1 Introduction
The four successive Intergovernmental Panel on Climate Change (IPCC) reports [8–11], offer a diagnostic of climate change in response to increasing atmospheric greenhouse gases which now covers almost 20 years of scientific research. This diagnostic was made using models of increasing complexity and resolution, but it is remarkably stable over the years [13]. The IPCC First Assessment Report (FAR), for example, used mostly low resolution atmospheric models developed in the late 1980s, generally coupled with a well mixed ocean boundary layer of about 50 m depth. On this basis the FAR described a warming pattern characterized by a response which was more pronounced over the continents and over the polar regions and less intense over the oceans. It also described changes in the precipitation patterns characterized by increased values near the Intertropical convergence zone (ITCZ) or at mid-latitudes and a general tendency toward drier subtropical areas. The results from the recent IPCC Fourth Assessment Report (AR4) have confirmed these patterns on the basis of a new generation of models, characterized by both a higher horizontal and vertical resolution and a more comprehensive physical content, including a coupling with ocean general circulation models, a full representation of soil hydrology, the effects of vegetation and river drainage, and the inclusion of more advanced cloud representations taking into account physical and microphysical processes.
These patterns characterizing the response of the models to increasing greenhouse gases therefore constitute an extremely robust result and this robustness constitutes one of the main elements which have allowed the scientists to alert society and decision makers on the dangers of an upcoming climate change. The indications about climate change distribution inherited from the FAR still constitute, consciously or not, the baseline of most impact studies analyzing the potential effects of climate changes in a given region. They are also, very remarkably, being confirmed now by the observations of current changes in the climate system, which indicate that both the temperature and the precipitation trends tend to follow the predicted patterns [11].
Decision making, however, requires much more precise information. A quantitative estimate of the changes, a more precise regional distribution of their effects, an associated evaluation of their variations, including the possibility of more frequent extreme events are needed to dimension infrastructures or, more generally, take the necessary measures to adapt societies or ecosystems to climate change. Providing this added information to decision makers, however, turns out to be an unexpectedly difficult problem. The assessment of regional impacts is marked by a continuous history of divergent results from the different climate models, as was stressed recently by Morel [16]. The aim of the present paper is to review some of these discrepancies and offer some perspective on their origin. Section 2 evokes some of the reasons explaining the remarkable stability of the global patterns of climate change, while Section 3 is, on the contrary, devoted to a brief review of the thermodynamic feedback effects which may explain why the simulated climate sensitivity is still affected by a large uncertainty. In Section 4 we show that the changes in temperature and precipitation may also depend on changes in the atmospheric circulation. This discussion is supported by a number of diagnostic derived from the AR4 intercomparison exercise [5]. In conclusion, we offer some simple perspectives about uncertainties affecting future climate models.
2 The robustness of global climate change patterns
The very large consensus concerning the main patterns of climate change, the stability of the results through the various IPCC reports necessarily indicate that these patterns are the result of a few dominant physical processes. These processes have been identified over the years. A first issue is that of resolution, since the stability of model results, as model resolution was steadily increasing, may be seen as a surprise. It has been known since the mid-1980s that an atmospheric resolution of about T42 (for spectral models, or equivalently 300 km grid mesh, for finite difference dynamical codes) was enough to resolve the largest part of the mid-latitude energy transport by baroclinic eddies. The more recent analysis of climate models also shows that a number of atmospheric dynamical features are not strongly affected by climate change as resulting from greenhouse increase. Fig. 1 shows the geographical limits of the Hadley cells as diagnosed from the AR4 models [5,6] and the variations which they may undergo in response to a CO2 doubling. The variations due to climate change are small compared to the differences between models. An important component of the climate response may therefore be explained simply by ignoring those changes. We may therefore distinguish: (i) a component of temperature and precipitation changes which does not depend on possible modifications of the Hadley cell extension and may offer some elements of consensus between models; and (ii) a component which does depend on these changes. This second component is less consensual, as the models tend to diverge in the assessment of these Hadley cell modifications.
