1. Introduction
The Early Anthropogenic Hypothesis (EAH) considers that human activities related to early agriculture, herding and deforestation may have played a crucial role in driving the onset of global warming between 7 and 5 ka BP, as inferred from the increase of atmospheric CO2 and CH4 emissions recorded in high-latitude ice cores [Ruddiman 2003; Ruddiman et al. 2020]. However, the impact of human activities on landscapes, and how they affected the Earth Critical Zone [ECZ; Lin 2009] since prehistoric times, remains poorly documented. Recently, a number of geochemical proxies of past continental chemical weathering have emerged. These have great potential to provide key information on the ECZ dynamics. Among them, Li isotopes have been successfully applied to fine-grained sediment records of river-borne particles exported to deltas, alluvial terraces and lakes [Bastian et al. 2017; Rothacker et al. 2018; Yang et al. 2021]. A covariation of Li isotopic compositions with various climate proxies during the Quaternary period has demonstrated the rapid response of soil/rock weathering to major precipitation changes, in particular during arid/humid precessional cycles in the tropics and during glacial/interglacial cycles at higher latitudes [Bastian et al. 2019, 2017; Yang et al. 2021; Rothacker et al. 2018; Dosseto et al. 2015]. In soils, the lightest lithium isotope (6Li) is preferentially incorporated into secondary clay mineral phases as a result of mass-dependent isotope fractionation occurring during mineral/water interactions [Hindshaw et al. 2018; Vigier et al. 2008]. The δ7Li values of clay minerals can reflect the degree of continental weathering expressed as the ratio between dissolution rate of primary minerals versus clay neoformation rates [Bastian & Mologni et al., 2021, Pogge von Strandmann et al. 2017, 2020; Vigier et al. 2009]. In large continental-scale basins, where chemical and physical erosion operate under steady-state conditions, this ratio can be related to the intensity of chemical weathering, expressed as the ratio of silicate chemical weathering over total denudation [W/D ratio; Bouchez et al. 2013; Caves Rugenstein et al. 2019; Dellinger et al. 2017]. The provenance of fine-grained sediment in the Nile delta can be identified through its neodymium isotopic signature (εNd) [Bastian & Mologni et al., 2021, Blanchet 2019; Blanchet et al. 2014; Revel et al. 2015; Weldeab et al. 2014]. As no significant fractionation is observed during chemical weathering, εNd reflects the isotopic composition of the parent bedrock and the origin of the material. Since the Nile basin encompasses the Precambrian African basement as well as the Ethiopian and Somalian Cenozoic basalts, which have contrasting isotopic compositions, the Nd isotopic composition of sediment loads is suitable to distinguish their source regions [Bayon et al. 2015; Blanchet 2019; Grousset et al. 1988]. Our approach combines the use of neodymium and lithium isotope proxy records, together with measured sedimentation rates, in order to provide constraints on past hydrological conditions, sediment transport, and chemical weathering intensity in the Nile River watershed.
Major hydro-climate variability related to changes in insolation and monsoon dynamics were observed over tropical Africa during the Quaternary period, during which time ∼21 ka cycles of intense humid periods alternated with arid periods [Blanchet 2019; Blanchet et al. 2021; Kutzbach et al. 2020; McGee and deMenocal 2017; Ménot et al. 2020; Pausata et al. 2020]. This variability has been documented in several high-temporal resolution marine sediment records retrieved from the Nile Deep Sea Fan [NDSF; Bastian & Mologni et al., 2021, Bastian et al. 2017; Blanchet et al. 2015; Ménot et al. 2020; Mologni et al. 2020; Revel et al. 2015].
Between ∼3 and ∼1 ka BP, the Nile River Basin was home to Pre-Aksumite cultures followed by the Aksumite Kingdom, known to be one of the most important ancient East African civilizations [Anfray 1967; Fattovich 2010; Gerlach 2015; Japp 2019; Phillipson 2012]. Ancient Egyptian civilization was established further northward along the Nile mainstream and delta, and its emergence as early as 5000 cal. years BP has been associated with changes in the annual cycle of the Nile summer floods [e.g. Manning et al. 2017; Sheisha et al. 2022] suggesting a relationship between the end of the African Humid Period (AHP) and the Protodynastic period in Egypt.
The Aksumite kingdom was mostly located in the Ethiopian highlands, an area almost exclusively composed of Cenozoic basaltic rocks (Ethiopian Traps), Mesozoic sediments underlain by the Pan-African basement (Figure 1b). The Ethiopian Traps are characterized by a radiogenic neodymium (Nd) isotopic signature with an εNd ranging between ∼0–∼7 [Garzanti et al. 2015], which differs largely from the εNd composition of the detrital sediment derived from other branches of the Nile catchment (Figure 1a). As a consequence, the Nd isotopic composition of the fine-grained sediment deposited at the NDSF can be used to identify the contribution of river-borne particles transported by the Blue Nile and the Atbara/Tekeze headwaters. These drain the Ethiopian Highlands which were occupied by the territories of the Pre-Aksumites and Aksumite Kingdom, as well as the White Nile branch (draining the Central African Craton), and those derived from aeolian inputs from the nearby Saharan Metacraton [Mologni et al. 2020; Bastian et al. 2017; Blanchet et al. 2013; Revel et al. 2015]. Previous studies on the combined use of lithium and neodymium isotopes in clay-size fractions of sediment deposited in the NDSF indicated a close relationship between hydrological changes, continental chemical weathering and the delivery of material derived from the Ethiopian Traps over the past 100 to 1 ka [Bastian & Mologni et al., 2021]. In this study, we reconstruct the variability of sediment provenance and chemical weathering in the Nile Basin using a marine sediment record covering the last 9000 years. This record cover to the period associated with the rise of the first East African complex polities, namely the Pre-Aksumite cultures (∼3 to ∼2 ka BP) and the Aksumite Kingdom (∼2 to ∼1 ka BP) that proceeded it [Fattovich 2010; Phillipson 2012], which are contemporaneous with the Ancient Egyptian Dynastic, Ptolemaic and Roman civilizations [Manning et al. 2017]. In addition to discussing original data on marine sediments, we use a wealth of information provided by a number of Holocene paleo-pedological [Bard et al. 2000; Gebru et al. 2009; Terwilliger et al. 2013, 2011], archaeological and paleoclimatic [Costa et al. 2014; Jaeschke et al. 2020] investigations conducted in the Ethiopian Highlands to discuss potential impacts of intensified land-use by Aksumite and Late Egyptian complex polities on sediment transport and chemical weathering intensity.
