1 Introduction
The tectonic knowledge of the Pyrenees benefited greatly more than 20 years ago from the acquisition of the ECORS deep seismic reflection profiles (Choukroune et al., 1989; Daignières et al., 1994). Subsequent crustal-scale models for the Pyrenean orogeny included restored sections to the pre-orogenic state that depicted a Mesozoic rift basin floored by a thinned continental crust (Beaumont et al., 2000; Muñoz, 1992; Roure et al., 1989; Teixell, 1998). In recent years, a revisit of the significance of the Pyrenean lherzolites and the application of concepts from passive continental margins led to new understandings of the pre-orogenic reconstruction, invoking extreme crustal attenuation and mantle exhumation during Mid-Cretaceous times (Clerc and Lagabrielle, 2014; Jammes et al., 2009; Lagabrielle and Bodinier, 2008; Lagabrielle et al., 2010; Masini et al., 2014; Tugend et al., 2014). In parallel, new paleothermometrical data have shown a generalized state of high heat flow during the mid- and late Cretaceous, beyond the well-known areas of North-Pyrenean HT metamorphism (Clerc et al., 2015). This heat flow may account for the isostatic equilibrium between extreme crustal thinning and moderate synrift sedimentary thickness and paleobathymetry in the Cretaceous basin (Winnock, 1974), as the hot nature of the Cretaceous margins and exhumed mantle domain counteracted the expected subsidence.
New models for the evolution of the late Cretaceous to Cenozoic Pyrenean orogeny can be now developed on the account of these pre-orogenic reconstructions. These models must now take into account feedbacks between the mid-Cretaceous hyperextension and the Pyrenean inversion as well as detailed geological and geophysical observations. The purpose of this paper is to revisit the orogenic evolution of the west-central Pyrenees based on the sequential reconstruction of a new crustal transect (Fig. 1), which, together with tectonics-sedimentation and thermochronological constraints, allows us to address specific points including the identification of the ancient margins and suture, the fit between the upper and lower crustal structure, the role of decoupling at different scales, and the timing and magnitude of orogenic shortening.
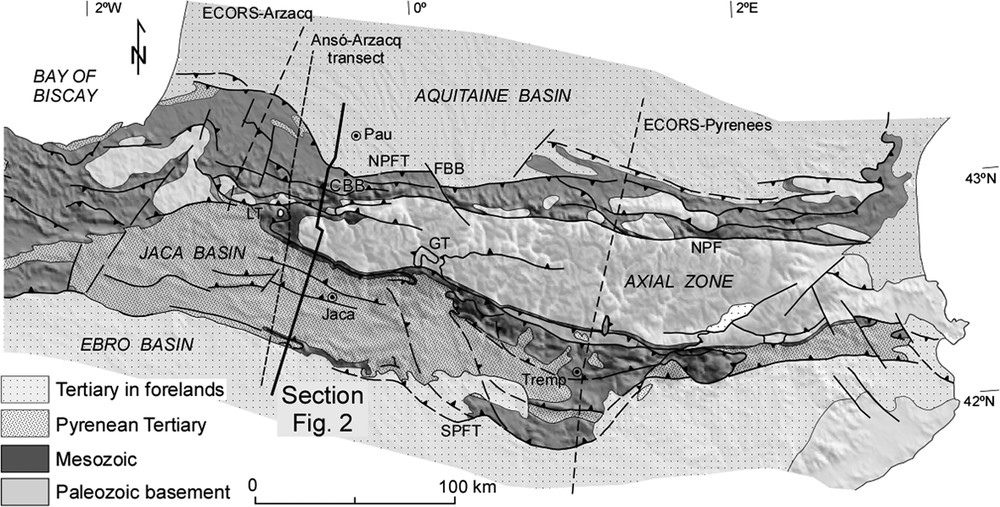
Geologic sketch map of the Pyrenees showing the location of the study section (Fig. 2) and other seismic or geologic transects discussed in the text. CBB: Chaînons Béarnais belt; FBB: transfer fault at Bagnères-de-Bigorre; GT: Gavarnie thrust; LT: Lakora thrust; NPF: North-Pyrenean fault; NPFT: North-Pyrenean Frontal thrust; SPFT: South-Pyrenean Frontal thrust.
2 Section of the west-central Pyrenees: identification of ancient continental margins and suture
A new crustal section of the west–central Pyrenees is presented in Fig. 2. It benefits from our current investigations of the northern Pyrenees in the frame of the ANR Pyramid project. The section runs across the Jaca basin, the western Axial Zone and the Chaînons Béarnais, 10–15 km to the east of the Ansó–Arzacq transect of Teixell (1998) (Fig. 1). Descriptions of the surface geology can be found in the works by Labaume et al. (1985), Teixell and García-Sansegundo (1995), Teixell (1996), Biteau et al. (2006), and Lagabrielle et al. (2010).
