Now that the concepts of stem cell biology are not only providing a useful theoretical basis for studies of cellular differentiation, but are also starting to provide paradigms underpinning emerging ideas for cellular therapies, it is a worthwhile exercise to re-examine the source and nature of Embryonic Stem cells which were first isolated from mouse embryos over twenty years ago 〚1〛.
Clearly embryonic development starting from a single zygote proceeds through extensive cell proliferation and progressive cellular differentiation. At early stages, there are cells with wide prospective fates but these are not necessarily self-renewing populations. Pluripotential stem cells were first experimentally identified in the mouse as the stem cell of teratocarcinomas by Kleinsmith and Pierce, who were able to passage the tumour by transfer of single cells isolated from embryoid bodies 〚2〛. Teratocarcinomas are tumours that arise from the gonads and, in contrast to most other neoplasms, contain a wide diversity of types of tissue and the passaged tumours were each able to produce the full range. Mouse testicular teratocarcinomas arose spontaneously in a specific inbred mouse strain (129) developed by LeRoy Stevens and his extensive studies showed that they arose by a proliferation of germ cells in the male genital ridge 〚3〛. Transplantation of an 11.5–12.5-day germinal ridge to the ectopic site of an adult testis resulted in teratocarcinoma formation from the foetal germ cells 〚4〛. At this stage of development, only the germ cells have a multipotential prospective fate and the tumour development could well have been explained by their progression through a spontaneous parthenogenetic activation and embryonic development. Stevens saw histologically, however, only a smooth progression to progressive growth of nests of teratocarcinoma stem cells – termed embryonal carcinoma (EC) cells. The alternative idea, that teratocarcinomas are embryo rather than germ cell derived was tested directly by transplanting early embryos to adult testes or kidneys 〚5〛. Passageable progressively growing teratocarcinomas were formed after transplantation of 1–3.5-day embryos. After gastrulation, an embryonic mesoderm has formed by invagination from the ectoderm and lies between the ectoderm and an embryonic endoderm. Nicola Skreb and his colleagues tested the developmental potency of these three layers in the rat embryo that they isolated by microdissection by ectopic transplantation. Only the embryonic ectoderm gave rise to teratomas with multiple tissue types and was hence pluripotential, but these were not progressively growing teratocarcinomas. Solter repeated these experiments with mouse gastrulating embryos and discovered that, in contrast to the rat, transplantable teratocarcinomas were formed. He therefore defined that at least in the mouse pluripotential stem cells could be recovered from stages up to about the end of gastrulation 〚6〛.
Using a transplantable teratocarcinoma that had been generated from a preimplantation embryo by Stevens, Evans was able to derive clonally isolated cell cultures that demonstrated their pluripotentiality by forming well-differentiated teratocarcinomas upon re-injection into mice 〚7〛. These also differentiated extremely well in vitro by an embryo-like route 〚8, 9〛. A series of observations of the properties of these and similar EC (embryonal carcinoma) cells started to provide convincing evidence of their homology with the pluripotential cells in the normal embryo, which were able to give rise to teratocarcinomas under conditions of ectopic transplantation. There seemed to be every reason to suppose that direct isolation into tissue culture should be possible 〚10〛.
The culture conditions – feeder cells and media – had been refined by culturing both mouse and human teratocarcinoma EC cells. Evans and Kaufman, by using implantationally delayed blastocysts as the embryo source, succeeded in establishing cultures of pluripotential cells directly without an in vivo tumour step 〚11〛. Subsequent studies showed isolation of such cell lines from numbers of both inbred and outbred strains and from normal 3.5-day blastocysts as well as those which had been implantationally delayed. Isolated 3.5-day ICMs may also be used 〚12〛. These cells, which have become known as Embryonic Stem Cells, share the properties of indefinite proliferative capacity, embryonic phenotype and differentiative capacity with their forerunners the EC cells but, in addition, being primarily derived, may be kept entirely karyotypically normal. This has allowed their use as vectors between tissue-culture genetic manipulation and selection and the mouse germline via chimaeric mice and is of major importance in providing a direct experimental mammalian genetics.
Eistetter 〚13〛 reported that ES cell cultures might be established from disaggregated 16–21-cell morulae with an apparent immediate growth of the colonies from some of the explanted single cells. Up to four separate ES cell colonies were founded from a single embryo.
The latest stage at which isolation into culture of ES cells from embryos has been reported is by Brook and Gardner, who used 4.5 day-old hatched, peri-implantational embryos flushed from the uterus 〚14〛. They microdissected the epiblast cells away from both the primary endoderm and the trophectoderm. Both whole epiblasts and those that had been disaggregated into single cells readily gave rise to ES cultures, but not if left in contact with endoderm. They also reconfirmed the benefit of using delayed blastocysts, showing that the isolated epiblasts of these were the most efficient source of ES cell cultures.