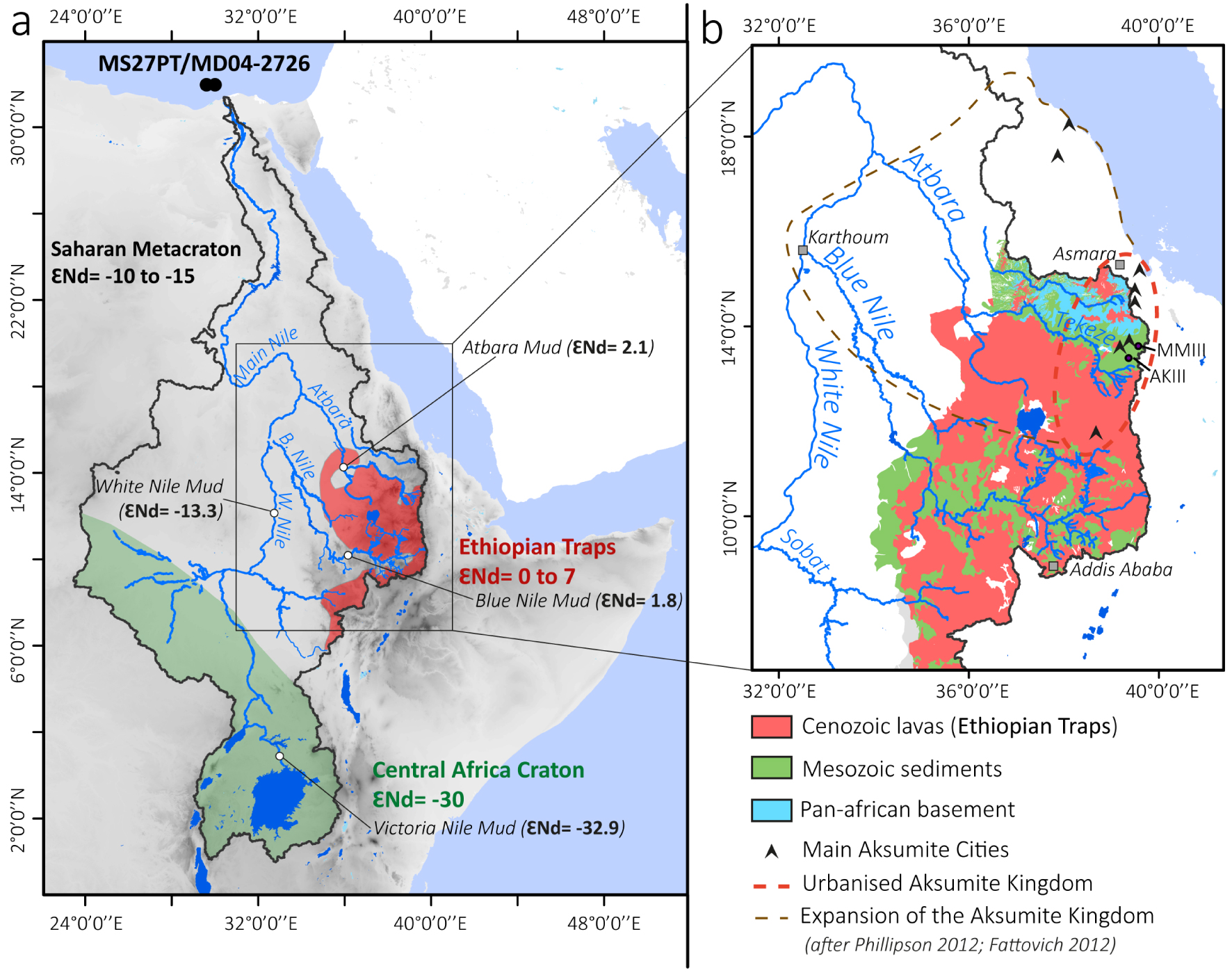
(a) Map of the Nile River basin and location of cores MS27PT (N31° 47.90′; E29° 27.70′, 1389 m water depth) and MD04-2726 (31° 51.000′ N, 29° 47.490′ E, 1058 m water depth) in the NDSF. Three main sources of sediment are identified in the Nile basin: the basaltic rocks (red) of the Ethiopian traps (Highlands), which are drained by the Blue Nile, the Atbara/Tekeze and Sobat rivers located in tropical latitudes (around 5 to 15° N); the Precambrian metamorphic rocks (green) of the Central African Craton located in the Ugandan headwaters region of the White Nile and drained by the Bahr el Jebel River; and the Saharan Metacraton sources [Abdelsalam et al. 2002; Grousset and Biscaye 2005; Scheuvens et al. 2013]. εNd values for the Victoria, White and Blue Nile River mud samples are from Garzanti et al. [2015]. (b) Map of the Blue Nile and Atbara River basins with the location of the main Aksumite cities [black triangles, after Fattovich 2010; Phillipson 2012], modern towns (grey squares), the limits of the Nile river catchment (black line), the urbanized Aksumite territories (red dotted line), the expansion of the Aksumite kingdom [brown dotted line; after Fattovich 2010; Phillipson 2012], Mai Maikden (MMIII, brown circle) and Adi Kolen (AKIII, green circle) soil sequence studies presented in Figure 3 [Gebru et al. 2009; Terwilliger et al. 2011, 2013]. Masquer
(a) Map of the Nile River basin and location of cores MS27PT (N31° 47.90′; E29° 27.70′, 1389 m water depth) and MD04-2726 (31° 51.000′ N, 29° 47.490′ E, 1058 m water depth) in the NDSF. Three main sources of sediment are identified in the Nile basin: the basaltic rocks ... Lire la suite
2. Materials and methods
The Nile Delta deep sea cores we sampled display irregular shoreline protuberances directly under the influence of the Nile River’s hydro-sedimentary activity [Mologni et al. 2020]. A substantial fraction of the fine-grained particulate load delivered from the Nile River is stored on the Egyptian continental slope in the form of mixed deposits of fine-grained turbidites and hemipelagic sediments. In this study, we investigate two well-dated sediment sequences: core MS27PT [N31° 47.90′; E29° 27.70′; 1389 m water depth; Bastian & Mologni et al., 2021] and core MD04-2726 [N31° 51.00′; E29°47.49′; 1058 m water depth; Mologni et al. 2020], which provide a continuous record of clastic particles exported from the Nile river basin over the last ∼100 ka and 11 ka, respectively. The age models of these sediment records are based on 59 previously published radiocarbon dates [Bastian & Mologni et al., 2021, Mologni et al. 2020; Revel et al. 2015, 2010]. In this study, we present new results obtained from the upper 2 m of MD04-2726, focusing on the finest (<2 μm) clay-size detrital fraction. Because the clay-size fractions deposited near the mouth of rivers include secondary clay minerals initially formed in soil weathering profiles, they are thus well suited for the investigation of past silicate chemical weathering in river catchments. Additionally, due to their small size and density, clays are expected to be efficiently delivered from source to sink [Bastian et al. 2017].
2.1. Lithium isotope analyses
Chemical purification of Li was performed at the LOV (Laboratoire Océanographique de Villefranche-sur-Mer) in a clean laboratory. A solution containing ∼60 ng of lithium was introduced on a cationic resin column (AG50X12) and Li was eluted using titrated ultrapure 1.0 N HCl [Vigier et al. 2008]. This separation was performed twice to ensure perfect Li–Na separation. LiCl solution was then evaporated to dryness and re-dissolved in 0.05 N HNO3 for isotope analyses. Lithium isotope analyses (δ7Li = ((7Li∕6Li)∕(7Li∕6Li)LSVEC − 1) × 1000, LSVEC being the international standard) were performed at the Ecole Normale Supérieure de Lyon (CNRS-INSU National Facilities) using a Neptune Plus (Thermo-Fisher) multi-collector inductively coupled plasma spectrometer (MC-ICP-MS) along with a sample-standard bracketing technique. A combination of Jet and X cones were used, as well as an Aridus II desolvating system, resulting in a sensitivity of 1 V 7Li∕ppb [Balter and Vigier 2014; Thibon et al. 2021]. Before analyses, Li fractions were diluted to match 4 ppb Li. Total procedural blanks were negligible (<10 pg Li), representing ∼0.02% maximum of the total Li fraction for each sample. The accuracy of isotopic measurements was assessed several times during each measurement session using reference Li7–N solution [Carignan et al. 2007] and other reference materials [BE-N and JB-2 rocks and seawater, see Verney-Carron et al. 2015].