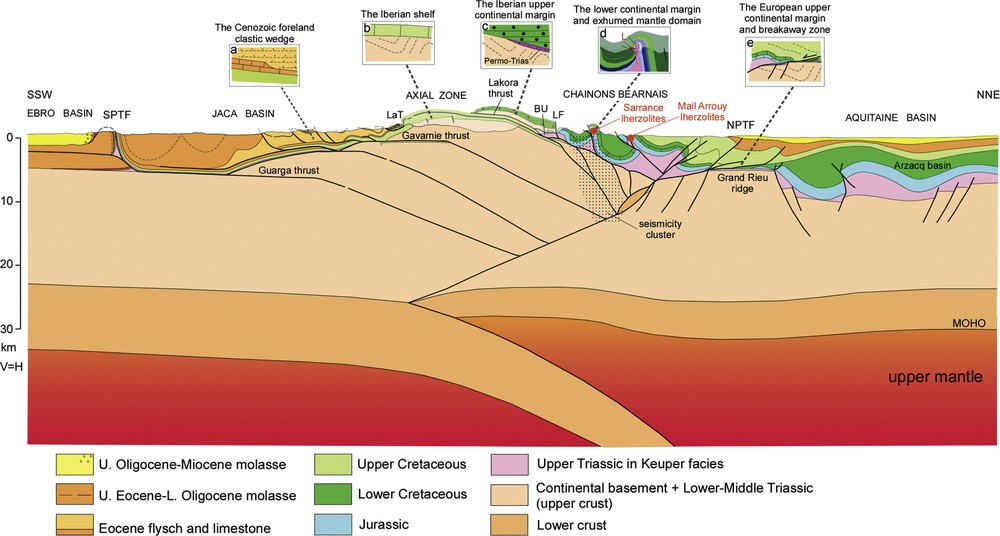
Crustal section of the west-central Pyrenees (location in Fig. 1). The near-surface geology is based on published sections (see text for references), combined with new observations and an unpublished section of the North-Pyrenean Frontal thrust area (courtesy of Total). The deep crustal structure is based on the interpretation of the ECORS-Arzacq seismic reflection profile and on receiver functions analysis of the PYROPE and older surveys (see text). The stippled area represents the cluster of microseisms characteristic of the North-Pyrenean Zone projected after Souriau et al. (2014), where the vertical precision of the seismic event location is of 3 km (Dumont et al., 2015). Inset boxes represent key features of the Mesozoic and Cenozoic basins for the reconstruction of the pre-orogenic architecture shown in Fig. 3 (see text for explanation). Inset d shows the present-day state of the Sarrance unit, including the Saraillé lherzolite (L). SPFT: South-Pyrenean Frontal thrust (External Sierras); NPFT: North-Pyrenean Frontal thrust; LF: Licq fault; BU: Bedous Triassic unit; LaT: Larra thrust.
A remarkable feature of this transect is that almost the entire profile in the near-surface is characterized by south-verging thrusts and folds, with the exception of the North-Pyrenean Frontal thrust zone. In the southern part, the Ebro and Jaca basins are Cenozoic foreland basins separated by the complex thrust front of the External Sierras. The External Sierras and the Jaca basin are detached in upper Triassic evaporites and shales (Keuper facies). The overlying Mesozoic succession is thin (a few hundred meters of shallow water and terrestrial deposits), whereas the synorogenic Tertiary succession is several thousand meters thick, including flysch and molasse. Under the northern Jaca basin and Axial Zone, thick-skinned basement thrusts uplift the basin floor (e.g., the Guarga and Gavarnie thrusts), with a structural relief of ca. 9.5 km with respect to the regional elevation of the Ebro basin basement. The Axial Zone corresponds to the hanging wall anticline of the Gavarnie thrust (Fig. 1).
North of the Axial Zone is the Lakora thrust with a large displacement (ca. 17 km), carrying a thin thrust sheet composed of the Iguntze–Mendibelza basement massifs and their cover of Albian conglomerates (Teixell, 1993). A lower thrust branch, the Larra thrust, propagated in the Axial Zone cover up to the Middle Eocene strata of the Jaca basin with ca. 5 km of displacement. The Iguntze–Mendibelza massifs have been eroded in the transect line, but are preserved 3 km to the west. They overlie an intermediate thrust unit of upper Triassic rocks (the Bedous unit) and are bound to the north by a Cretaceous normal fault, the Licq fault. North of the Licq fault, the Chaînons Béarnais belt (CBB) is a system of Jurassic to Lower Cretaceous carbonate anticlinal ridges separated by synclines with thick Albian flysch, passing to Upper Cretaceous flysch in the north. The CBB is detached in the Upper Triassic, folding resulting from the rising and squeezing of diapiric salt walls initiated during the Jurassic–Early Cretaceous extension (Canérot, 1988; Labaume and Teixell, 2014). The basement under the CBB can be inferred to plunge northwards, until it attains the regional elevation of the Aquitaine basin. A system of south-verging basement thrusts involving Upper Paleozoic rocks under the Bedous unit and CBB, not exposed in the study transect, is schematically projected from observations in the Aspe and Ossau valleys, a few kilometers east of the section. Under the northern CBB, a shallow slice of lower crust or mantle material is inferred from a Bouguer gravity high (Casas et al., 1997; Souriau et al., 2014). A north-dipping seismic band is reported to the south of the gravity anomaly (Souriau et al., 2014). At the northern edge of the CBB, the North-Pyrenean Frontal thrust (NPFT) appears in the Mesozoic–Paleogene succession as an imbricate fan of north-verging thrusts, poorly emergent to the surface (Biteau et al., 2006), and here pictured according to an unpublished section (courtesy of Total). The NPFT is interpreted at depth to continue along a south-dipping crustal ramp (Teixell, 1998). A few spaced folds and thrusts of Paleogene age occur in the Aquitaine foreland up to about 50 km north of the NPFT; their total shortening is limited to a few kilometers (Biteau et al., 2006).
The cross-section in Fig. 2 is completed to the crust-mantle boundary according to geophysical data. The depth and extent of the lower crust and Moho are taken from refraction seismic data in Daignières et al. (1982) and from the interpretation of the ECORS-Arzacq reflection profile by Teixell (1998), recently supported by receiver function analysis (Chevrot et al., 2015; Díaz et al., 2012), which shows a slab of Iberian lower crust plunging into the mantle until a depth of ca. 60 km.
