large number of years are necessary to reduce the uncertainties occasioned by annual changes in soil storage, which are governed mainly by interannual variability in precipitation, and the steady-state condition was assumed for the soil storage variation. In order to obtain recharge as a residue, precipitation, actual evapotranspiration, and surface runoff were obtained independently.
Precipitation was obtained directly from two rainfall gauges from the Brazilian Water Agency (Brasil, 2016), both gauges (2554023 and 2453026) located inside each of the researched watersheds. Actual evapotranspiration values were obtained from MOD16 (Mu et al., 2011), which is based on the Penman–Monteith method and adapted to be used with remote sensing data. Meteorological data and energy balance were obtained from reanalysis, while land use data were obtained from MODIS. A few works have already used MOD16 to obtain groundwater recharge estimates (Jovanovic et al., 2015; Lucas et al., 2015; Rawat et al., 2012). Surface runoff values were obtained using the HEC-HMS rainfall–runoff model (Feldman, 2000), where the Soil Moisture Accounting (SMA) model (Bennett, 1998) was used to represent the soil. Both watersheds were calibrated and validated through the observed data, and only the portion that represents surface runoff was extracted from the model and used for the proposed water-budget; the results of this further analysis can be found in the Supplementary Material.
2.2.2 Hydrograph separation
Groundwater recharge methods attempt to quantify the water added to the water table, whereas baseflow methods separate the part of streamflow hydrograph attributed to groundwater discharge (Risser et al., 2005). The water-budget equation to estimate the groundwater recharge for an aquifer is as follows (Healy, 2010):
(2) |
In Eq. (2), R is the recharge, is the change in groundwater storage, is the baseflow, is the groundwater evapotranspiration, and () is the net groundwater flow out of the aquifer, which includes pumping and inter-aquifer flow.
Assuming that groundwater recharge is equal to baseflow is equivalent to assuming that all other terms are negligible, which would mean that there is no change in aquifer storage, no exchanges of water with the underlying aquifers, no underflow, no withdrawal or injection of groundwater, and no groundwater loss to evapotranspiration (Healy, 2010). Studies have showed that annually 29.5 mm and 1.1 mm of water are pumped from the SFV and SFF basins (Melati, 2018); however, typical data available in South America at a watershed scale display a lack of available information and does not allow one to understand the magnitudes of the other terms. The assumption that all groundwater recharge discharges along the river channels can also lead to errors, as there is additional regional discharge that can occur due to watershed gradients (Freeze and Cherry, 1979; Sophocleous, 2002). Yet many studies applied this method and found results comparable to those of other hydrogeological methods (Risser et al., 2005), and this is the assumption employed here.
The portion of the hydrograph that represents baseflow can be approximated using different techniques (Chow et al., 1988). A widely used technique is digital filtering (Eckhardt, 2005; McMahon and Nathan, 1990). Although digital filtering is a purely empirical approach, it removes much of the subjectivity that occurs in manual separation, providing consistent, reproducible results (Healy, 2010). The digital filter proposed by Eckhardt (2005) was used, based on the fact that its parameters are recession constant, which is usually obtained by recession analysis and the BFImax parameter, which represents the maximum baseflow index value that can be modelled by the algorithm. The recession constant has a stronger influence on the calculated baseflow than the BFImax parameter (Eckhardt, 2012). The filter is presented in Eq. (3):
(3) |
In Eq. (3), is the baseflow at the time step, and are model parameters, is the baseflow at the time step before, and is total river flow at the time step.
Recession constant analysis can be obtained using different techniques. The RECESS program (Rutledge, 1998) allows the constant to be obtained by hydrograph for long periods of time. It is manually possible to obtain the constant when considering that aquifer discharge has a linear relationship with its volume during recessions. Using two discharge values from the hydrograph and the time between them allows for a reasonable estimate of the recession constant (Collischonn and Dornelles, 2013). To obtain the recession constant for each watershed, the “baseflow filter for flow gauges” tool, present in the MGB–IPH hydrological model (Collischonn et al., 2007; Pontes et al., 2017), was used in this study.