2.2. Neodymium isotope analyses
Neodymium isotopic ratios were measured at the Pôle Spectrométrie Océan (Brest, France). For convenience, Nd isotopic ratios results are expressed as: εNd = [[143Nd∕144Nd(meas.)∕143Nd∕144Nd(CHUR)] − 1] × 104. The CHUR (Chondritic Uniform Reservoir) value is 0.512638 [Jacobsen and Wasserburg 1980]. Neodymium was purified using conventional ion chromatography [Bayon et al. 2012]. Nd isotopic compositions were determined using sample-standard bracketing by analysing JNdi-1 standard solutions every two samples. Mass bias corrections were made using the exponential law considering 146Nd∕144Nd = 0.7219. Mass-bias corrected values for 143Nd∕144Nd were normalized to a JNdi-1 value of 143Nd∕144Nd = 0.512115 [Tanaka et al. 2000]. Repeated analyses of bracketed JNdi-1 standard solutions during the course of this study yielded 143Nd∕144Nd of 0.512117 ± 0.000012 (2 SD, n = 38), corresponding to an external reproducibility of ∼±0.23ε (2 SD).
3. Results: Li and Nd isotopic compositions of clay fractions
Bastian & Mologni et al. [2021] show how the Nd isotopic composition of clay-size fractions from core MS27PT varies from εNd < –3 during humid periods to −8 during arid periods, following the variability of insolation between precession maxima and minima periods between 100 and 3 cal. ka BP (Figure 2).
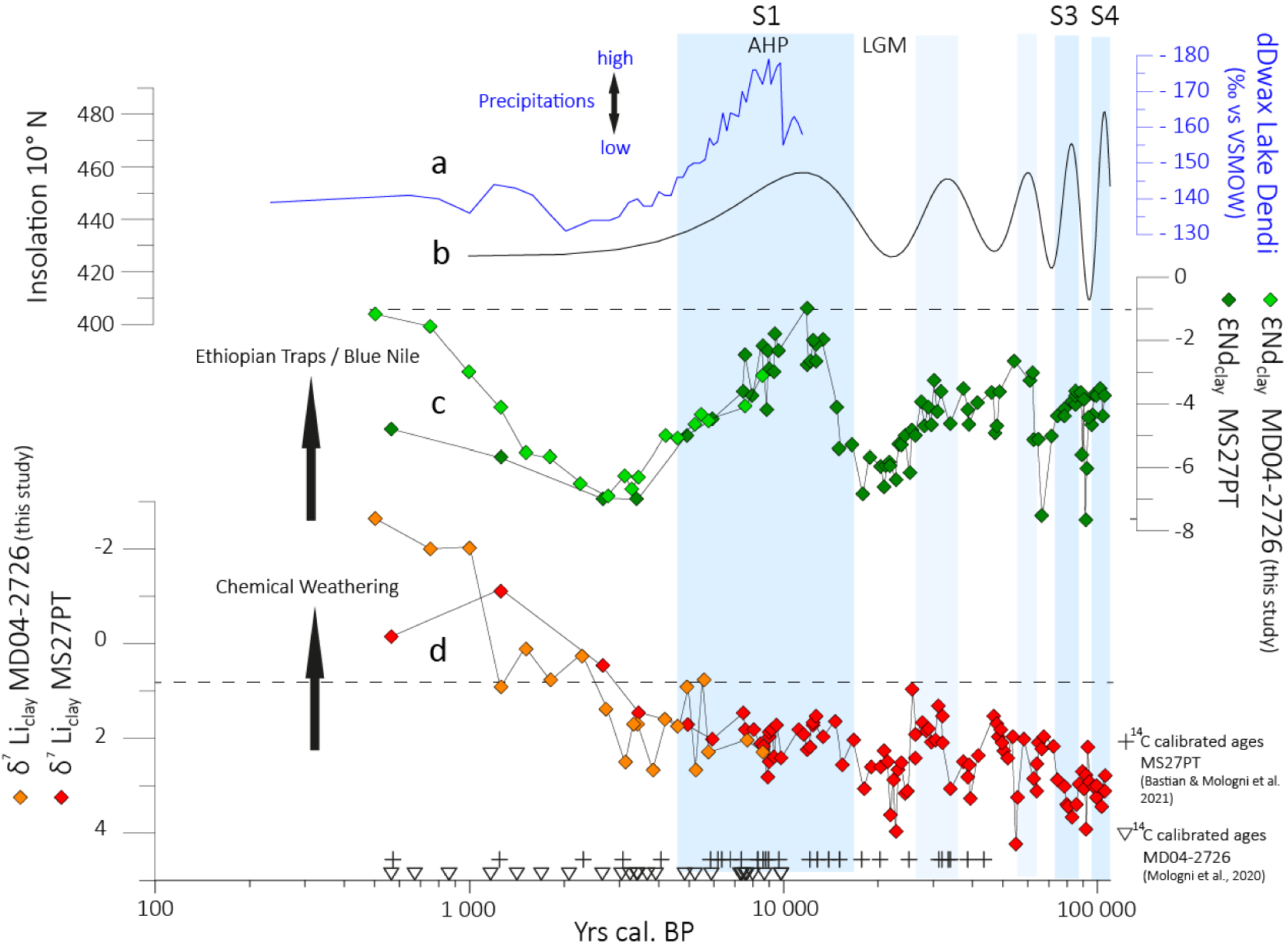
A selection of paleoenvironmental proxy records for East Africa. (a) δDwax of Lake Dendi [Jaeschke et al. 2020]; (b) July insolation in watt∕m2 at 10° N [Berger and Loutre 1991]; (c) εNd values of clay-size fractions of cores MS27PT (dark green points) and MD04-2726 (light green points) cores; (d) δ7Li values of clay-size fractions of cores MS27PT (red points) and MD04-2726 (orange points) cores. Black crosses and reversed triangles indicate 14C calibrated ages of MS27PT and MD04-2726 cores respectively. S1, S3 and S4 refer to Sapropel 1, 3 and 4 respectively; AHP refers to “African Humid Period” and LGM to “Last Glacial Maximum” period. Masquer
A selection of paleoenvironmental proxy records for East Africa. (a) δDwax of Lake Dendi [Jaeschke et al. 2020]; (b) July insolation in watt∕m2 at 10° N [Berger and Loutre 1991]; (c) εNd values of clay-size fractions of cores MS27PT (dark green points) and ... Lire la suite
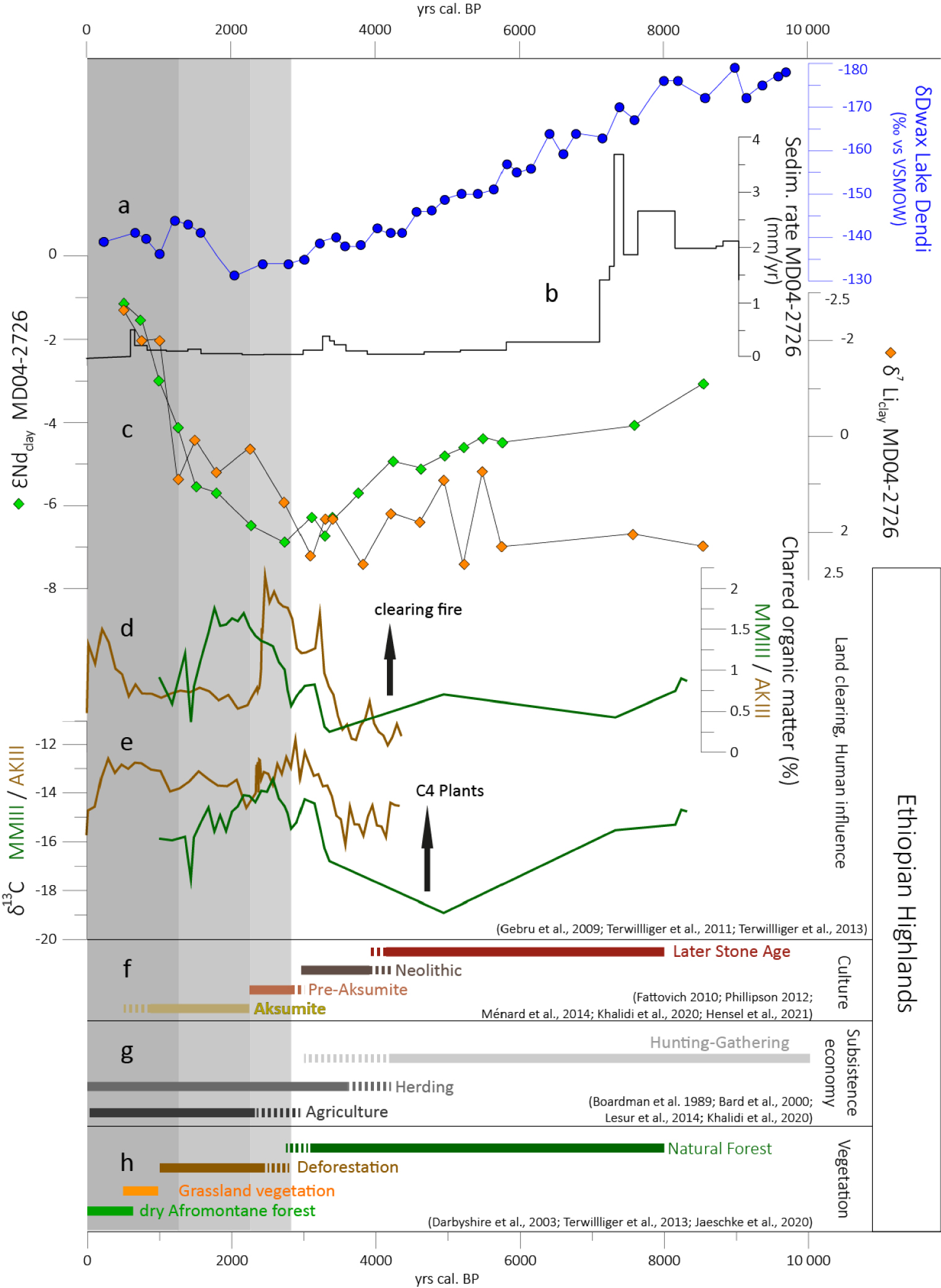
