[Introduction aux métamatériaux mécaniques et à leurs propriétés effectives]
Les métamatériaux sont des composites de conception rationnelle constitués de briques élémentaires qui sont composées d’un ou plusieurs matériaux constitutifs. Les propriétés des métamatériaux peuvent aller au-delà de celles des matériaux constitutifs, à la fois qualitativement et quantitativement. En outre, leurs propriétés peuvent être mises en correspondance avec certains modèles de milieux continus généralisés. Nous présentons une procédure générale de conception de métamatériaux élastiques à base de systèmes de type masses et de ressorts. Nous montrons quavec cette approche simple, nous pouvons concevoir tout un ensemble de propriétés effectives, y compris celles de métamatériaux élastiques non linéaires avec instabilités ou parties programmables — définis par un module de masse, de cisaillement et une masse volumique. Nous présentons des designs et calculs numériques afin dillustrer les lois de comportement. Enfin, nous discutons de l’apport d’un stimulus thermique aux métamatériaux mécaniques.
Metamaterials are rationally designed composites made of building blocks which are composed of one or more constituent materials. Metamaterial properties can go beyond those of the ingredient materials, both qualitatively and quantitatively. In addition, their properties can be mapped on some generalized continuum model. We present the general procedure of designing elastic metamaterials based on masses and springs. We show that using this simple approach we can design any set of effective properties including linear elastic metamaterials,—defined by bulk modulus, shear modulus, mass density—and nonlinear metamaterials,—with instabilities or programmable parts. We present designs and corresponding numerical calculations to illustrate their constitutive behavior. Finally, we discuss the addition of a thermal stimulus to mechanical metamaterials.
Publié le :
Mots-clés : Métamatériaux, Paramètres effectifs, Élasticité, Anisotropie, Ondes, Élasticité de Cauchy, Équation de Navier
Xueyan Chen 1, 2 ; Nicolas Laforge 1 ; Qingxiang Ji 1, 2 ; Huifeng Tan 2 ; Jun Liang 3, 2 ; Gwenn Ulliac 1 ; Johnny Moughames 1 ; Samia Adrar 1 ; Vincent Laude 1 ; Muamer Kadic 1
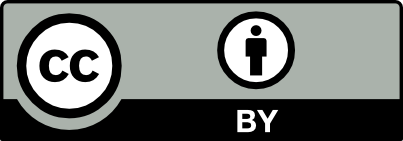
@article{CRPHYS_2020__21_7-8_751_0, author = {Xueyan Chen and Nicolas Laforge and Qingxiang Ji and Huifeng Tan and Jun Liang and Gwenn Ulliac and Johnny Moughames and Samia Adrar and Vincent Laude and Muamer Kadic}, title = {Introduction to mechanical metamaterials and their effective properties}, journal = {Comptes Rendus. Physique}, pages = {751--765}, publisher = {Acad\'emie des sciences, Paris}, volume = {21}, number = {7-8}, year = {2020}, doi = {10.5802/crphys.30}, language = {en}, }
TY - JOUR AU - Xueyan Chen AU - Nicolas Laforge AU - Qingxiang Ji AU - Huifeng Tan AU - Jun Liang AU - Gwenn Ulliac AU - Johnny Moughames AU - Samia Adrar AU - Vincent Laude AU - Muamer Kadic TI - Introduction to mechanical metamaterials and their effective properties JO - Comptes Rendus. Physique PY - 2020 SP - 751 EP - 765 VL - 21 IS - 7-8 PB - Académie des sciences, Paris DO - 10.5802/crphys.30 LA - en ID - CRPHYS_2020__21_7-8_751_0 ER -
%0 Journal Article %A Xueyan Chen %A Nicolas Laforge %A Qingxiang Ji %A Huifeng Tan %A Jun Liang %A Gwenn Ulliac %A Johnny Moughames %A Samia Adrar %A Vincent Laude %A Muamer Kadic %T Introduction to mechanical metamaterials and their effective properties %J Comptes Rendus. Physique %D 2020 %P 751-765 %V 21 %N 7-8 %I Académie des sciences, Paris %R 10.5802/crphys.30 %G en %F CRPHYS_2020__21_7-8_751_0
Xueyan Chen; Nicolas Laforge; Qingxiang Ji; Huifeng Tan; Jun Liang; Gwenn Ulliac; Johnny Moughames; Samia Adrar; Vincent Laude; Muamer Kadic. Introduction to mechanical metamaterials and their effective properties. Comptes Rendus. Physique, Metamaterials 2, Volume 21 (2020) no. 7-8, pp. 751-765. doi : 10.5802/crphys.30. https://comptes-rendus.academie-sciences.fr/physique/articles/10.5802/crphys.30/