Pieces of crust and mantle rocks (lherzolites) occur in the core of the CBB thrust-fold ridges at the base of the Mesozoic series, generally embedded in tectonized remnants of Triassic Keuper sediments (cf. the Sarrance and Mail Arrouy lherzolites close to the section in Fig. 2), or locally reworked in the Lower Cenomanian flysch (western end of the Mail Arrouy at Urdach; Lagabrielle et al., 2010). They attest that the CBB derive from a Cretaceous domain of hyperextended continental crust and exhumed mantle (Jammes et al., 2009; Lagabrielle et al., 2010), requiring the existence of a central domain floored by peridotite between the continental margins of Iberia and Europe. The insets in Fig. 2 highlight features of the Mesozoic and Cenozoic basins, which are key elements for the reconstruction of the pre-orogenic architecture. Inset 2a illustrates the South-Pyrenean foreland basin, where synorogenic carbonate and clastic sequences record a progressive southward depocenter migration and provide timing constraints for the main south-verging thrusts (from Lakora to Guarga, e.g., Teixell, 1996). As for the pre-orogenic configuration, four key elements can be identified in the present section:
- • the Iberian shelf (inset 2b), characterized by upper Cretaceous limestones directly overlying the Paleozoic of the Axial Zone;
- • the upper Iberian continental margin (inset 2c), now represented in the Iguntze–Mendibelza allochthonous massifs, where a proximal conglomerate is laterally equivalent to the lower part of the North-Pyrenean Albian–Cenomanian “Black Flysch” (the Mendibelza conglomerate, Boirie and Souquet, 1982; Souquet et al., 1985) laps southwards on Upper Paleozoic rocks or Permo-Triassic red beds. This landward onlap attests to basinward tilting of the margin. The occurrence of remains of Permo-Triassic red beds may indicate that Upper Triassic Keuper facies and a Jurassic–Cretaceous cover existed there once, but they probably glided down the margin before the onlap of the Albian conglomerate (Ducasse et al., 1986). The basal boundary of the conglomerate has been interpreted as an extensional detachment fault (Johnson and Hall, 1989; Masini et al., 2014). However, this contact is actually a stratigraphic onlap rather than a fault, and there are no clues that it roots in a basement-involved extensional ramp as depicted in Masini et al. (2014). We interpret the basinward tilting of the Iguntze–Mendibelza massifs as resulting from necking of the upper continental margin (see also Tugend et al., 2014). Assuming that an Upper Triassic–Lower Cretaceous cover was ever there, this cover glided down gravitationally along the basal décollement which now underlies the CBB (later reactivated as a thrust);
- • the lower Iberian continental margin and the exhumed mantle domain (inset 2d), represented by the CBB squeezed diapiric system containing ultramafic bodies. The occurrence of shallow water carbonates up to the Upper Aptian indicates that the CBB units were originally on relatively thick continental crust, and glided down to the exhuming peridotite domain across the stretching continental margin during the Albian–Cenomanian (and later on climbed back up the margin to overlie the present-day continental crust). In parallel with extreme stretching and gliding, temperatures up to 300–350 °C were attained in the Jurassic–Cretaceous sediments (Clerc et al., 2015). The fragments of upper crustal rocks and mantle lherzolites were collected along the Triassic décollement (Lagabrielle et al., 2010; Corre et al., 2016) during the extensional and/or the later contractional gliding;
- • the upper European continental margin, represented by the basement high of Grand Rieu (inset 2e), a Cretaceous horst where the Jurassic and Lower Cretaceous are truncated by what we view as an extensional fault breakaway zone, from which they glided southward down the margin before inversion along the NPFT. In the southern part of the Grand Rieu ridge, the Upper Cretaceous locally rests on the Lower Triassic, a situation comparable to the Iguntze–Mendibelza and Axial Zone massifs to the south.
The described configuration leads to identify the Iberian continental margin in the Lakora thrust sheet and in the present-day basement of the CBB. It follows that the European continental margin must be largely buried (underthrust) under the deep ramp of the NPFT, and then the NPFT must be considered as the suture zone itself, at the level of the upper and middle crustal basement. These inferences form the basis for a restored model for the west-central Pyrenees before the Pyrenean orogeny, presented thereafter.
3 A model for the Cretaceous basin
The section in Fig. 3 represents a model for the pre-orogenic basin restored to the Late Cretaceous. The reconstruction is based on restored layer lengths and often constrained fault cutoffs, so it adjusts to the true dimensions of the known elements of the continental margins. The reconstructed width of the exhumed mantle domain between the margins is uncertain. The restored separation between the lherzolite bodies at Sarrance and Mail Arrouy ridges is ca. 15 km, which gives a minimum width (see discussion below). The geological elements described favor a model of distributed deformation conforming to a boudinage-like geometry, with relatively narrow continental margins (< 40 km wide). In these margins, the crust is progressively thinned away from the shelf edges at the northern Axial Zone and Grand Rieu ridge, with a relatively smooth topography of the upper continental basement (sloping ∼10°). Long-offset faults or tilted fault blocks as reported by Ducasse et al. (1986) and Masini et al. (2014) for the Mauléon basin are not evidenced in the studied transect. We propose instead a restored geometry similar in style and dimensions to the stretched margins of the Parentis basin imaged by the ECORS-Biscay seismic profile (Bois et al., 1997; Pinet et al., 1987).