A diagram illustrating paleoenvironmental proxies in association with an archaeological timeline for East Africa during the Holocene. (a) δDwax of Lake Dendi [Jaeschke et al. 2020]; (b) sedimentation rate of core MD04-2726; (c) εNd (light green) and δ7Li (orange) in clay-size fractions of core MD04-2726; (d) charred organic matter (%) of Mai Maikden (MMIII, green line) and Adi Kolen (AKIII, brown line) soil profiles [Gebru et al. 2009; Terwilliger et al. 2011, 2013]; (e) δ13C of Mai Maikden (MMIII, green line) and Adi Kolen (AKIII, brown line) soil profiles [Gebru et al. 2009; Terwilliger et al. 2011, 2013]. Changes in: (f) Human culture [after Fattovich 2010; Hensel et al. 2019; Khalidi et al. 2020; Ménard et al. 2014; Phillipson 2012], (g) Subsistence economy [after Bard et al. 2000; Boardman 1999; Khalidi et al. 2020; Lesur et al. 2014], (h) Vegetation [after Darbyshire et al. 2003; Terwilliger et al. 2013; Jaeschke et al. 2020] across the Ethiopian highlands. Masquer
A diagram illustrating paleoenvironmental proxies in association with an archaeological timeline for East Africa during the Holocene. (a) δDwax of Lake Dendi [Jaeschke et al. 2020]; (b) sedimentation rate of core MD04-2726; (c) εNd (light green) and δ7Li (orange) in clay-size fractions of ... Lire la suite
Our new results show that the εNd values of clays separated from core MD04-2726 vary between −2.3 to −6 at the end of the AHP (Table 1). This range of εNd values is similar to one observed in the core MS27PT over the last 100 ka insolation cycles. Surprisingly, after 2.3 ka, the Nd isotopic composition of clay-size fractions from both cores increases gradually but significantly, to reach a maximum radiogenic εNd value of −1 around 400 years ago.
δ7Liclay and εNdclay data for clays from the MD04-2726 core
ID Clay sample | Depth (cm) | Age (years cal. BP) | δ7Li (‰) | εNd | 2σ |
---|---|---|---|---|---|
C5 | 1 | 501 | −2.64 | −1.14 | 0.29 |
C7 | 5 | 751 | −2 | −1.53 | 0.25 |
C14 | 9 | 1000 | −2.04 | −3.03 | 0.25 |
C3 | 13 | 1248 | 0.93 | −4.11 | 0.19 |
C6 | 17 | 1497 | 0.076 | −5.53 | 0.26 |
C9 | 21 | 1801 | 0.78 | −5.7 | 0.3 |
C13 | 25 | 2269 | 0.21 | −6.48 | 0.22 |
C15 | 29 | 2737 | 1.39 | −6.89 | 0.24 |
C11 | 33 | 3115 | 2.525 | −6.25 | 0.26 |
C2 | 37 | 3290 | 1.74 | −6.69 | 0.23 |
C4 | 41 | 3409 | 1.69 | −6.33 | 0.25 |
C1 | 45 | 3810 | 2.67 | −5.63 | 0.37 |
C18 | 49 | 4212 | 1.59 | −4.93 | 0.23 |
C16 | 53 | 4613 | 1.79 | −5.09 | 0.24 |
C8 | 57 | 4948 | 0.92 | −4.8 | 0.21 |
C10 | 61 | 5215 | 2.67 | −4.61 | 0.26 |
C17 | 65 | 5482 | 0.74 | −4.35 | 0.23 |
C12 | 69 | 5750 | 2.3 | −4.48 | 0.26 |
C20 | 128 | 7564 | 2.03 | −4.09 | 0.19 |
C19 | 186 | 8543 | 2.29 | −3.06 | 0.26 |
The δ7Li composition of clays from core MS27PT varies between 4‰ and 1‰ during the last 100 ka (Table 2), following the alternation between insolation minima and maxima periods. As for Nd isotopes, after 3 ka, clay δ7Li reached values that are unprecedented in the last 100 ka, and it decreased gradually, to reach a value of −2.5‰ 400 years ago.