[1] Honeycomb carbon: a review of graphene, Chem. Rev., Volume 110 (2009) no. 1, pp. 132-145 | DOI
[2] Graphene based electrochemical sensors and biosensors: a review, Electroanalysis, Volume 22 (2010) no. 10, pp. 1027-1036 | DOI
[3] A review on mechanical exfoliation for the scalable production of graphene, J. Mater. Chem. A, Volume 3 (2015) no. 22, pp. 11700-11715 | DOI
[4] A review on carbon nanotubes and graphene as fillers in reinforced polymer nanocomposites, J. Ind. Eng. Chem., Volume 21 (2015), pp. 11-25 | DOI
[5] Mechanical and tribological properties of self-lubricating metal matrix nanocomposites reinforced by carbon nanotubes (CNTs) and graphene–a review, Composites B, Volume 77 (2015), pp. 402-420 | DOI
[6] Energy absorption capability and crashworthiness of composite material structures: a review, Appl. Mech. Rev., Volume 51 (1998) no. 10, pp. 635-649 | DOI
[7] A review of mechanical drilling for composite laminates, Compos. Struct., Volume 94 (2012) no. 4, pp. 1265-1279 | DOI
[8] A review of recent research on mechanics of multifunctional composite materials and structures, Compos. Struct., Volume 92 (2010) no. 12, pp. 2793-2810 | DOI
[9] Mechanical metamaterials associated with stiffness, rigidity and compressibility: A brief review, Prog. Mater. Sci., Volume 94 (2018), pp. 114-173 | DOI
[10] Elastic metamaterials and dynamic homogenization: a review, Int. J. Smart Nano Mater., Volume 6 (2015) no. 1, pp. 41-60 | DOI
[11] Flexible mechanical metamaterials, Nat. Rev. Mater., Volume 2 (2017) no. 11, p. 17066 | DOI
[12] Micro-/nanostructured mechanical metamaterials, Adv. Mater., Volume 24 (2012) no. 36, pp. 4782-4810 | DOI
[13] Photonic band-gap structures, J. Opt. Soc. Am. B, Volume 10 (1993) no. 2, pp. 283-295 | DOI
[14] Photonic band structure: The face-centered-cubic case employing nonspherical atoms, Phys. Rev. Lett., Volume 67 (1991) no. 17, p. 2295 | DOI
[15] Special points for Brillouin-zone integrations, Phys. Rev. B, Volume 13 (1976) no. 12, p. 5188 | DOI | MR
[16] Improved tetrahedron method for Brillouin-zone integrations, Phys. Rev. B, Volume 49 (1994) no. 23, p. 16223 | DOI
[17] Elastodynamics (volume 1, finite motions), J. Appl. Mech., Volume 42 (1975), p. 748 | DOI
[18] Applications of an energy-momentum tensor in nonlinear elastodynamics: Pseudomomentum and eshelby stress in solitonic elastic systems, J. Mech. Phys. Solids, Volume 40 (1992) no. 7, pp. 1543-1558 | DOI | MR | Zbl
[19] Wave Propagation in Elastic Solids, Vol. 16, Elsevier, Amsterdam, The Netherlands, 2012
[20] On modifications of Newton’s second law and linear continuum elastodynamics, Proc. R. Soc. A, Volume 463 (2007) no. 2079, pp. 855-880 | DOI | MR | Zbl
[21] 3D metamaterials, Nat. Rev. Phys., Volume 1 (2019) no. 3, pp. 198-210 | DOI
[22] Vibrations of lattice structures and phononic band gaps, Q. J. Mech. Appl. Math., Volume 56 (2003) no. 1, pp. 45-64 | DOI | MR
[23] Dispersion and localization of elastic waves in materials with microstructure, Proc. R. Soc. A, Volume 467 (2011) no. 2134, pp. 2874-2895 | DOI | MR | Zbl
[24] Dispersion and localisation in structured Rayleigh beams, Int. J. Solids Struct., Volume 51 (2014) no. 25-26, pp. 4452-4461 | DOI
[25] Low-frequency dispersion and attenuation in partially saturated rocks, J. Acoust. Soc. Am., Volume 94 (1993) no. 1, pp. 359-370 | DOI
[26] Characteristics of mechanical metamaterials based on buckling elements, J. Mech. Phys. Solids, Volume 102 (2017), pp. 151-164 | DOI | MR
[27] Which elasticity tensors are realizable?, J. Eng. Mater. Technol., Volume 117 (1995) no. 4, pp. 483-493 | DOI