The BFImax parameter cannot be measured and, as such, subjectivity is often involved in its obtainment. Studies show that it might be possible to find a typical BFImax value using hydrological and hydrogeological characteristics (Eckhardt, 2005). However, recent studies have shown that this approach is not always appropriate (Collischonn and Fan, 2013; Zhang et al., 2017). A recent contribution (Collischonn and Fan, 2013) proposed a method of obtaining BFImax based entirely on discharge records using a backwards running filter. The aforementioned authors proposed two different ways to estimate BFImax, one based on the application of the inverse filter (IF), and the other on the Q90/Q50 ratio (QR).
2.2.3 Hydrological modelling (MGB–IPH)
The semi-distributed MGB–IPH rainfall–runoff model was initially developed to simulate large basins (Collischonn et al., 2007; Pontes et al., 2017), although, the model has been used for various other applications in smaller Brazilian basins (Felix and Paz, 2016; Monte et al., 2016; Sorribas et al., 2012). In this case, the model information from groundwater compartments was evaluated in order to obtain groundwater recharge indirectly.
The MGB–IPH model is composed of modules to calculate the soil-water budget, evapotranspiration, flow propagation, and flow routing through the drainage network. Areas with similar characteristics (land use and soil type) were grouped in the same hydrological response unit (HRU), where each soil-water budget is computed. Each catchment that composes a watershed contains a limited number of distinct HRU. All flow s(surface, interflow and groundwater) generated from the soil-water budget for each HRU are summed, routed to the stream channel, and then routed further through the river network (Collischonn et al., 2007; Fan and Collischonn, 2014). Fig. 3 presents a simplified schematic representation of the MGB–IPH model.
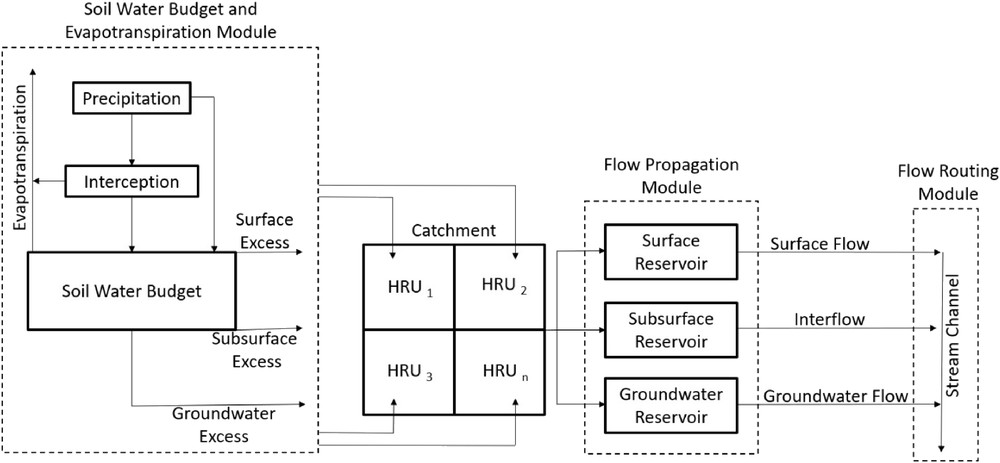
Simplified schematic representation of the MGB–IPH model. Adapted from Pontes et al. (2017).
The hydrometeorological data and physiographic characteristics of the watersheds are required as inputs. Precipitation and stream flows were obtained from the Brazilian National Water Agency network (available at <hidroweb.ana.gov.br>). Climatic variables for Penman–Monteith evapotranspiration were obtained from the Brazilian National Institute of Meteorology (Brasil, 1992). Physiographic characteristics such as division watersheds, river reaches, and hydraulic river characteristics were obtained from a digital elevation model (DEM), taken from the Shuttle Radar Topography Mission (SRTM). This model was provided by the National Aeronautics and Space Administration (NASA), and computed using the IPH-Hydro Tools program (Siqueira et al., 2016), a tool of the MGB–IPH model. In order to obtain each HRU, soil types were taken from the Brazilian Agricultural Research Agency (Embrapa, 2007) and classified as deep (Latosols and Nitosols) and shallow (Neosols), while land uses (Binacional, 2014) were classified as agriculture, forests, fields, wetlands, and urban areas, resulting in 8 HRUs. The MGB–IPH model calls for both fixed parameters and parameters requiring calibration to be input. Both of these were based on the HRU classification.