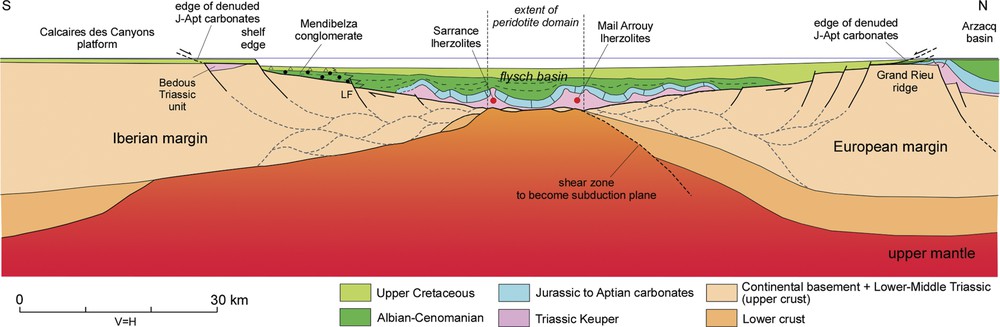
Restored version of the central part of the study transect to the Late Cretaceous (Santonian) showing the pre-orogenic architecture of the continental margins, the exhumed mantle domain and the Mesozoic basin (see text for discussion). Dashed lines represent an interpretative pattern of anastomosed shear zones contributing to crustal thinning. LF: Licq fault.
A similar mechanism of stretching with distributed deformation was envisaged for the distal margins in the eastern North-Pyrenean Zone by Clerc and Lagabrielle (2014). Here, we infer that both the proximal and distal portions of the margins are involved in large-scale ductile necking, as indicated by the basinward-tilted proximal Iberian margin below the Mendibelza conglomerate. This does not preclude the existence of extensional faults in the uppermost crust, but without major displacements to produce landward tilting or to break the continuity of the Triassic décollement level along the margin. We interpret the distal Iberian margin as constituted by upper crustal material at the top-basement surface, as suggested by the nature of the crustal fragments, occasionally adjacent to mantle bodies, embedded in the Triassic of the CBB. Most of these crustal fragments show mylonitic deformation (Corre et al., 2016). Hence, this distal upper crust was probably ductily stretched and left behind a dismembered lower crust, in analogy with observations in modern distal margins (Angola, Brazil; Contrucci et al., 2004; Unternehr et al., 2010) and numerical models (Huismans and Beaumont, 2014).
In this framework, the Mesozoic sedimentary cover was detached in the Triassic Keuper facies (Lagabrielle et al., 2010), denuding the upper margins and constituting a floating lid between the two stretching continental margins. The restoration of the CBB leads to an ancient system of salt anticlines (salt walls) without fault rollovers or rafts, separated by synformal minibasins with continuity of the Jurassic–Cretaceous carbonate layer (Labaume and Teixell, 2014). Indeed, detailed mapping by the latter authors does not support the existence of extensional rafts of carbonate layers now separated by large thrusts, as proposed by Jammes et al. (2009) and Tugend et al. (2014). The Albian–Cenomanian Black Flysch deposits (Souquet et al., 1985) are thought as having accumulated during the period of maximum extension and having onlapped the denuded segments. On the other hand, an early, prefolding bedding-parallel fabric conspicuous in the Jurassic to Albian succession of the CBB (grain-shape foliation in recrystallized carbonates, slaty cleavage in pelitic rocks) indicates ductile stretch, which probably accommodated a significant component of extension.
Hence, the west-central Pyrenees share elements with the central and eastern Pyrenees hot paleomargins (Clerc and Lagabrielle, 2014), consistent with paleotemperatures of 300–350 °C in the basin. Following inferences from the east and from the mylonitic deformation of basement blocks in the Triassic of the CBB (Corre et al., 2016), a network of anastomosed shear zones is sketched in Fig. 3, representing a ductile extensional duplex stretching the upper crust in the distal margins. Lozenge-shaped bodies may have included lower crustal boudins, which were later incorporated into the North-Pyrenean basement and are now attested to by gravity (Casas et al., 1997; Souriau et al., 2014). The width of the Pyrenean margins deduced from section restoration (< 40 km) is comparable to some present-day, non-inverted passive margin tracts as the continental slope of the Armorican margin (Thinon et al., 2003), although they are markedly narrower than others like the South Atlantic margins (Peron-Pinvidic et al., 2013). On the other hand, another thick Cretaceous basin extends north of the Grand Rieu ridge, the Arzacq basin (Fig. 2). This can be viewed as a proximal expression of the stretched European plate, integrated into a wide continental margin (150 km across), in which the Grand Rieu ridge corresponds to a marginal high.
4 Sequential restoration of the orogenic evolution
The timing of the Pyrenean compressional structures wherever known from tectonics–sedimentation relationships or thermochronology is combined with displacement transfer considerations to propose a sequential reconstruction of the studied transect to key steps. This sequential reconstruction is based on the restored section featuring the hyperextended crust and exhumed mantle, just before the onset of the orogeny (Fig. 4a). Due to inherent difficulties in reconstructing paleotopography, topographic and bathymetric issues are not addressed in a quantitative way.