[28] An Introduction to Metamaterials and Waves in Composites, CRC Press, Boca Raton, Florida, USA, 2011 | DOI
[29] On the practicability of pentamode mechanical metamaterials, Appl. Phys. Lett., Volume 100 (2012) no. 19, 191901 | DOI
[30] Metamaterials beyond electromagnetism, Rep. Prog. Phys., Volume 76 (2013) no. 12, 126501 | DOI
[31] On three-dimensional dilational elastic metamaterials, New J. Phys., Volume 16 (2014) no. 3, 033032 | DOI
[32] Tailored 3D mechanical metamaterials made by dip-in direct-laser-writing optical lithography, Adv. Mater., Volume 24 (2012) no. 20, pp. 2710-2714 | DOI
[33] Three-dimensional mechanical metamaterials with a twist, Science, Volume 358 (2017) no. 6366, pp. 1072-1074 | DOI
[34] New twists of 3D chiral metamaterials, Adv. Mater., Volume 31 (2019) no. 26, 1807742 | DOI
[35] Compressive behaviour of stainless steel micro-lattice structures, Int. J. Mech. Sci., Volume 68 (2013), pp. 125-139 | DOI
[36] Elastically-isotropic truss lattice materials of reduced plastic anisotropy, Int. J. Solids Struct., Volume 138 (2018), pp. 24-39 | DOI
[37] Stiffness and specific energy absorption of additively-manufactured metallic bcc metamaterials composed of tapered beams, Int. J. Mech. Sci., Volume 141 (2018), pp. 101-116 | DOI
[38] Effective properties of the octet-truss lattice material, J. Mech. Phys. Solids, Volume 49 (2001) no. 8, pp. 1747-1769 | DOI | Zbl
[39] Tailored buckling microlattices as reusable light-weight shock absorbers, Adv. Mater., Volume 28 (2016) no. 28, pp. 5865-5870 | DOI
[40] Additively-manufactured metallic micro-lattice materials for high specific energy absorption under static and dynamic loading, Acta Mater., Volume 116 (2016), pp. 14-28 | DOI
[41] Mechanical properties of an improved 3D-printed rhombic dodecahedron stainless steel lattice structure of variable cross section, Int. J. Mech. Sci., Volume 145 (2018), pp. 53-63 | DOI
[42] A new type of low density material: Shellular, Adv. Mater., Volume 27 (2015) no. 37, pp. 5506-5511 | DOI
[43] Smooth-shell metamaterials of cubic symmetry: Anisotropic elasticity, yield strength and specific energy absorption, Acta Mater., Volume 164 (2019), pp. 301-321 | DOI
[44] Programmable mechanical metamaterials, Phys. Rev. Lett., Volume 113 (2014) no. 17, 175503 | DOI
[45] Cellular Solids: Structure and Properties, Cambridge University Press, Cambridge, UK, 1999
[46] 3D plate-lattices: An emerging class of low-density metamaterial exhibiting optimal isotropic stiffness, Adv. Mater., Volume 30 (2018) no. 45, 1803334
[47] Mechanical metamaterials at the theoretical limit of isotropic elastic stiffness, Nature, Volume 543 (2017) no. 7646, p. 533 | DOI
[48] Foam topology: bending versus stretching dominated architectures, Acta Mater., Volume 49 (2001) no. 6, pp. 1035-1040 | DOI
[49] Viscoelastic Solids, Vol. 9, CRC Press, Boca Raton, Florida, USA, 1998
[50] Theory of Viscoelasticity: An Introduction, Academic Press Inc., New York, USA, 2012
[51] Elasticity and anelasticity of metals, J. Phys. Chem., Volume 53 (1949) no. 9, p. 1468-1468 | DOI
[52] Light-weight shell-lattice metamaterials for mechanical shock absorption, Int. J. Mech. Sci., Volume 169 (2020), 105288 | DOI
[53] Energy Absorption of Structures and Materials, Woodhead Publishing Limited, Cambridge, UK, 2003
[54] Energy dissipation mechanisms in hollow metallic microlattices, J. Mater. Res., Volume 29 (2014) no. 16, pp. 1755-1770 | DOI
[55] Strong, lightweight, and recoverable three-dimensional ceramic nanolattices, Science, Volume 345 (2014) no. 6202, pp. 1322-1326 | DOI
[56] Energy absorption of thin-walled square tubes with a prefolded origami pattern part I: geometry and numerical simulation, J. Appl. Mech., Volume 81 (2014) no. 1, 011003
[57] Architected origami materials: How folding creates sophisticated mechanical properties, Adv. Mater., Volume 31 (2019) no. 5, 1805282