The MGB–IPH model was calibrated manually for both watersheds (SFV and SFF), given temporal data limitation. The first half of the data was used for calibration, and the second half for validation in both watersheds. A daily time interval was used for the simulations. The SFV watershed was calibrated from January 1996 to December 2001 and validated from January 1989 to December 1995. The SFF watershed was calibrated from January 2009 to December 2014 and validated from January 2002 to December 2008.
In order to evaluate calibration and validation, the following efficiency indicators were used: Nash–Sutcliffe coefficient (NS), Nash–Sutcliffe coefficient using discharge logarithms values (NSlog) and relative streamflow volume error (ΔV). The equations can be found in the Supplementary Material.
The calibration of SFV and SFF showed NS of 0.83 and 0.65, NSlog of 0.82 and 0.70 and ΔV of −0.90% and 19.90%, respectively. Validation for SFV and SFF showed NS of 0.75 and 0.66, NSlog of 0.76 and 0.71, and ΔV of −1.60% and 13.30%, respectively. The observed and simulated MGB–IPH hydrograph for a short period of time can be found in the Supplementary Material.
To avoid that the results obtained are biased by the parameters of the chosen mode, we also performed a further model evaluation analysis considering 500 of the optimized calibration parameters as set. The results of this further analysis can be found in the Supplementary Material. These tests revealed that all the possible parameters set, which have the same equifinality as the model calibration, generate consistent values with the ones adopted for the study.
For calibration and validation of hydrological models, the efficiency indicators obtained were considered acceptable.
3 Results
3.1 Water budget
Surface runoff, actual evapotranspiration and precipitation obtained for the water budget method proposed can be found in the Supplementary Material for both watersheds.
The average results obtained and their coefficient of variation indicate a groundwater recharge of 530 ± 170 mm and 352 ± 141 mm for the SFV and SFF watersheds, respectively. It is important to note that the method applied in this instance does not differentiate interflow and groundwater discharges. Instead, both are treated as a single discharge. The SFV watershed presents a higher average recharge (28%) in relation to the total annual precipitation when compared to the SFF watershed (19%).
3.2 Hydrograph separation
The Eckhardt hydrograph separation filter was applied to observed and simulated (from MGB–IPH) data at both researched watersheds, using two different approaches to obtain the BFImax parameter (QR and IF). The BFImax and recession constant values computed, with the results of annual groundwater recharge, can be found in the Supplementary Material.
Considering the QR BFImax method, average results and their obtained coefficient of variation indicate groundwater recharges of 516 ± 169 mm and 289 ± 90 mm for the SFV and SFF watersheds in observed data, respectively, and 583 ± 147 mm and 317 ± 93 mm for the SFV and SFF watersheds in simulated data, respectively. The SFV watershed presents an average recharge of 26% in relation to the total annual precipitation, compared to 15% of the SFF watershed for the observed data, and 30%–17% in the simulated data. Considering the IF BFImax method, average results and their obtained coefficient of variation indicated groundwater recharges of 642 ± 209 mm and 319 ± 99 mm for the SFV and SFF watersheds in the observed data, respectively, and 642 ± 157 mm and 401 ± 118 mm for the SFV and SFF watersheds in the simulated data, respectively. The SFV watershed presents an average recharge of 33% in relation to the total annual precipitation, compared to 17% of the SFF watershed for the observed data, and 33%–21% in the simulated data. The observed hydrograph data with baseflow results for both watersheds can be found in the Supplementary Material.
3.3 Hydrological modelling (MGB–IPH)
Discharges from the three different MGB–IPH reservoirs (surface, subsurface and groundwater) were extracted and are displayed in Fig. 4.