δ7Liclay and εNdclay data measured in the clay fractions from the MS27PT core
ID Clay sample | Depth (cm) | Age (years cal. BP) | δ7Li (‰) | 2σ | εNd | 2σ |
---|---|---|---|---|---|---|
A28 | 2 | 1258 | −1.15 | 0.01 | −5.69 | 0.1 |
A24 | 7 | 2640 | 0.47 | 0.06 | −6.97 | 0.14 |
A33 | 11 | 3449 | 1.45 | 0.01 | −7 | 0.2 |
A30 | 17 | 4923 | 1.72 | 0.01 | −5.01 | 0.12 |
A2 | 22 | 5925 | 2.02 | 0.06 | −4.51 | 0.16 |
A22 | 35 | 7430 | 1.47 | 0.02 | −3.64 | 0.13 |
A46 | 39 | 7582 | 1.85 | 0.02 | −2.45 | 0.14 |
A34 | 52 | 7946 | 1.82 | 0.02 | −3.62 | 0.17 |
A29 | 54 | 8007 | 1.8 | 0.01 | −3.81 | 0.12 |
L21 | 70 | 8403 | 2.11 | 0.06 | ||
A48 | 82 | 8551 | 2.14 | 0.01 | −2.1 | 0.13 |
L22 | 95 | 8712 | 2.47 | 0.04 | ||
A3 | 102 | 8891 | 2.83 | 0.02 | −4.2 | 0.19 |
A39 | 111 | 8980 | 1.95 | |||
A35 | 114 | 8999 | 2.49 | 0.01 | −2.27 | 0.12 |
L23 | 120 | 9068 | 1.88 | 0.08 | ||
A57 | 137 | 9203 | 1.79 | 0.01 | −2.98 | 0.17 |
A5 | 152 | 9338 | 2.41 | 0.02 | −3.01 | 0.23 |
A47 | 172 | 9498 | 1.72 | 0.01 | −1.73 | 0.17 |
A58 | 194 | 9683 | 2.04 | 0.01 | −2.34 | 0.27 |
L24 | 206 | 9820 | 2.43 | 0.06 | ||
L26 | 243 | 11,165 | 1.81 | 0.05 | ||
L27 | 255 | 11,601 | 1.89 | 0.06 | ||
A6 | 263 | 11,892 | 2.27 | 0.02 | −0.98 | 0.17 |
A31 | 269 | 12,074 | 2.15 | 0.01 | −2.82 | 0.2 |
A44 | 274 | 12,256 | 1.73 | 0.01 | −2.65 | 0.23 |
A27 | 278 | 12,522 | 1.66 | 0.02 | −1.99 | 0.12 |
A25 | 281 | 12,695 | 1.53 | 0.02 | −2.76 | 0.11 |
A7 | 283 | 12,810 | 1.79 | 0.02 | −2.11 | 0.15 |
A54 | 289 | 13,309 | 1.99 | 0.02 | −1.97 | 0.2 |
A56 | 296 | 14,588 | 1.62 | 0.02 | −3.65 | 0.14 |
296 | 14,934 | 1.98 | 0.04 | −4.24 | 0.12 | |
A8 | 298 | 15,280 | 2.58 | 0.02 | −5.44 | 0.17 |
A45 | 300 | 16,635 | 2.03 | 0.02 | −5.25 | 0.16 |
A9 | 300 | 17,989 | 3.08 | 0.02 | −6.88 | 0.14 |
A55 | 301 | 18,855 | 2.59 | 0.01 | −5.63 | 0.22 |
A32 | 303 | 20,588 | 2.61 | 0.02 | −5.97 | 0.15 |
B6 | 304 | 21,024 | 2.22 | 0.03 | −6.63 | 0.31 |
B4 | 305 | 21,461 | 2.52 | 0.04 | −6.01 | 0.14 |
A10 | 306 | 21,897 | 3.63 | 0.01 | −5.82 | 0.16 |
B5 | 307 | 22,334 | 2.88 | 0.04 | −5.99 | 0.14 |
B8 | 308 | 22,770 | 4 | 0.03 | −6.4 | 0.23 |
A37 | 310 | 23,207 | 2.65 | 0.01 | −5.66 | 0.18 |
B9 | 310 | 23,643 | 2.51 | 0.03 | −5.23 | 0.12 |
B11 | 311 | 24,079 | 2.87 | 0.03 | −5.25 | 0.12 |
B10 | 312 | 24,516 | 3.16 | 0.04 | −4.95 | 0.08 |
B12 | 313 | 24,952 | 3.12 | 0.04 | −5.7 | 0.14 |
B14 | 314 | 25,389 | 1.77 | 0.08 | −6.18 | 0.16 |
A36 | 316 | 25,825 | 0.93 | 0.01 | −4.83 | 0.18 |
A60 | 317 | 26,411 | 1.92 | 0.01 | −5.05 | 0.16 |
B3 | 321 | 27,630 | 1.65 | 0.04 | −3.9 | 0.16 |
B7 | 323 | 28,312 | 1.86 | 0.04 | −4.74 | 0.27 |
A61 | 325 | 28,994 | 1.74 | 0.01 | −4.08 | 0.33 |
B2 | 327 | 29,677 | 2.09 | 0.03 | −4.68 | 0.45 |
B1 | 329 | 30,359 | 2.02 | 0.02 | −3.26 | 0.12 |
A59 | 331 | 31,041 | 1.3 | 0.01 | −4.29 | 0.18 |
D3 | 335 | 31,881 | 1.53 | 0.04 | −3.64 | 0.25 |
D10 | 343 | 32,107 | 2.12 | 0.04 | −3.75 | 0.22 |
D12 | 357 | 34,078 | 3.09 | 0.02 | −4.67 | 0.25 |
E9 | 370 | 37,689 | 2.48 | 0.04 | −3.55 | 0.22 |
E3 | 374 | 38,841 | 2.83 | 0.03 | −4.09 | 0.21 |
E19 | 375 | 39,024 | 2.56 | 0.04 | −4.56 | 0.23 |
E15 | 376 | 39,101 | 2.82 | 0.03 | −4.15 | 0.25 |
E4 | 377 | 39,183 | 3.31 | 0.02 | −4.65 | 0.23 |
D8 | 385 | 41,627 | 2.34 | 0.03 | −4.01 | 0.25 |
E12 | 395 | 46,755 | 1.52 | 0.03 | −3.6 | 0.32 |
E14 | 397 | 47,811 | 1.72 | 0.02 | −4.98 | 0.22 |
E2 | 398 | 48,339 | 2 | 0.03 | −4.66 | 0.22 |
F6 | 399 | 48,630 | 1.98 | |||
E8 | 400 | 49,081 | 1.81 | 0.01 | −3.62 | 0.22 |
F4 | 403 | 50,015 | 2.27 | |||
F3 | 408 | 51,572 | 2.44 | |||
F2 | 414 | 53,440 | 1.95 | |||
E18 | 419 | 54,997 | 4.27 | 0.06 | −2.66 | 0.24 |
F5 | 421 | 55,619 | 3.34 | |||
F1 | 429 | 58,110 | 1.98 | |||
D7 | 440 | 61,535 | 0.03 | 2.7 | −3.29 | 0.24 |
D9 | 443 | 62,469 | 2.87 | 0.02 | −3.03 | 0.25 |
D2 | 447 | 63,715 | 2.52 | 0.04 | −5.18 | 0.26 |
E1 | 448 | 64,026 | 3.09 | 0.02 | −5.15 | 0.24 |
E11 | 451 | 64,960 | 2.08 | 0.02 | −5.09 | 0.23 |
D4 | 455 | 66,206 | 2.21 | 0.03 | −6.66 | 0.23 |
D11 | 459 | 67,275 | 1.92 | 0.04 | −7.55 | 0.25 |
D1 | 469 | 72,290 | 2.17 | 0.03 | −4.99 | 0.25 |
D5 | 476 | 74,500 | 2.9 | 0.03 | −4.4 | 0.27 |
E7 | 495 | 78,500 | 3.01 | 0.02 | −4.17 | 0.24 |
E5 | 506 | 79,600 | 3.38 | 0.03 | −4.41 | 0.25 |
D6 | 520 | 81,000 | 3.45 | 0.03 | −4.09 | 0.24 |
D20 | 540 | 83,000 | 3.67 | 0.05 | −3.9 | 0.23 |
D15 | 553 | 84,300 | 3.52 | 0.03 | −4.1 | 0.25 |
E13 | 567 | 85,700 | 3.41 | 0.02 | −3.71 | 0.23 |
E17 | 578 | 87,320 | 2.96 | 0.02 | −3.58 | 0.23 |
D19 | 590 | 89,374 | 2.71 | 0.03 | −3.59 | 0.24 |
D16 | 597 | 90,455 | 3.09 | 0.03 | −5.66 | 0.23 |
D13 | 602 | 91,201 | 2.67 | 0.04 | −3.85 | 0.26 |
D18 | 609 | 92,080 | 3.99 | 0.03 | −7.69 | 0.23 |
D22 | 617 | 93,085 | 2.14 | 0.04 | −6.03 | 0.26 |
E16 | 627 | 94,341 | 2.95 | 0.02 | −4.44 | 0.26 |
D17 | 641 | 96,100 | 3.03 | 0.03 | −4.65 | 0.26 |
E22 | 645 | 96,603 | 3.07 | 0.02 | −4.27 | 0.2 |
E6 | 666 | 99,304 | 3.28 | 0.02 | −3.71 | 0.22 |
D14 | 679 | 100,874 | 2.99 | 0.01 | −3.8 | 0.24 |
E10 | 694 | 102,758 | 3.45 | 0.02 | −3.47 | 0.25 |