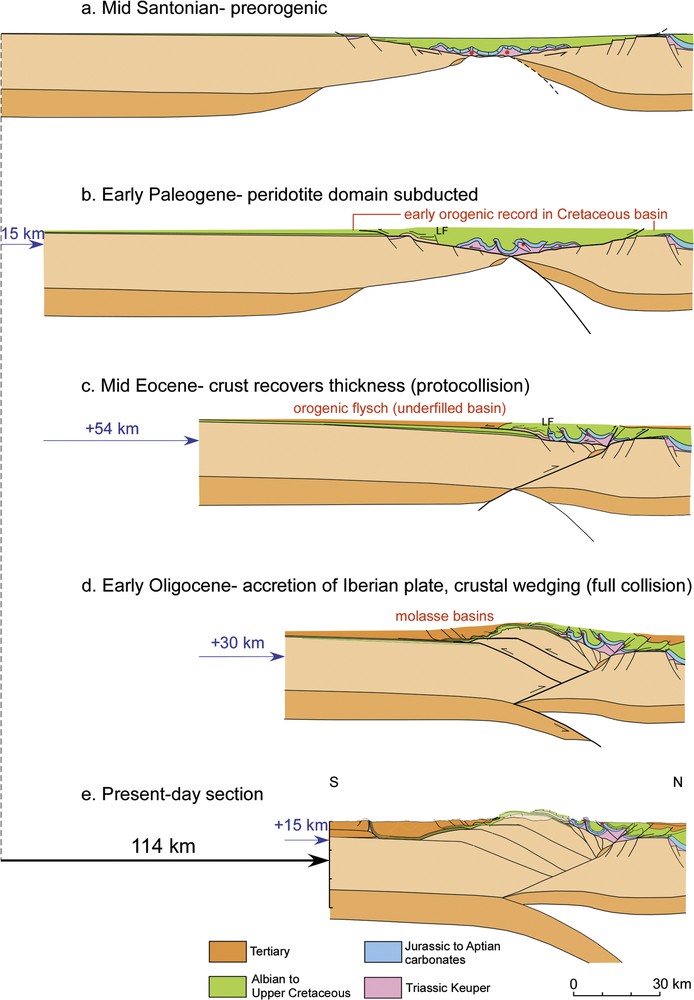
Sequential reconstruction of the crustal-scale section of Fig. 2 to selected steps in the orogenic history of the west-central Pyrenees (see text for explanation).
We infer that the early stage of Pyrenean convergence in the Late Cretaceous involved the closure of the exhumed peridotite domain, by subduction of the mantle lithosphere (Fig. 4b). There is no evidence for sediments being subducted: the sedimentary cover of the Cretaceous basin was detached and thrust as a pop-up along the Lakora thrust and NPFT. A similar process is inferred for the east-central Pyrenees at that time (Mouthereau et al., 2014). The sedimentary succession of the CBB slipped back up the continental margins and the salt anticlines were squeezed and thrust, contributing to lift the lherzolite bodies and distal margin fragments. The Licq fault acted as a local buttress and was shortcut by the Lakora thrust. The earliest record of convergence in the vicinity of the two main active thrusts is recorded by the flexural drowning of the Upper Cretaceous shelf limestones in the present-day Axial Zone (Teixell, 1996), and by a fan of growth strata in the Campanian–Maastrichtian flysch in the footwall of the NPFT (Poitevin et al., 2014). The west-central Pyrenees were poorly emergent at that time, as indicated by the turbiditic nature of sedimentation where preserved on both sides of the CBB, and by the maintenance of high temperatures in the North-Pyrenean Zone before an onset of cooling at ca. 50 Ma (according to thermochronology modeling by Vacherat et al., 2014 and Bosch et al., 2016).
A change occurred when the Iberian and European margins met after complete subduction of the peridotite domain. The present-day juxtaposition of the margins dictates that the early collision was accommodated by the overriding of the Iberian margin onto the top of the European margin along the deep ramp of the NPFT, which sutured the plates (Fig. 4c). During this stage, labeled as “protocollision”, the continental crust recovered its prerift thickness. Thrusting was accommodated in the basin by continued slip of the NPFT as well as by the Lakora–Larra thrust, which experienced its period of major displacement. It should be noted that, at variance with a previous interpretation of Teixell (1998) and Dumont et al. (2015), the Lakora thrust is envisaged as a detachment structure, not rooted in a south-directed crustal ramp. It is difficult to ascertain the precise time when the protocollision initiated. Mouthereau et al. (2014) placed the initial suturing in the east-central Pyrenees in Late Campanian times (70–75 Ma), based on thermochronology and increased subsidence in the Aquitaine foreland basin towards the end of the Cretaceous. However, a subsidence increase in the northern foreland of the west-central Pyrenees is not recorded before 54 Ma (Desegaulx and Brunet, 1990), and the onset of cooling in the Mauléon basin and in the CBB at ca. 50 Ma (Bosch et al., 2016; Vacherat et al., 2014) suggests that the overriding of the continental margins in the study transect probably begun in Early Eocene times. It was certainly ongoing during the Mid-Eocene, causing the rapid flexural subsidence and southward migration of the Ypresian to Lower Bartonian turbiditic clastic wedge ahead of the Lakora thrust (Barnolas and Teixell, 1994; Labaume et al., 1985). The study segment of the Pyrenean orogen emerged slowly during this protocollision stage, as the underfilled flysch troughs on both sides of the belt had main source areas in the east (Mutti, 1984; Serrano, 2001). The first transverse provenance is not recorded before late Lutetian times in the uppermost turbidites on the southern side (Remacha et al., 1998; Roigé et al., 2016) and syn-orogenic conglomerate also begun to accumulate during the Lutetian on the northern side (Biteau et al., 2006; Serrano, 2001). Hence, cooling recorded in the northern Pyrenees in Early to Early–Mid Eocene times (Bosch et al., 2016; Vacherat et al., 2014) could reflect exhumation, but more likely it may reflect a temperature drop in the upper crust, as the latter was regaining thickness by the overriding of the continental margins at the suture (Fig. 4c).
By Late Eocene times, full collisional deformation was expressed by the thick-skinned thrust accretion of the Iberian shelf basement (Fig. 4d). A piggy-back sequence of thrust propagation in the Iberian plate, rooted in the former suture, was initiated by the Gavarnie thrust (Late Eocene to Early Oligocene; Teixell, 1996; Jolivet et al., 2007), which uplifted the Axial Zone ramp anticline and caused exhumation of the leading edge of the previously emplaced Lakora thrust sheet and its immediate footwall (Bosch et al., 2016). A subsequent major displacement along the Guarga thrust (Fig. 4e) caused the main emergence of the External Sierras mountain front in Late Oligocene to Early Miocene times, and produced a period of accelerated exhumation of the granitic massifs of the Axial Zone in the hinterland (Bosch et al., 2016). This basement thrust sequence is associated with the creation of strong structural relief and topographic emergence, recorded in the thick molasse sequences of the Jaca and Ebro basins spanning from the Late Eocene to the Early Miocene (Puigdefàbregas, 1975), as well as in the coeval molasses of the Aquitaine basin (Biteau et al., 2006). We propose that this stage begun by late–Mid to Late Eocene times when the stretched tracts of lower crust of the two plates met and collided, triggering the north-directed subduction or underthrusting of the Iberian lower crust. This created a crustal root and high topography at the surface, which promoted erosion and molasse production into the foreland basins.