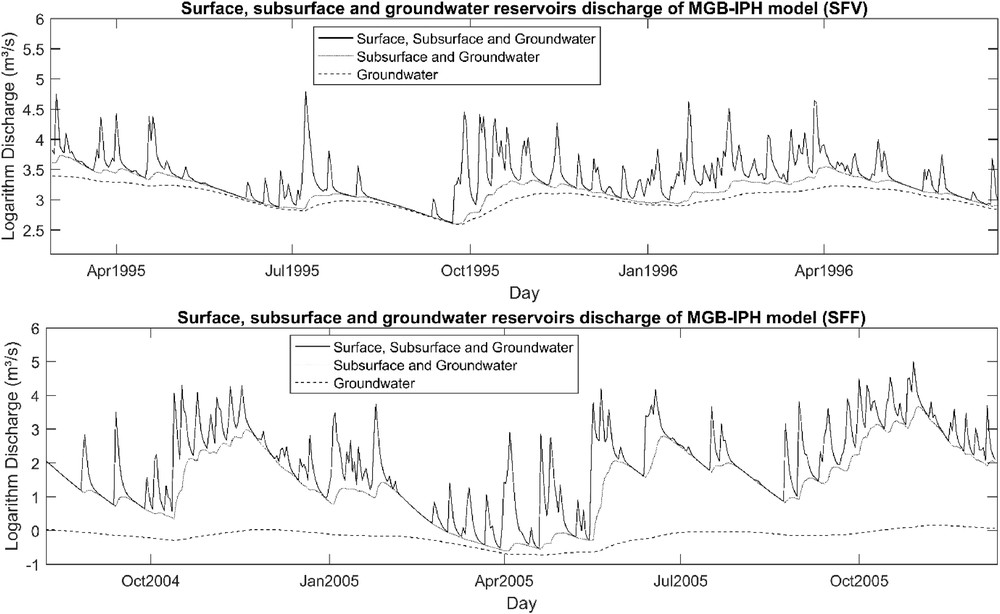
Logarithm discharges from the surface, subsurface, and groundwater MGB–IPH reservoirs.
It was verified that, in the SFV basin, groundwater discharges are more representative in dry periods, whereas, in the SFF basin, we have a division between interflow and groundwater discharges over these same periods. Annual discharges for the period between 1975 and 2015 were computed; the averages and their variation coefficient were considered to estimate the groundwater recharge; the results can be found in the Supplementary Material.
Considering recharge based on discharges from subsurface and groundwater reservoirs, the average results and their variation coefficient indicated groundwater recharges of 600 ± 161 mm and 373 ± 187 mm for the SFV and SFF, respectively. The SFV presented an average recharge of 32% in relation to the annual precipitation, compared to 21% within the SFF. When considering recharge based only on discharges from the groundwater reservoir, the results indicate groundwater recharges of 489 ± 95 mm and 48 ± 6 mm for the SFV and SFF. The SFV presented an average recharge of 26% in relation to annual precipitation, as compared to 3% of the SFF.
For the SFV, watershed groundwater reservoir contribution is quite representative. Having periods with little or no rain, stream flows are represented mainly by aquifer discharges. The SFF watershed presented a slightly different behavior. Discharges from the groundwater reservoir represented only a fraction of stream flows in periods with less rain. The interflow makes up a more representative fraction of stream flows.
3.4 Comparison of the groundwater recharge results
The average groundwater recharge results obtained by water budget, hydrograph separation, and hydrological modelling are present in Fig. 5, and annually in Figs. 6 and 7. More information about the results can be found in the Supplementary Material.

Groundwater recharge results for SFV and SFF.
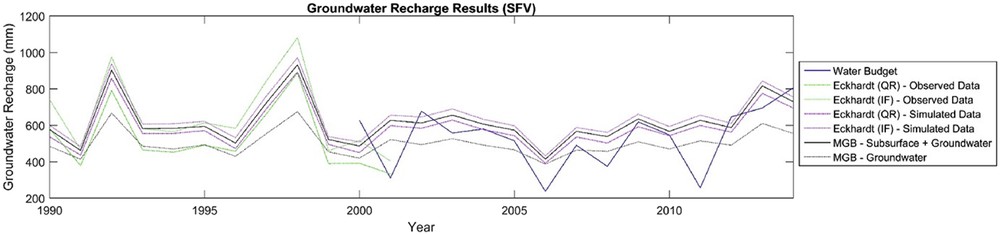
Annual groundwater recharge results for the SFV (1990–2014).
