[Modélisation de processus physiques de plantes supérieures à l’aide de répliques de feuilles pour des applications spatiales]
À l’avenir, la culture de plantes supérieures sera un élément clé des systèmes de support de vie biorégénératifs. Cela nécessitera une compréhension approfondie des phénomènes qui jouent un rôle important au cœur du métabolisme des plantes et de leur interaction avec l’environnement. Les plantes sont des organismes complexes qui doivent être étudiés à l’aide de répliques de feuilles. Ceci permet l’étude des phénomènes physiques à la surface des feuilles, sans interactions biochimiques ou biologiques, ni variabilité génétique. Pour évaluer l’influence de la gravité, il est nécessaire de développer des modèles mécanistes précis du comportement des plantes dans l’espace. Cet article de synthèse présente l’état de l’art des répliques foliaires et des phénomènes concomitants, pour une application spatiale.
In the future, higher plant cultivation will be a key component of Bioregenerative Life-Support Systems. This will require a deep understanding of phenomena that play an important role at the core of plant metabolism and of their interaction with the environment. Plants are complex organisms that must be studied with the use of leaf replicas. This enables the study of physical phenomena at the leaf surface, without biochemical or biological interactions nor genetic variability. To assess the influence of gravity, it is a necessary step to develop precise mechanistic models of plant behaviour in space. This review article presents the state-of-the-art of leaf replicas and concomitant phenomena, with a space gaze.
Révisé le :
Accepté le :
Première publication :
Publié le :
Mots-clés : Réplique foliaire, Transpiration, Bilan énergétique, Systèmes de support de vie, Échange de chaleur, Phénomène biophysique, Modélisation mécaniste
Joanna Kuzma 1, 2 ; Lucie Poulet 1 ; Jean-Pierre Fontaine 1 ; Claude-Gilles Dussap 1
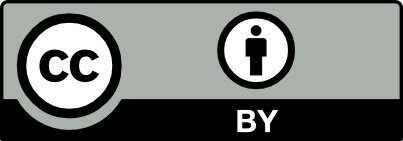
@article{CRMECA_2023__351_S2_97_0, author = {Joanna Kuzma and Lucie Poulet and Jean-Pierre Fontaine and Claude-Gilles Dussap}, title = {Modelling physical processes in higher plants using leaf replicas for space applications}, journal = {Comptes Rendus. M\'ecanique}, pages = {97--113}, publisher = {Acad\'emie des sciences, Paris}, volume = {351}, number = {S2}, year = {2023}, doi = {10.5802/crmeca.152}, language = {en}, }
TY - JOUR AU - Joanna Kuzma AU - Lucie Poulet AU - Jean-Pierre Fontaine AU - Claude-Gilles Dussap TI - Modelling physical processes in higher plants using leaf replicas for space applications JO - Comptes Rendus. Mécanique PY - 2023 SP - 97 EP - 113 VL - 351 IS - S2 PB - Académie des sciences, Paris DO - 10.5802/crmeca.152 LA - en ID - CRMECA_2023__351_S2_97_0 ER -
%0 Journal Article %A Joanna Kuzma %A Lucie Poulet %A Jean-Pierre Fontaine %A Claude-Gilles Dussap %T Modelling physical processes in higher plants using leaf replicas for space applications %J Comptes Rendus. Mécanique %D 2023 %P 97-113 %V 351 %N S2 %I Académie des sciences, Paris %R 10.5802/crmeca.152 %G en %F CRMECA_2023__351_S2_97_0
Joanna Kuzma; Lucie Poulet; Jean-Pierre Fontaine; Claude-Gilles Dussap. Modelling physical processes in higher plants using leaf replicas for space applications. Comptes Rendus. Mécanique, Physical Science in Microgravity within the Thematic Group Fundamental and Applied Microgravity, Volume 351 (2023) no. S2, pp. 97-113. doi : 10.5802/crmeca.152. https://comptes-rendus.academie-sciences.fr/mecanique/articles/10.5802/crmeca.152/
[1] Life Support Baseline Values and Assumptions Document, 2022 NASA/TP-2015218570/REV2, NASA JSC. (English only) https://ntrs.nasa.gov/citations/20210024855
[2] Fundamentals of life support systems, Spaceflight Life Support and Biospherics, Springer, Netherlands, Dordrecht, 1996, pp. 79-173 | DOI
[3] Agriculture for space: people and places paving the way, Open Agric., Volume 2 (2017), pp. 14-32 | DOI