[58] Ultralight metallic microlattices, Science, Volume 334 (2011) no. 6058, pp. 962-965 | DOI
[59] Using origami design principles to fold reprogrammable mechanical metamaterials, Science, Volume 345 (2014) no. 6197, pp. 647-650 | DOI
[60] Multistable architected materials for trapping elastic strain energy, Adv. Mater., Volume 27 (2015) no. 29, pp. 4296-4301 | DOI
[61] Harnessing instabilities to design tunable architected cellular materials, Annu. Rev. Mater. Res., Volume 47 (2017), pp. 51-61 | DOI
[62] Negative thermal expansion in transversely isotropic space frame trusses, Phys. Status Solidi B, Volume 250 (2013) no. 10, pp. 2062-2069 | DOI
[63] Spacecraft Thermal Control Handbook, Fundamental Technologies, vol. 1, American Institute of Aeronautics and Astronautics, Reston, Virginia, USA, 2002, pp. 373-403 (Online version available at: http://www.knovel.com/knovel2/Toc.jsp)
[64] Origami and kirigami inspired self-folding for programming three-dimensional shape shifting of polymer sheets with light, Extreme Mech. Lett., Volume 11 (2017), pp. 111-120 | DOI
[65] 3D printed reversible shape changing components with stimuli responsive materials, Sci. Rep., Volume 6 (2016), p. 24761 | DOI
[66] Designing microstructural architectures with thermally actuated properties using freedom, actuation, and constraint topologies, J. Mech. Design, Volume 135 (2013) no. 6, 061004
[67] Design to self-assembly, Archit. Design, Volume 82 (2012) no. 2, pp. 68-73 | DOI
[68] Self-folding thermo-magnetically responsive soft microgrippers, ACS Appl. Mater. Interfaces, Volume 7 (2015) no. 5, pp. 3398-3405 | DOI
[69] Self-folding all-polymer thermoresponsive microcapsules, Soft Matter, Volume 7 (2011) no. 7, pp. 3277-3279 | DOI
[70] Dense solid microstructures with unbounded thermal expansion, J. Mech. Behav. Mater., Volume 7 (1996) no. 2, pp. 85-92 | DOI
[71] Stiff, strong, zero thermal expansion lattices via material hierarchy, Compos. Struct., Volume 107 (2014), pp. 654-663 | DOI
[72] Lightweight mechanical metamaterials with tunable negative thermal expansion, Phys. Rev. Lett., Volume 117 (2016) no. 17, 175901 | DOI
[73] Concepts for structurally robust materials that combine low thermal expansion with high stiffness, J. Mech. Phys. Solids, Volume 55 (2007) no. 9, pp. 1803-1822 | DOI | MR | Zbl
[74] Tailorable thermal expansion hybrid structures, Int. J. Solids Struct., Volume 46 (2009) no. 11-12, pp. 2372-2387 | DOI | Zbl
[75] Composites with extremal thermal expansion coefficients, Appl. Phys. Lett., Volume 69 (1996) no. 21, pp. 3203-3205 | DOI
[76] Design of materials with extreme thermal expansion using a three-phase topology optimization method, J. Mech. Phys. Solids, Volume 45 (1997) no. 6, pp. 1037-1067 | DOI | MR
[77] Optimality of thermal expansion bounds in three dimensions, Extreme Mech. Lett., Volume 12 (2017), pp. 97-100 | DOI
[78] Poroelastic metamaterials with negative effective static compressibility, Appl. Phys. Lett., Volume 110 (2017) no. 17, 171901
[79] Micro-structured two-component 3D metamaterials with negative thermal-expansion coefficient from positive constituents, Sci. Rep., Volume 7 (2017), p. 40643 | DOI
- Cellular metastructures for elastic wave attenuation using chaotic soft-walled billiard effects, Nonlinear Dynamics (2025) | DOI:10.1007/s11071-025-10931-9
- Nonlinear dynamics analysis of origami structures based on the bar hinge model, Structures, Volume 71 (2025), p. 108088 | DOI:10.1016/j.istruc.2024.108088
- Failure mechanisms of anisotropic pentamode-based bridge bearings: A dynamic analysis, Engineering Structures, Volume 301 (2024), p. 117292 | DOI:10.1016/j.engstruct.2023.117292
- Mechanical metamaterials, Reports on Progress in Physics, Volume 86 (2023) no. 9, p. 094501 | DOI:10.1088/1361-6633/ace069
Cité par 4 documents. Sources : Crossref
Commentaires - Politique