The architecture displayed in Figs. 4d and e implies an efficient decoupling between the upper and lower crust for the Iberian plate. Propagation of deformation into the Iberian plate implies that the European plate was operating as a backstop, favoring accretion in the proside of the orogen (e.g. models by Jammes and Huismans, 2012). However, the preexisting strong anisotropy of the NPFT suture promoted indentation of this backstop, and the accreted thrusts of the Iberian plate rooted in the NPFT rather than in the propagating décollement between the upper and lower crust (i.e. the wedge tip) (Figs. 4d, e).
The comparison of the present-day and of the completely restored section (including the exhumed mantle domain with a minimum width estimate) yields a total orogenic shortening of ca. 114 km for the studied transect. The sequential restoration suggests some 15 km of early shortening associated with the subduction of the peridotite domain (Fig. 4b), followed by ca. 54 km in the protocollision stage, associated with crustal ramping along the deep NPFT and internal shortening of the CBB basement (Fig. 4c), and by ca. 45 km of shortening during the full collisional stage, largely accommodated by south-directed thrusting in the southern Pyrenees (but with a certain component along the NPFT) (Fig. 4d,e). Errors and potential underestimations of the total shortening value are discussed below.
5 Discussion
Our reconstruction implies that in the studied transect, the basement of the North-Pyrenean Zone (CBB and Lakora unit) belongs to the Iberian plate. The dense body under the northern part of that zone may represent a fragment of lower crust or mantle belonging to the edge of the Iberian or the European margin, marking the suture zone at the NPFT. The North-Pyrenean seismicity cluster (Fig. 2), which is characterized by normal-fault focal mechanisms, may represent extensional sinking of this dense body (Souriau et al., 2014), although its shape and depth extent are largely unknown. Following the image of the ECORS-Pyrenees profile (Roure et al., 1989), we have represented the body as a thrust slice of lower crust, which we tentatively placed as a dismembered piece of Iberian lower crust in the restoration.
Major displacement along a suture at the deep NPFT ramp is required not only by the present-day superposition of the continental margins, but also to account for the imaged position of the deep Iberian and European crusts. Should the European plate override the Iberian plate along the Lakora thrust, the leading edge of the European lower crust would be under the northern North-Pyrenean Zone (Jammes et al., 2009). However, seismic reflection and receiver function interpretation place the edge of the deep European plate further south, under the northern Axial Zone (Díaz et al., 2012; Masini et al., 2014; Teixell, 1998). Interpreting the basement of the North-Pyrenean Zone as belonging to the Iberian plate is at variance from the eastern and east-central Pyrenees, where the exposed North-Pyrenean basement massifs are ascribed to the European plate (Clerc and Lagabrielle, 2014; Lagabrielle et al., 2010; Mouthereau et al., 2014; Vissers and Meijer, 2012) (Fig. 5). This implies a major change from an eastern segment where the suture is between the Axial Zone and the North-Pyrenean Zone (namely the North-Pyrenean Fault), below which the Iberian margin has been underthrust, to a western segment where the European margin has been underthrust during the protocollision stage (Fig. 4c). Further research of the transfer zone is needed, though we speculate it is to the west of the Barousse basement massif, possibly in the cross-fault zone near Bagnères-de-Bigorre (FBB in Fig. 1). The position of the suture in the west-central Pyrenees is well defined at the level of the crustal basement. In contrast, in the Mesozoic–Cenozoic sedimentary cover, the plate boundary appears rather as a diffuse zone, comprising the entire bivergent prism ejected between the Lakora thrust and the NPFT as the crustal basement blocks collided.