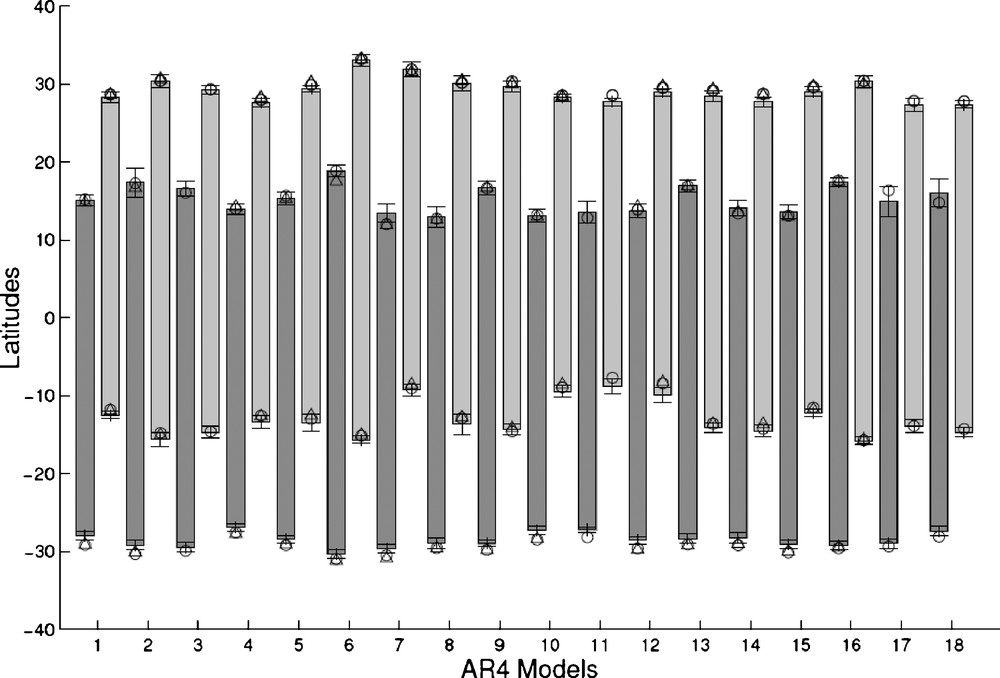
Hadley cell limits: for each of the 18 models, the two bars represent the simulated extension of the winter Hadley cell in the present climate conditions, for the Southern Hemisphere (left bar, June, July, August) and the Northern Hemisphere (right bar, December, January, February). At each bar extremity, the indicated range represents the simulated interannual variability and the circle and triangle the displacement of the cells for, respectively, transient or stabilized warming conditions associated with a CO2 doubling.
Limite des cellules de Hadley : pour chacun des 18 modèles, les deux barres représentent l’étendue simulée des cellules de Hadley dans l’Hémisphère Sud (barre de gauche, juin, juillet, août) et dans l’Hémisphère Nord (barre de droite, décembre, janvier, février). La fourchette à l’extrémité de chaque barre représente la variabilité interannuelle de chaque modèle, alors que les cercles et triangles indiquent les déplacements des cellules de Hadley pour un réchauffement associé, respectivement à des conditions de doublement du CO 2 atmosphérique transitoires ou stabilisées.
In consequence, let us first consider that the limit between the traditional atmospheric circulation regimes (Hadley cells in the Tropics, mid-latitude disturbances) is little affected by climate changes. The most important changes are then driven by thermodynamic rather than dynamic features. The geographical patterns of climate change warming then primarily depend on a few amplifying or feedback factors. The larger warming over the polar regions, although not fully understood, is certainly the combined result of a decreased surface albedo associated with sea ice and snow melting, of the heat accumulation within the Arctic ocean associated with a stronger penetration of the solar flux, and a very stable atmospheric temperature lapse rate which maintains a stronger warming near the ground. The larger continental warming is generally attributed to the larger heat capacity of the oceans, although some have also pointed the different continental and oceanic share between the sensible and latent heat fluxes that participate to the surface cooling in response to radiative heating. The explanations of the global patterns of precipitation changes resulting from a global warming are probably more counterintuitive. The larger precipitation values in the equatorial regions, shown by all models, are not the result of an increasing strength of the Hadley and Walker cell circulation [7]. For example, Fig. 2 [5,6] shows that on the contrary, in most models, the intensity of the Hadley circulation tends to diminish with global warming, as will be further commented in Section 4 [19]. The increased equatorial precipitations therefore mostly result from an increased atmospheric loading in water vapor, associated with the existence of higher saturation levels in a warmer world. The same argument holds to explain larger precipitation values at mid-latitudes. The smaller precipitation values in the dry subtropical areas, a feature which is also shown very systematically by most models, reflect on the contrary, a different physical rationale, probably as robust, although more complex. In a warmer and moister world, the Hadley cells transport more heat and more moisture toward the Equator in the lower atmospheric levels. It must therefore export more geopotential in the poleward direction, to maintain a total transport of energy which is oriented from the equator toward the poles. In this process, the upper part of the Hadley cell and the detrainment of moist air from convective system have to be located at higher altitudes, where the atmosphere is also drier and the subtropical subsiding regions are also drier.