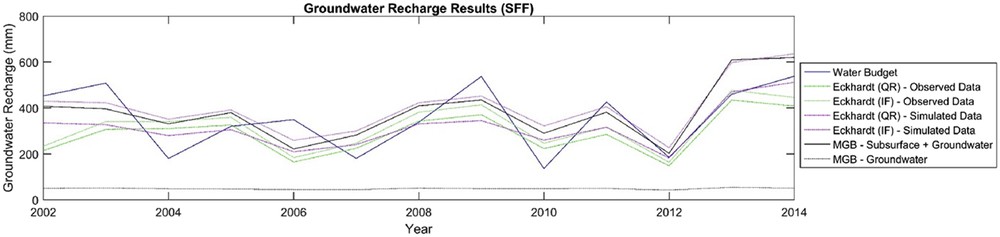
Annual groundwater recharge results for the SFF (2002–2014).
The SFV groundwater recharge results presented a variation between 26% and 33% in relation to the total annual precipitation, while the SFF presented a variation between 15% and 21%, with an exception for the MGB groundwater result with 3%. Generally, the different methods applied produced similar results. However, as groundwater recharge is quite difficult to quantify, the convergence of the results of some methods does not guarantee greater precision of the actual results. That being the case, this work suggests the average among all applied methods, 30% for the SFV and 16% for the SFF, as representative values of groundwater recharge.
4 Discussion
Each method applied revealed similar results in the calculation of groundwater recharge, where magnitude remained similar in most methods and their variations. The water balance method as it was designed, computed groundwater recharge as the sum of interflow and groundwater discharges. The results (SFV: 530 ± 170 mm and SFF: 352 ± 141 mm) were close to those obtained in the hydrograph separation using the QR method (516 ± 169 mm and SFF: 289 ± 90 mm) and the IF method (SFV: 642 ± 209 mm and SFF: 319 ± 99 mm) with the observed data. When compared to the MGB–IPH interflow and groundwater discharges, the results (SFV: 600 ± 161 mm and SFF: 373 ± 187) also coincided, indicating consistency between methods.
In relation to the MGB–IPH model and hydrograph separation results, different outcomes were verified for each watershed. The SFV displayed results close to hydrograph separation for the QR (516 ± 169 mm) and IF (642 ± 209 mm) methods on observed data when analyzing the MGB–IPH interflow and groundwater discharges (600 ± 161 mm), also only groundwater discharge (489 ± 95). The SFF watershed displayed results close to the hydrograph separation for the QR (289 ± 90 mm) and IF (319 ± 99 mm) methods with the observed data only when analyzing the MGB–IPH interflow and groundwater discharges (373 ± 187). Groundwater discharge alone (48 ± 6) differed substantially from the hydrograph separation results. Thus, according to the MGB–IPH model, the results indicate that the hydrograph separation approach to interflow and groundwater discharges together.
The lower groundwater reservoir discharges for the SFF could be explained by its high slopes, which affect the occurrence of groundwater recharge. Additionally, the occurrence of groundwater soil phreatic aquifer is quite common in SASG (Reginato and Strieder, 2006), which may contribute to high values of interflow, which is a more dynamic low-residence-time reservoir.
A common problem in groundwater recharge studies using hydrograph separation is the discontinuity of temporal data between discharge gauges. The results of this study showed that hydrological models may be a good alternative to work around this problem, as hydrograph separation with the observed data for the QR (SFV: 516 ± 169 mm and SFF: 289 ± 90 mm) and IF (SFV: 642 ± 209 mm and SFF: 319 ± 99 mm) methods are in the same magnitude as its simulated data for the QR (SFV: 583 ± 147 and SFF: 317 ± 93) and IF (SFV: 642 ± 157 and SFF: 401 ± 118) methods.