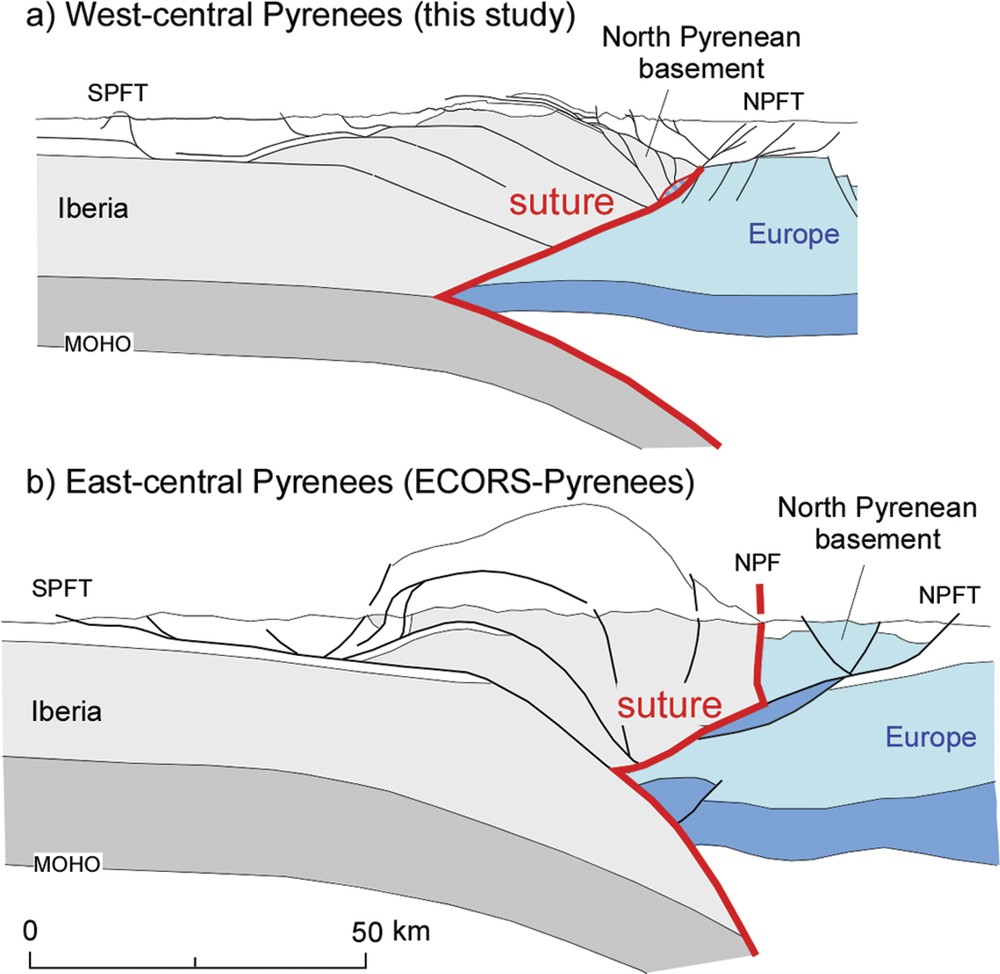
Comparison of crustal sections across the western and eastern parts of the central Pyrenees showing the Iberian (grey) and European (blue) plates and the position of the suture between them (red). The ECORS-Pyrenees section in (b) is simplified from Beaumont et al. (2000) (location in Fig. 1). SPFT: South-Pyrenean Frontal thrust; NPFT: North-Pyrenean Frontal thrust; NPF: North-Pyrenean fault.
With the available information, the upper segment of the NPFT near the surface is represented as a relatively low-displacement structure (after a cross-section reproduced in Fig. 2 by courtesy of Total), which may seem at odds with the large displacement envisaged for the deep segment of the thrust at the basement level (constrained by the thickness of the European crust being cutoff obliquely, i.e. at least 35 km). A component of this large displacement was transferred backwards into the cover detachment of the CBB and the Lakora thrust (Fig. 4c), and an undetermined component of displacement may have also been transferred to the Aquitaine basin as bedding-parallel detachments. With poor exposure and imaging in seismic profiles, a special research focus on the NPFT is needed to address issues as the actual shortening and displacement transfer.
The upper crustal geometry of the European margin is obscured under the NPFT and little direct information can be retrieved. As for the Iberian margin, the nature of basement fragments in the Triassic and the actual crustal configuration led us to tentatively reconstruct the distal margin as formed by upper crust. Diverse mechanisms of lower crust removal in distal margins are discussed by Reston (2007); a detailed assessment of these mechanisms is beyond the scope of this paper, although for the Pyrenean paleomargins we favor models involving heterogeneous stretching at the crustal scale. The restored geometry for the Iberian margin here proposed could be a representative of Huismans and Beaumont's (2014) model III for continental margins, where a strong lower crust is removed and advected away from the thinned margin.
The proposed model is simply a geometrical reconstruction of the immediate pre-orogenic state, and therefore it does not address the kinematics by which the two continental margins arrived to that position during the Jurassic and Early Cretaceous (including the left-lateral drift of Iberia). A large strike-slip component between Iberia and Europe during the Albian-Cenomanian thinning stage is proposed on certain reconstructions (Olivet, 1996). In contrast, other authors propose that the lateral drift of Iberia essentially occurred before the Albian or Aptian (Jammes et al., 2009). Our geometrical reconstruction of the margins does not provide elements to support unequivocally one model or the other. Narrow margins such as ours are more often observed at transform margins than at classical orthogonal passive margins, which are typically longer (Mascle and Basile, 1998), but diagnostic evidence for strike-slip or pull-apart basin opening mechanisms during the Albian–Cenomanian is lacking.
At the level of the deep crust in the present-day cross-section, the resolved geometry of the deep NPFT and the seismic images of the lower crust favor a model of crustal-scale indentation similar to that proposed by Teixell (1998), recently supported by a study of teleseismic P-to-S wave conversions by Chevrot et al. (2015). As discussed above, our data do not support a model where the Lakora thrust plunges down to offset the lower crust and Moho, and neither provide evidence for the Guarga thrust to oversteepen abruptly downsection to link with the lower crust and Moho offset (Muñoz, 2002). A removal of the lower crust in extension would agree with the limited extent of the lower crustal root imaged in seismics (50–60 km deep, Chevrot et al., 2015).
The ca. 114 km shortening here calculated represents ca. 35 km more than that calculated for the Ansó-Arzacq section by Teixell (1998). A minor part of this difference may be explained by the general trend of shortening reduction from east to west in the Pyrenees. The rest will be associated with the denudation of the Mesozoic in the continental margins in parallel with the opening of the exhumed peridotite domain, which was not considered before. Certainly the contrast that still remains between the shortening here calculated from geological data with the 160–180 km of Iberia–Europe convergence since the Late Cretaceous required by certain plate kinematic models (see synthesis in Vissers and Meijer, 2012 and Mouthereau et al., 2014) leads to enquire about possible sources of shortening underestimation. We argue that there is little degree of freedom to increase the values of total shortening by a significant amount. Vissers and Meijer (2012), Jammes et al. (2014) and Mouthereau et al. (2014) suggested a significant component of shortening (ranging between 300 km and 50–60 km) that left no signal as it was accommodated by subduction of a wide peridotite domain. This could have been the case as long as the sedimentary cover of that hyperextended domain was dragged down by peridotite and subducted into the mantle. However, we believe this is unlikely in the case of the studied section where the thick and buoyant sedimentary pile of the CBB and the Mauléon basin was detached and offscraped from the subducting peridotite. Hence, all the area loss by subduction must be necessarily recorded by thrusting detectable in balanced cross-sections.