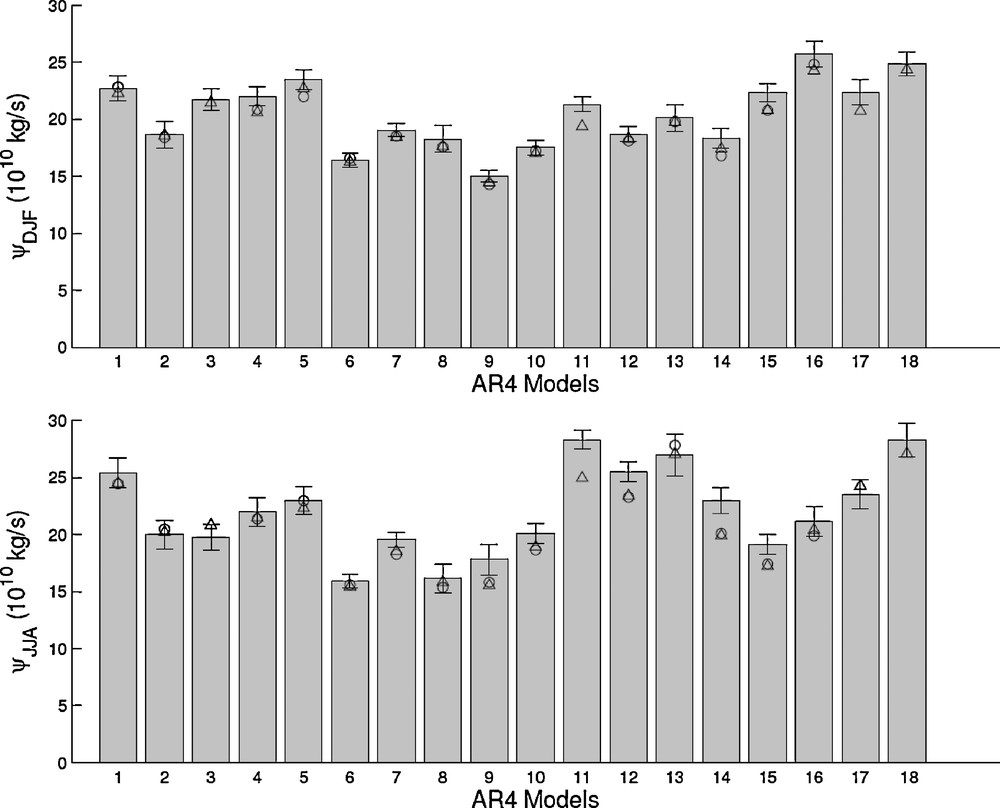
Strength of the winter Hadley cell in the Northern Hemisphere (above, December, January, February) and in the Southern Hemisphere (below, June, July, August) for the same models as in Fig. 1. This strength is diagnosed through the streamfunction of the zonally averaged flow. The indicated range represents the simulated interannual variability, and the circle and triangle indicate the displacement of the change of the cell strength in transient or stabilized warming conditions associated with a CO2 doubling.
Intensité des cellules de Hadley en hiver dans l’Hémisphère Nord (en haut, décembre, janvier, février) et dans l’Hémisphère Sud (en bas, juin, juillet, août) pour les mêmes modèles que laFig. 1. La fourchette à l’extrémité de chaque barre représente la variabilité interannuelle de chaque modèle, alors que les cercles et triangles indiquent les variations d’intensité des cellules de Hadley pour un réchauffement associé, respectivement à des conditions de doublement du CO2atmosphérique transitoires ou stabilisées.
3 Thermodynamic feedbacks
In view of the robustness of climate change patterns associated with temperature and precipitation, it may come as a surprise that the climate sensitivity displayed by the different models can, on the contrary, be very different from one model to the other. Climate sensitivity is defined as the global equilibrium warming which occurs in response to a CO2 doubling. The Charney report, in 1979, based on the results of two models only, determined that climate sensitivity could lie within a range going from 1.5 to 4.5 °C. Fig. 3, from the IPCC Third Assessment report shows that this range has hardly changed over the years, with global equilibrium temperature changes still between 2 and 5 °C approximately. The same figure also shows a general consistency between the global temperature and precipitation changes, which constitutes a good indication that climate sensitivity is an important indicator of climate impacts.