[4] Selection of leafy green vegetable varieties for a pick-and-eat diet supplement on ISS, 45th International Conference on Environmental Systems ICES-2015-252, Bellevue, Washington, July (2015)
[5] Supplemental food production with plants: a review of NASA research, Front. Astron. Space Sci., Volume 8 (2021), 734343 | DOI
[6] Plants for human life support in space: from Myers to Mars, Gravitat. Space Res., Volume 23 (2010), pp. 25-36
[7] Gardening for therapeutic people-plant interactions during long-duration space missions, Open Agric., Volume 2 (2017), pp. 1-13 | DOI
[8] Spaceflight hardware for conducting plant growth experiments in space: The early years 1960–2000, Adv. Space Res., Volume 31 (2003), pp. 183-193 | DOI
[9] Growth chambers on the International Space Station for large plants, Acta Hortic., Volume 2 (2016) no. 1, pp. 215-222 | DOI
[10] Key gaps for enabling plant growth in future missions, AIAA SPACE and Astronautics Forum and Exposition, American Institute of Aeronautics and Astronautics, Orlando, FL, 2017 | DOI
[11] Plant biology for space exploration – Building on the past, preparing for the future, Life Sci. Space Res., Volume 29 (2021), pp. 1-7 | DOI
[12] Understanding reduced gravity effects on early plant development before attempting life-support farming in the Moon and Mars, Front. Astron. Space Sci., Volume 8 (2021), 729154 | DOI
[13] Large-scale crop production for the Moon and Mars: current gaps and future perspectives, Front. Astron. Space Sci., Volume 8 (2022), 733944 | DOI
[14] Plant’s response to space environment: a comprehensive review including mechanistic modelling for future space gardeners, Bot. Lett., Volume 163 (2016), pp. 337-347 | DOI
[15] The effect of gravity on surface temperature and net photosynthetic rate of plant leaves, Adv. Space Res., Volume 28 (2001), pp. 659-664 | DOI
[16] Important role of air convection for plant production in space farming, Biol. Sci. Space, Volume 24 (2010), pp. 121-128 | DOI
[17] The biophysical limitations in physiological transport and exchange in plants grown in microgravity, J. Plant Growth Regul., Volume 21 (2002), pp. 177-190 | DOI
[18] Farming in space: Environmental and biophysical concerns, Adv. Space Res., Volume 31 (2003), pp. 151-167 | DOI
[19] Air distribution in a fully-closed higher plant growth chamber impacts crop performance of hydroponically-grown lettuce, Front. Plant Sci., Volume 11 (2020), 537 | DOI
[20] Wind increases leaf water use efficiency, Plant Cell Environ., Volume 39 (2016), pp. 1448-1459 | DOI
[21] Spatial examination of leaf-boundary-layer conductance using artificial leaves for assessment of light airflow within a plant canopy under different controlled greenhouse conditions, Agric. For. Meteorol., Volume 280 (2020), 107773 | DOI
[22] Effects of air current speed on gas exchange in plant leaves and plant canopies, Adv. Space Res., Volume 31 (2003), pp. 177-182 | DOI
[23] Stomatal control and leaf thermal and hydraulic capacitances under rapid environmental fluctuations, PLoS One, Volume 8 (2013), e54231 | DOI
[24] Development of a mechanistic model of leaf surface gas exchange coupling mass and energy balances for life-support systems applications, Acta Astronaut., Volume 175 (2020), pp. 517-530 | DOI
[25] Radiometric method for determining canopy stomatal conductance in controlled environments, Agronomy, Volume 9 (2019), 114 | DOI
[26] Bridging the gap between leaf surface and the canopy air space: Leaf size matters for heat transfer resistance at canopy-scale, EGU General Assembly 2022, Vienna, Austria, 23–27 May 2022, EGU22-4268 (2022) | DOI
[27] Reducing the evaporative demand improves photosynthesis and water use efficiency of indoor cultivated lettuce, Agronomy, Volume 11 (2021), 1396 | DOI
[28] The competition between liquid and vapor transport in transpiring leaves, Plant Physiol., Volume 164 (2014), pp. 1741-1758 | DOI
[29] Thermography methods to assess stomatal behaviour in a dynamic environment, J. Exp. Bot., Volume 71 (2020), pp. 2329-2338 | DOI