The width of the exhumed peridotite domain in our reconstruction is a minimum value suggested by the occurrence of the lherzolite bodies. Enlarging the peridotite domain will represent adding more denudational gliding to the ancient continental margins, and subsequently more thrust displacement to the NPFT (which we have seen as problematic with the data available), or to the Licq and Lakora faults. However, the displacement of the Lakora thrust is constrained by hanging wall and footwall cutoffs (Teixell, 1993). A component of undetected south-directed thrusting may be envisaged for the Licq fault (in which the Triassic–Jurassic rocks define a hanging wall flat), but it is not possible to determine by how much with the preserved geometry. Our kinematic reconstruction already considers some 10 km of thrust displacement along that fault, to bring the Jurassic–Cretaceous section in contact with the northern border of the Iguntze-Mendibelza massifs (Fig. 4). Our detailed mapping in the CBB does not support the existence of large thrusts as proposed by Tugend et al. (2014). Moreover, the most prominent cleavage in the CBB is bedding-parallel and appears to predate folding, and although oblique cleavage is observed at certain localities, it is not regionally penetrative to account for large shortening. Slaty cleavage is penetrative in the southern Axial Zone and northern Jaca basin, and a component of shortening is underestimated there, although balanced cross-sections in the area used competent stratigraphic units (Labaume et al., 1985; Teixell, 1996) to minimize the uncertainty. Cross-sections in the Aquitaine basin by Biteau et al. (2006) show that inversion of extensional faults and salt structures north of the NPFT caused shortening not exceeding 5–6 km. The origin of this shortening by inversion of underlying basement faults or by displacement transfer from the NPFT remains unclear. Added to the shortening calculated for the Pyrenean thrust belt, a total amount of ca. 120 km could be admitted for the Pyrenees-Aquitaine basin system. Hence, if we are to follow the convergence magnitude indicated by the published plate reconstructions, much of the extra shortening needed should be probably searched out of the Pyrenees and their close forelands.
Our conservative reconstruction implies a slow shortening rate at the beginning of convergence during the Late Cretaceous and Paleocene (< 1 mm/a). This contrasts with the rapid rate proposed by Mouthereau et al. (2014) for the same period and process (3 mm/a), which would slow down in the Tertiary. Initial shortening in the Pyrenees was likely driven by far-field forces (the push from the South Atlantic) and not by negative buoyancy of the exhumed mantle domain (i.e. slab pull), which was actually hotter than normal, so we do not expect high rates at the beginning of convergence. Calculated shortening rate accelerated to ca. 3 mm/a during the Early and Mid Eocene, and slowed down to ca. 2.5 mm/a during the Late Eocene to the Early Miocene (Fig. 4).
6 Conclusions
The major events in the Pyrenean mountain building, from the Mid-Cretaceous hyperextension of margins and mantle exhumation to the late Cretaceous–Cenozoic subduction and collision, are reassessed on the basis of a new structural transect of the west-central Pyrenees. The recognition of the ancient continental margins of Iberia and Europe in the present-day cross-section allows identifying a crustal suture at the deep ramp of the North-Pyrenean Frontal thrust, by which the Iberian margin, now represented by the North-Pyrenean Zone basement, overrode the European margin towards the north during an early stage of collision.
Our proposed reconstruction to pre-orogenic times shows narrow continental margins where much of the extension was accommodated by distributed deformation in a large-scale boudinage-like structure. Tilted fault blocks were not dominant tectonic elements in the stretched margins. The sedimentary cover sequences were detached at the level of the upper Triassic and constituted a floating, continuous lid that glided down the margins into the peridotite domain. The cover was internally deformed by diapiric salt anticlines without detectable rafts or extensional rollovers. Extension was accommodated by wholesale gliding and a component of ductile stretching.
Sequential restoration illustrates that initial stages of convergence during the late Cretaceous and the earliest Tertiary involved subduction of the exhumed mantle domain and the pop-up expulsion of the sedimentary lid above. After the exhumed peridotite domain was closed by subduction, a protocollision stage involved the overriding of the Iberian margin on the European margin, with continued thrusting of the lid (Lakora and North-Pyrenean Frontal thrusts). This probably started in Early Eocene times, and was associated with flysch sedimentation in the southern foreland basin, recording a progressive basin infill until the late–Mid Eocene. From late–Mid/Late Eocene times, we propose that collision of the lower crust of the two plates promoted thrust accretion of the Iberian plate, causing major topographic emergence and the onset of typical collisional molasse sedimentation on both sides of the belt. The Iberian lower crust was decoupled and subducted to the north, while the European plate was indented into the decoupling boundary. Comparison of the present-day and restored sections provides an estimate of shortening of 114 km, which includes the subduction of the exhumed peridotite domain. The total shortening value can be enlarged to ca. 120 km if we take into account the sparse compressional structures in the Aquitaine foreland.
Acknowledgements
This work was supported by the ANR (France) PYRAMID project and by projects CGL2010-15416 and CGL2014-54180-P of the MINECO (Spain). We thank the critical revisions by the reviewers R. Huismans and F. Roure and by the editor M. Godard, who helped us to clarify specific points and to improve the original manuscript.