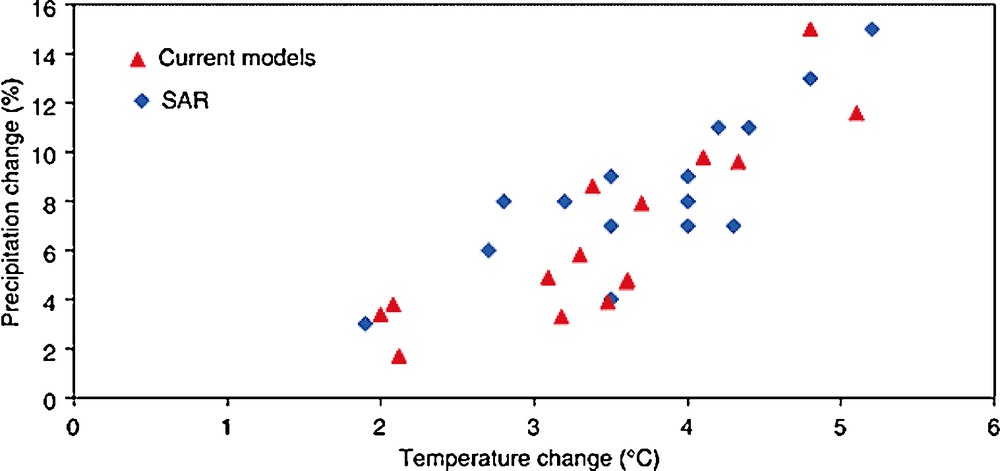
(from [10], using results from Le Treut and McAvaney, [12]) Temperature and precipitation changes corresponding to an equilibrium response to CO2 doubling, from models used in the Second (diamonds) and Third (triangles) Assessment Reports.
(Diagramme du GIEC[10], utilisant des résultats de Le Treut et McAvaney,[12]) Changements de température et de précipitations correspondant à la réponse d’équilibre de modèles des deuxième (losanges) et troisième (triangles) rapports du GIEC pour un doublement du CO2atmosphérique.
This is why such a persisting range in climate sensitivity, also confirmed by the AR4, is very troublesome: better constraining climate sensitivity probably constitutes one of the most important open scientific questions. Over the years, it has been demonstrated that climate sensitivity is dependent on a small number of key feedback processes. The most important of them is probably water vapor feedback, which roughly doubles climate sensitivity, in most models. Following a pioneering paper par Raval and Ramanthan [18], the IPCC Second Assessment Report referred to the existence and importance of this feedback as very consensual. The complexity of the processes involved already mentioned by Lindzen [14], was strongly revisited in the TAR. The idea of the water vapor feedback is indeed based on a simple initial argument: as temperature increases, the Clausius-Clapeyron law indicates than the water vapor saturation value also increases, and that this may cause an increase in the water vapor mixing ratio itself, which in turn favors an additional greenhouse effect. But this water vapor and greenhouse increase is effective only when changes in the water vapor atmospheric content are really dictated by changes in its saturation value, which is not the case, for example, in semiarid areas, or, partly, in the upper atmosphere. In addition, any change in the atmospheric temperature (as may result from a change in the atmospheric temperature lapse rate) will also modify the infrared emission by water vapor and therefore the amplitude of water vapor feedback. The complexity of cloud feedbacks is much larger still, because clouds act both on the terrestrial part of the radiative budget (positive greenhouse feedback) and on the solar part (negative albedo feedback). While models converge in predicting a positive water vapor feedback, the net cloud effect is much less certain. Other feedbacks concern the land surface (snow and ice albedo, land cover) and also add to these uncertainties.
Relating those modeled feedbacks to actual observations is a very difficult task: future climates are of course not documented at all and past climate observations, although very precious, do not cover many atmospheric parameters and processes. The only time span for which we possess a sufficient knowledge of water vapor and cloud modifications is the period in which satellite data have been available, therefore the last 25 years approximately. During this period the earth has experienced a number of climate fluctuations. The most important one is of course the seasonal cycle, but the satellite era is also marked by interannual variability, including a number of ENSO events, volcanic eruptions such as the Pinatubo, etc. Using these changes to assess water vapor and cloud feedbacks is now a classical approach, already developed in the mid-1990s [3], for water vapor feedbacks; [4], for methodological aspects concerning cloud feedbacks. Such a method has been used recently by Bony and Dufresne [2] to show the specific contribution of low clouds to climate sensitivity. This approach is based on the idea that, in the Tropics at least, there is a certain robustness of the thermodynamic feedbacks, which can be characterized according to the atmospheric circulation regimes in which they tend to develop. This approach is applicable to determine future climate changes if we can assume that the atmospheric circulation will not change too much in the future or that we will be able to predict those changes if they occur.