In evaluating the results of both the physical description (geomorphology, soil type, climate, land use, and hydrological characteristics) and groundwater recharge (water budget, hydrograph separation, and hydrological modelling) (Fig. 8), an understanding about interactions between aquifers, rivers and groundwater recharge can be achieved for the SFV and SFF. However, results are not spatially limited once they can be used to understand areas similar to SASG.

Physical description and groundwater recharge results.
The predominance in the SFV of lesser sloping hills with elongated and flattened tops, paired with deeper soils (latosols and nitosols) in agricultural areas favors water infiltration into the fractured aquifer due to the reduction of surface runoff. The occurrence of groundwater recharge in the SFV (between 26 and 33%) also favors the maintenance of higher baseflows in rivers, where a constant of recession of 79 days was found.
However, the SFF watershed presented hills with higher slopes where the tops are only elongated with shallower soils (nitosols), which are preferred areas for the occurrence of recharge, a configuration that hindered infiltration occurrence. The groundwater recharge occurrence in the SFF (between 3 and 21%) also encumbers the maintenance of baseflows in rivers, where a constant of recession of 29 days was found.
No research was found indicating groundwater recharge estimates in SFV and SFF basins for result comparison; however, Borges et al. (2017) presented groundwater recharge estimations in two nearby hydrographic basins located partially on the same aquifer system, the Iguaçu (70,800 km2) and Piquiri (24,171 km2) basins; the results obtained were 16.9% and 24.3% of the total annual precipitation, respectively; the authors also presented the average of 19.5% for the whole SASG. Yet, beyond the different basin areas, some physical characteristics (geology, topography, soil, and climate) display differences, even though the values are within the range obtained for the SFV and SFF basins.
Regarding the results usage beyond the two studied basins, the groundwater recharge estimates obtained serve as reference values for other hydrographic basins with the same physical and climate characteristics. Also, the results showed that the physical characteristics are relevant as regards the importance of interflow. Also, the differences obtained between the methods allow other authors to choose only one of them and still be aware of the uncertainties associated. For example, it is common in groundwater use planning studies to adopt only one of these techniques, mainly the most simplified. Moreover, the results showed that digital filters always tend to return groundwater recharges that add interflow with groundwater discharge; the uncertainties associated with the representativity of interflow demonstrated by hydrograph separation and shown in the model may indicate that the groundwater recharge estimate could be lower than those obtained considering hydrograph numerical filters, and this comparison is not much explored in the literature.
5 Conclusions
The purpose of this research was to present a comparison of the applications of different groundwater recharge estimation methods, the influence of physical characteristics on groundwater recharge, and to discuss the interaction between aquifers and rivers for large-scale subtropical basins. Topography and deep soils better explained the differences between the SFV and SFF watersheds. Thus, as the results confirmed it, groundwater recharge analysis requires physical analysis, especially in regard to larger areas. Spatial analysis should avoid overestimated results in sloped areas.
In general, the methods utilized were consistent; however, the results indicate that the hydrograph separation by numerical filters or water budget are related to the sum of interflow and groundwater discharges when compared to the MGB–IPH hydrological model. When considering only the hydrological model's groundwater discharge, the results were disparate by an 18% decrease for SFV and 87% for SFF.
The MGB–IPH model proved itself to be a useful tool for understanding the occurrence of annual groundwater recharge. In addition, the sub-basins' model discretization based on geomorphology was adequate to appropriately represent recharge studies.
The main uncertainty associated with this research is related to interflow representation. Only the MGB–IPH model represents this discharge, unlike hydrograph separation and water budget methods. Another important consideration is that since all methods use the same surface discharges as the input data, errors in measurements would affect all results.
For future researches, it is recommended to compare the MGB–IPH results with groundwater recharge methods that are not solely based on surface hydrological data. In addition, it is also necessary to evaluate the model's potentiality for non-subtropical basins with stream loss and evaluate possible modifications to represent these dynamics.
Acknowledgments
The authors would like to thank the Hydraulic Research Institute (IPH) of the Federal University of Rio Grande do Sul (UFRGS) and the Brazilian National Council for Scientific and Technological Development (CNPq) for funding this research.