D23 | 705 | 104,140 | 2.99 | 0.02 | −3.97 | 0.2 |
D25 | 712 | 105,020 | 2.76 | 0.02 | −4.42 | 0.25 |
D24 | 719 | 105,899 | 3.15 | 0.03 | −3.74 | 0.23 |
4. Discussion
4.1. Evidence for non-climatic forcing on chemical weathering since 3000 years cal. BP
A striking result of our study is that since 3000 cal. years BP, the Li isotopic composition of clay-size fractions has decreased to negative values, differing substantially from the observed δ7Li trend observed over the last 100 cal. ka BP [Figure 2; Bastian & Mologni et al., 2021]. This observation implies (1) that a new forcing gradually appeared around ∼3000 cal. BP, resulting in an increase in the intensity of chemical weathering, and (2) that this forcing dominated other controlling factors that were at play before, namely those related to insolation-driven hydro-climate variability and ocean dynamics [see e.g. Bastian & Mologni et al., 2021].
Estuarine and deltaic processes may contribute to an in situ weathering of primary minerals deposited in the NDSF [Zhang et al. 2022a, b]. However, it has been demonstrated by Bastian et al. [2017] that variations in δ7Li recorded in the NDSF clay fraction cannot be explained by diagenetic processes. Early clay diagenesis and seawater isotopic fractionation is normally characterised by higher δ7Li than continental weathering products [Wei et al. 2020], which can even be associated with Li absorption from seawater [δ7Li = 31.2; Misra and Froelich 2012]. More negative δ7Li recorded at the top of the core allow us to exclude this effect for the NDSF. Indeed, small quantity of organics in sediments could favour the stabilization of deposited primary phases [Krissansen-Totton and Catling 2017; Michalopoulos and Aller 2004].
The provenance of clays transported by the Nile River can be inferred from Nd isotopes. The clay-size fractions deposited during the last 3000 years at sites MD04-2726 and MS27PT display a trend towards markedly increasing εNd values similar to the highly radiogenic values typical of the Ethiopian Traps that are generally encountered during past African Humid Periods. The same trend towards more radiogenic values (up to εNd ∼ 0.4) is also recorded for the last 1880-year interval of core P362/2-33, a nearby marine sediment core retrieved from the NDSF Rosetta system [Blanchet et al. 2015, 2013]. Over the last 100 ka, the time intervals corresponding to the deposition of clays with radiogenic (high) εNd signatures were systematically associated with an increase in sedimentation rates (up to ∼100 cm/ka), best explained by higher physical erosion rates in the Ethiopian Traps area [Bastian & Mologni et al., 2021, Revel et al. 2010]. In contrast, since 3000 years cal. BP, the observed increase in εNd values has been associated with relatively low sedimentation rates [>2 cm/ka; Revel et al. 2015] and low terrigenous fluxes [<4 g∕cm2∕ka; Revel et al. 2014], in agreement with lower insolation and precipitation patterns (Figure 2). As previously shown in Bastian & Mologni et al. [2021] and Mologni et al. [2020], this may result from low fluvial transport of fine-grained sediments still active during arid climates, since more that 80% of the sediment deposited at the NDSF originates in the Ethiopian Highlands [Garzanti et al. 2015]. This can be explained by Blue Nile seasonal discharge, supported by summer monsoon precipitations even during arid periods [Harrower et al. 2020; Sulas 2014].
Overall, the radiogenic εNd signature of clay-size fractions in cores MD04-2726 and MS27PT indicate a Blue Nile/Ethiopian Traps provenance over the last ∼3000 years. A reworking of Ethiopian clay can be excluded because it would result in a shift toward lower, rather than higher εNd values. Previously deposited sediments with high radiogenic εNd values could have been reworked by the intensive irrigation management conducted along the Nile mainstream during the Ptolemaic or Roman periods, concomitant with the development of the Aksumite civilization [Flaux et al. 2012; Pennington et al. 2017; Stanley and Bernhardt 2010]. However, in that case, one would have expected a shift towards lower εNd values. Indeed, basaltic Nd values (εNd = −2 to ∼0) of Ethiopian Traps origin would have been diluted by the input of sediments with the lowest εNd values originating from the White Nile River (εNd = −13.3) and from aeolian sources, which are not preserved [εNd = −15; from the Saharan Metacraton; Abdelsalam et al. 2002; Garzanti et al. 2015].
Altogether, the low δ7Li and high εNd signatures of the Late Holocene support a strong non-climatic forcing of chemical weathering conditions in the Ethiopian Traps. These results are at odds with what would normally be expected based on the arid hydro-climatic conditions in East Africa during this period and based on chemical weathering trends observed over the last 100 ka. In consequence, we suggest that anthropogenic control was a probable driving force of chemical weathering in the Ethiopian Highlands.