4 Dynamic feedback
We have shown in the preceding sections that a large part of the current consensus concerning model results, e.g. the stability of the global patterns of precipitation and temperature changes or the best hopes to better constrain climate sensitivity are all based on the idea that the atmospheric circulation will not be deeply changed in response to climate change.
Most models confirm the idea that, indeed, atmospheric circulation changes may be held as a second order effect if one considers the global climate response. If this was not the case, the spread between model estimates of climate change would be much larger, in particular at regional scale. In Fig. 2, for example, we have shown the diagnostic of the winter Hadley cell intensity, by a number of models which participated to the last AR4 simulations and to the World Climate Research Programme (WCRP) Coupled Model Intercomparison Programme (CMIP) [5,6]. The range of the results is very large and, knowing the huge importance of the Hadley cell in shaping up the climate distribution over the Earth, this very large spread obtained using recent models only, is really troublesome. In addition, when compared with such variations, the modifications brought up by a CO2 doubling appear to be small and are themselves not characterized by a real consensus between models: although most models indicate a weakening of the Hadley circulations in a warmer world, with a poleward extension of both the Hadley cells and mid-latitude circulations [20], some models show an opposite response.
Such changes in the atmospheric circulation may have an impact larger than anticipated. Pierrehumbert [17] has shown that climate sensitivity results in a large part from the competition between the continuous destabilization of equatorial regions, submitted to a run-away greenhouse effect, and the stabilizing effect of the subtropics. Dynamical processes are necessarily involved in this competition and changes, for example, of the area covered by ascending motions are likely to modify climate sensitivity [1].
In addition, changes in the Hadley cell intensity are generally accompanied by (equally small) changes in the Hadley cell limits, which tend to extend poleward [15]. Gastineau [5] or Gastineau et al. [6] have reviewed some of the mechanisms which may explain the lack of consensus of current models in simulating both the Hadley limits and their changes and how this may in turn induce uncertainties concerning, for example, local changes in precipitations. These uncertainty factors are numerous: the Hadley cell intensity and extension are affected by the atmospheric vertical stratification, or the latitudinal temperature gradients at mid-latitude, which also govern in part the dynamics of mid-latitude perturbations.
These uncertainties are even larger and more systematic if one considers not only the mean meridional circulation but also the more complex features which correspond to longitudinal asymmetries: monsoons, ENSO ... The role of dynamical changes in determining the local aspects of climate modifications can be very important and their study is only beginning.
5 Conclusion
The assessment of the simulated climate changes from various climate models has been carried so far with the objective of alerting government and decision makers on the reality of climatic risk. This alert has been greatly favored because a number of key features in the climate response are organized following very robust patterns, which are confirmed by recent models, with a higher resolution and a more comprehensive physics. This robustness is partly due to the fact that many global aspects of climate changes are not strongly affected by changes in the atmospheric dynamics.
Assessing local climate changes is a much more difficult challenge because it does imply a good understanding of how atmospheric dynamics may be affected by a climate warming. This is a task which is largely in front of us and, at the local or regional levels, model results are still affected by large uncertainties.
A key question for the future is to know what we can expect from the continuing increase in model resolution. A number of parameterization problems affecting climate sensitivity (for example: low cloud formation and dissipation) will not be solved unless model resolution becomes so high that it may capture small turbulent and convective cells in the atmospheric boundary layer. Likewise the nonlinear interactions which, especially at mid-latitudes, condition the predictability, or lack or predictability, of regional climate change patterns will not necessarily be better represented in models with an increased resolution. Increasing model resolution is an important and necessary challenge, but it may not be sufficient. It is one of the necessary advancements if we wish to better explore and predict the regional impacts of climate changes. But it is not the only way of improving models: better formulations and tests of the model physical parameterizations are also necessary. Even with such efforts, the global amplitude of future climate changes, the regional distribution of these changes may very well remain uncertain: success in substantially improving climate projections cannot be warranted at this stage.