[30] Technical note: An experimental set-up to measure latent and sensible heat fluxes from (artificial) plant leaves, Hydrol. Earth Syst. Sci., Volume 21 (2017), pp. 3377-3400 | DOI
[31] Dynamic leaf energy balance: deriving stomatal conductance from thermal imaging in a dynamic environment, J. Exp. Bot., Volume 70 (2019), pp. 2839-2855 | DOI
[32] The effect of gravity on surface temperatures of plant leaves: Effect of gravity on leaf temperatures, Plant Cell Environ., Volume 26 (2003), pp. 497-503 | DOI
[33] Effects of gravity on stem sap flow and water and heat exchange in the leaves of sweetpotato, Int. J. Microgravity Sci. Appl., Volume 35 (2018) no. 3, p. 350302-1–350302-6 | DOI
[34] The exchange of momentum, mass, and heat between an artificial leaf and the airflow in a wind-tunnel, Q. J. R. Meteorol. Soc., Volume 94 (1968), pp. 44-55 | DOI
[35] Boundary layer conductance of the leaves of some tropical timber trees, Plant Cell Environ., Volume 3 (1980), pp. 443-450 | DOI
[36] Heat transport coefficients for constant energy flux models of broad leaves, Bound.-Layer Meteorol., Volume 7 (1974), pp. 139-150 | DOI
[37] Effects of gravity on transpiration of plant leaves, Ann. N. Y. Acad. Sci., Volume 1161 (2009), pp. 166-172 | DOI
[38] Development of a simple thermal method for measuring sap flow in plants for space experiments, Biol. Sci. Space, Volume 32 (2018), pp. 17-21 | DOI
[39] Stomatal design principles in synthetic and real leaves, J. R. Soc. Interface, Volume 13 (2016), 20160535 | DOI
[40] Diffusion through stomates, Am. J. Bot., Volume 50 (1963), pp. 866-872 | DOI
[41] A study of transpiration from porous flat plates simulating plant leaves, Int. J. Heat Mass Transf., Volume 22 (1979), pp. 469-483 | DOI
[42] Leaf boundary layer conductance in ventilated greenhouses: An experimental approach, Agric. For. Meteorol., Volume 144 (2007), pp. 180-192 | DOI
[43] Boundary layer conductance for contrasting leaf shapes in a deciduous broadleaved forest canopy, Agric. For. Meteorol., Volume 139 (2006), pp. 40-54 | DOI
[44] A heated leaf replica technique for determination of leaf boundary layer conductance in the field, Agric. For. Meteorol., Volume 72 (1995), pp. 261-275 | DOI
[45] Buoyancy effect on forced convection in the leaf boundary layer, Plant Cell Environ., Volume 13 (1990), pp. 965-970 | DOI
[46] Effects of abiotic stress on plants: a systems biology perspective, BMC Plant Biol., Volume 11 (2011), 163 | DOI
[47] Rapid responses to abiotic stress: priming the landscape for the signal transduction network, Trends Plant Sci., Volume 24 (2019), pp. 25-37 | DOI
[48] Thriving under stress: How plants balance growth and the stress response, Dev. Cell, Volume 55 (2020), pp. 529-543 | DOI
[49] The number and size of the stomata, Bot. Gaz., Volume 46 (1908), pp. 221-224 | DOI
[50] Potatoes as a crop for space life support: effect of CO
[51] The plant’s energy balance, Plant Physiological Ecology, Springer, New York, NY, 2008, pp. 225-236 | DOI
[52] Plastic phenotypic response to light of 16 congeneric shrubs from a panamanian rainforest, Ecology, Volume 81 (2000), pp. 1925-1936 | DOI
[53] What is the minimal optimal sample size for plant ecophysiological studies?, Plant Physiol., Volume 178 (2018), pp. 953-955 | DOI
[54] Review and analysis of over 40 years of space plant growth systems, Life Sci. Space Res., Volume 10 (2016), pp. 1-16 | DOI
[55] How the optical properties of leaves modify the absorption and scattering of energy and enhance leaf functionality, Remote Sensing of Plant Biodiversity (J. Cavender-Bares; J. A. Gamon; P. A. Townsend, eds.), Springer International Publishing, Cham, 2020, pp. 349-384 | DOI
[56] A physical modeling approach for higher plant growth in reduced gravity environments, Astrobiology, Volume 18 (2018), pp. 1093-1100 | DOI
[57] Fundamentals of boundary-layer theory, Boundary-Layer Theory, Springer, Berlin, Heidelberg, 2017, pp. 29-49 | DOI
[58] Stomatal control of transpiration: scaling up from leaf to region, Advances in Ecological Research, Volume 15, Elsevier, 1986, pp. 1-49 | DOI
Cité par Sources :
Commentaires - Politique