4.1.1. Concomitant increase of land use and chemical weathering since 3000 BP in the Ethiopian highlands
East Africa is widely known as one of the cradles of humankind because the volcano-tectonic formation of the East African Rift System created the ideal living conditions for early hominins and for the preservation of their remains [WoldeGabriel et al. 2001]. Comprehensive archaeological studies have demonstrated that during the long period of transition from solely hunter-gathering, to herding around 12 to 3 ka cal. BP, populations were relatively well adapted to the effects of hydrological changes in the region as a result of flexible mobility and subsistence strategies [Chritz et al. 2019; Khalidi et al. 2020; Kuper and Kröpelin 2006; Mologni et al. 2021; Wright et al. 2015]. The Late Holocene witnessed a gradual aridification during which the first East African complex polities in Highland Ethiopia and Eritrea developed and integrated agriculture into their socio-economic fabric [Boardman 1999]. The Ethiopian plateau, located in the headwaters region of the Blue Nile, saw the emergence of the first Pre-Aksumite polities around 3000 BP, followed by the foundation of the first Aksumite urban settlements around ∼2000 years cal. BP [Fattovich 2010; Phillipson 2012]. Extending over the source region of the Blue Nile and Atbara/Tezeke rivers, the pre-Axumite to Axumite period was accompanied by the intensification of agricultural practices [Blond et al. 2018; Figure 1b; Boardman 1999]. Even if the use of irrigation systems was not necessary because of abundant seasonal monsoonal rainfall [Harrower et al. 2020], anthropic small-scale management of primary landscapes became widespread across the highland region [Blond et al. 2018; Sulas 2014]. From ∼2600 BP onwards, increasing demographic pressure over the Ethiopian highlands was accompanied by intensified land use and deforestation [Darbyshire et al. 2003], soil degradation [Bard et al. 2000] and water management [Blond et al. 2018; Federica and Innocent 2018; Sulas 2014; Sulas et al. 2009]. Organic proxy investigations of soil profiles from the headwaters region of the Atbara/Tekeze rivers (Adi Kolan=AKIII, Mai Maikden=MMIII; Figure 1b) revealed a strong increase in land clearing and anthropogenic fire activity between ∼3000 and ∼2000 years cal. BP [Gebru et al. 2009; Terwilliger et al. 2013, 2011; Figure 3c, d]. Other proxies such as hydrogen (HI) and oxygen (OI) indices from Rock-Eval pyrolysis also indicated intense chemical alteration of organic matter due to agricultural humidification processes and/or human-induced fires [Terwilliger et al. 2013, 2011]. Such extensive anthropogenic landscape management had a major impact on the pedogenic system, leading to an increase of soil leaching, and potentially explaining the decrease of δ7Li values during this period.
In this context, the δ7Li proxy evidence for enhanced chemical weathering in the Ethiopian Traps starting ∼3000 BP is concomitant with anthropogenic landscape degradation recorded during the Pre-Aksumite and Aksumite periods (Figure 3). During the same period, large erosion rates in the Ethiopian highlands were recorded in Lake Dendi sediment records, in agreement with intensive land-use activity [Jaeschke et al. 2020].
Overall, the concomitance between the intensification of land use beginning around 3000 BP and enhanced soil alteration (inferred from negative δ7Li values) suggests that agricultural management systems played a key role in this dramatic shift. The impact of human activities on Li isotopes appears clearly distinct from the effect induced by an increase in precipitation during high insolation periods. It should be specified that the area of the Pre-Aksumite and Aksumite polities to which our results refer are those drained by tributaries of the Nile River, which cover only one part of the overall territory of these polities (Figure 1b). Consequently, our results reflect the impact of anthropic activities in regions that were part of the territory of the Aksumite kingdom and its precursors, and not necessarily that of the main centres [Fattovich 2010; Phillipson 2012].
It is interesting to note that paleolimnological and historical data obtained for the Late Holocene record of Lake Fayum along the northern Nile indicate an increase of land-use since ∼4 ka BP, hence 1000 years earlier than the observed εNd shift in our NDSF cores [Butzer 1997; Hamdan et al. 2020; Hassan et al. 2006]. Similarly, various indices of land-use intensification in the Nile delta have been recorded since ∼4 ka BP [Zhang et al. 2022a, b]. The fact that there was a recorded increase of human activities in the Nile delta and no change in εNd signature around 4 Ka BP indicates that the reworking of fine-grained sediments with radiogenic Nd isotopic signatures and erosion processes were negligible during this period. In contrast, the co-evolution of chemical weathering (inferred from δ7Li) and intensive land-use patterns associated with pre-Aksumite and Aksumite complex polities (inferred from εNd and sedimentological evidence) over the last 3000 years suggest that the increase of chemical weathering intensity was mostly generated in the Blue Nile basin, and therefore in the basaltic highlands. Further high resolution studies of local pedo-sedimentary archives will be needed in order to more precisely quantify the effects of land use on weathering in this region, and which may be more significant than what has been illustrated by studies of deltaic sediments.
4.2. Towards a global scale impact of anthropogenic activities on Earth systems since the late Holocene?
As discussed above, the combined Nd and Li isotope records are consistent with an increase of chemical weathering intensity in the Ethiopian highlands (Figure 4a). This is during a period characterized by deforestation, the development of agriculture, water management, and the exploitation of soil resources attributed to pre-Aksumite and Aksumite polities between 3000 and 1000 years cal. BP. This finding is consistent with the hypothesis of an early anthropogenic forcing in the Nile River Basin, adding further evidence to the large scale impact of pre-historic human activities on continental weathering.
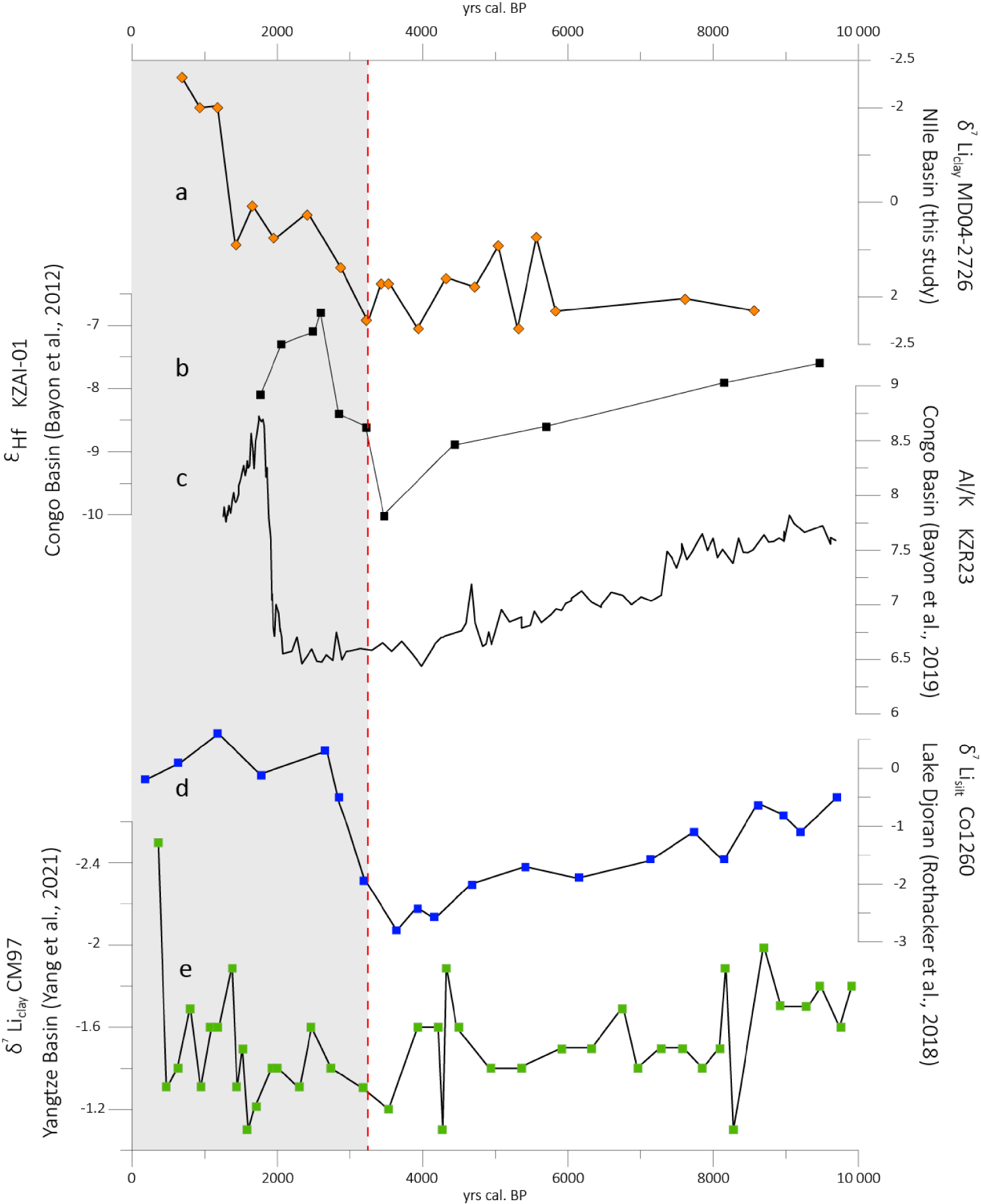
Diagram illustrating the intensity of continental chemical weathering worldwide during the Late Holocene. (a) clay δ7Li of MD04-2726 core (this study); (b) εHf variations in KZAl-01 core [Congo basin; Bayon et al. 2012]; (c) Bulk sediment Al/K ratio in KZR23 core [Congo basin; Bayon et al. 2019]; (d) silt δ7Li of Co1260 [Djoran Lake; Rothacker et al. 2018]; (e) Clay δ7Li evolution determined for the CM97 core [Yangtze basin; Yang et al. 2021].
Several compelling comparisons can be made with other large continental regions. In highland Yemen, a direct correlation has been made between combined settlement increase, development of terraced agriculture, environmental degradation and soil erosion during the Yemen Bronze Age [between 5000 and 4000 BP; Wilkinson 2005, 1997]. Similarly to Ethiopia, Yemen highland erosion is attributed to the combination of climatic drying and major societal changes, which left a heavy human footprint on the landscape [Bard et al. 2000; Wilkinson 1997]. In the Congo Basin, Bayon et al. [2012, 2019] reported an abrupt event of intense chemical weathering during the third millennium BP. During this time interval, the highest aluminum/potassium (Al/K) and hafnium isotopic (εHf; Figure 4b) ratios of the last 40 thousand years were encountered in deltaic records recovered near the mouth of the Congo River. In Central Africa, this weathering event coincided with a collapse of the rainforest and accelerated soil erosion, but also with the southward migration of Bantu-speaking people from a region located between Nigeria and Cameroon [Bayon et al. 2019, 2012; Garcin et al. 2018; Saulieu et al. 2021; Seidensticker et al. 2021]. While debate exists about the cause of this major environmental crisis, several authors have suggested that both the vegetation shift and the chemical weathering event were partly caused by an intensification of iron smelting and land use related to the Bantu expansion [Bayon et al. 2012; Garcin et al. 2018; Bayon et al. 2019]. In south-eastern Europe, Rothacker et al. [2018] reported an anomalous (i.e. unrelated to the climate trend) increase of δ234U and δ7Li values in a sedimentary record from Lake Dojran (Greece) over the last 3300 years cal. BP. These isotopic variations have been interpreted as a marker of both intensive soil erosion and chemical weathering (Figure 4c). Combined with additional biomarker data and inferences from archaeological studies, this feature coincided with the first signs of agriculture in the Dojran basin, as well as the development of cultivated and ruderal plants. Eventually, in China, Yang et al. [2021] suggested that small but significant Li isotope fluctuations recorded in a sediment core from the Yangtze delta since ∼2 ka, were associated with increase in sedimentation rates, and might be related to the rapid development of agricultural practices and other human activities in the lowland region of the Yangzte River basin (Figure 4d).
Although the number of case studies using chemical weathering proxies remains limited to date, most of them highlight an early anthropogenic forcing on continental chemical weathering over the last few thousand years. Additional high temporal resolution studies are now needed in order to determine the late Holocene origin and evolution of chemical weathering at a global scale, and to precisely quantify the environmental impact caused by the rise of early polities worldwide.
5. Conclusions
Over recent years, arguments in favour of human-induced climate alteration during prehistoric times have provided support for the Early Anthropogenic Hypothesis. However, the impact of human activities on chemical weathering and on Earth surface processes remains poorly documented. In this article, we have explored the relationships between the evolution of soil weathering and the rise of early complex polities and urban societies in East Africa, namely during the Pre-Aksumite and Aksumite periods. Using Nd (εNd) and Li (δ7Li) isotopes in the clay-size fraction of marine sediments retrieved from the Nile Deep Sea Fan, we reconstructed the evolution of continental weathering in the Nile Basin over the last 9 ka. Our results show a trend towards higher εNd and very low δ7Li values over the last 3000 years cal. BP, indicating both enhanced weathering processes and sediment transport from the area of the Ethiopian highlands drained by the Blue Nile. The negative δ7Li values, which are unprecedented over the last 100 ka, suggest that this event cannot be explained by natural hydroclimatic forcing. Instead, using archaeological, paleo-pedological and paleo-botanical data, we observed that this weathering event was concomitant with the emergence and rise of the Pre-Aksumite and Aksumite polities, which developed after 3000 ka BP in the headwaters region of the Blue Nile. The association between the onset of intensive agricultural and hydrological management by Pre-Axumite and Aksumite populations, and enhanced chemical weathering in the Blue Nile catchment points towards an early anthropogenic degradation of soils in East Africa. Taken together with other case-studies and proxy records worldwide, we posit that human activities have acted as a major driver of continental chemical weathering since 4.3-3 ka BP, driving the hypothesis of an Earlier Anthropogenic disruption of the Earth Critical Zone over a larger scale.
Conflicts of interest
Authors have no conflict of interest to declare.
Acknowledgements
This work was financially supported by BQR Geoazur- OCA, an INSU-SYSTER grant and the ANR INTOCC (ANR-15-CE31-0013). The MS27PT and MD04-2726 cores were recovered during the MIMES and VANIL cruises respectively. We greatly thank Philipe Télouk for his help with the MC-ICP-MS at the CNRS-INSU national service at Lyon.