These epiblasts of 4.5-day embryos are at a stage before formation of the epithelial sheet of definitive embryonic ectoderm. It is noticeable that explantation of 6-day embryonic ectoderm (now in an epithelium) into tissue culture under suitable conditions does not result in ES cell cultures. It was noted by Evans 〚10〛 that the phenotype of the 6-day and older embryonic ectoderm is entirely different from EC cells, despite the ready formation of teratocarcinomas from this stage, and he speculated that, under the in vivo conditions of ectopic transplantation, there might be a de-differentiation.
Nowadays, the dogmas of irreversibility of cellular differentiation are not necessarily so strongly believed and there is, indeed, an interesting phenomenon reported in vitro, which supports reversibility of cellular commitment at this stage of development. Rathgen et al. 〚15, 16〛 discovered that conditioned medium from the Hep G2 human hepatocellular carcinoma cell line contained factors that induced ES cells in culture to change into another distinct cell type. The cell colony morphology is changed from the typical piling clump of mouse ES cells to a more flattened monolayer form with more distinct cell outlines. This distinct cell type was called EPL (early primitive ectoderm-like), because of the conclusion drawn from their studies that this cell type more closely resembles early ectoderm. This is supported by their analysis of gene expression (Fig. 1). These cells are still pluripotential and show extensive differentiation in tissue culture. Their embryoid body differentiation shows a slightly different course of development than those from ES cells, being more precocious in ectoderm formation and with a reduced visceral endoderm 〚17〛.
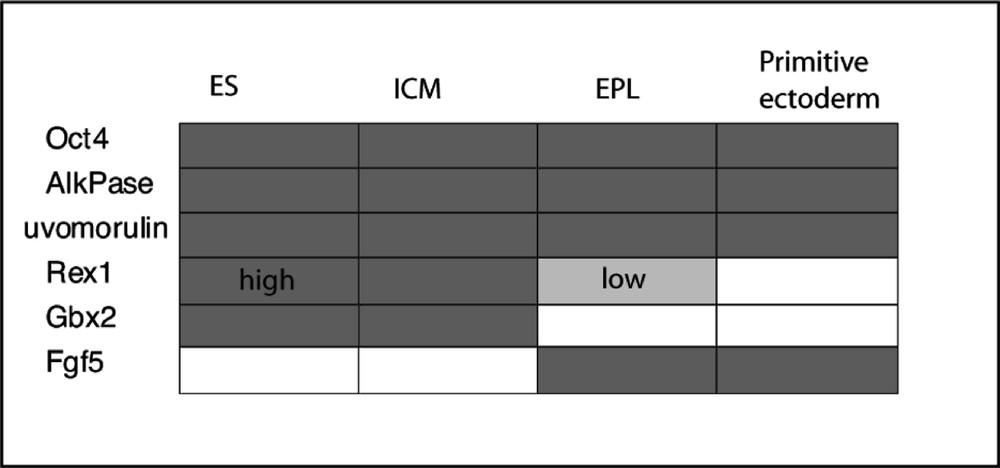
Expression patterns of ES and EPL cells compared with that of ICM and primitive ectoderm. After Rathgen et al. 〚14〛.
In contrast to ES cells EPL cells do not readily colonise blastocysts to form chimaeric mice. They can be indefinitely maintained in passage culture in the presence of 50% MEDII-conditioned medium without LIF. Interestingly, however, these cells revert to ES cells after withdrawal of the MEDII factors and in the presence of LIF. These studies strongly suggest that ES cells are not yet primitive ectoderm, but a precursor. They also support the suggestion that, under suitable circumstances, a de-differentiation from an ectodermal cell to an ES cell may take place, thus providing an explanation for the origin of teratocarcinomas from post 4.5-day embryos and the failure to directly isolate ES cells from embryonic ectoderm.
It is only from the mouse that fully characterised ES cells have been obtained, but cultures of cells that have some of the expected properties have been isolated from numbers of other species. Only the hamster cells 〚17〛 (another rodent) are the morphologically similar. The early development of many mammals is similar up to the blastocyst stage, but in most, unlike the mouse and rat, the ICM of the blastocyst develops into an embryonic disc with a clearly epithelialised embryonic ectoderm. It may be for this reason that cell lines isolated from bovine, porcine and ovine embryos 〚18〛 grow more in a monolayer than the typical mouse ES cell clumps (Fig. 2). It is noticeable too that the descriptions of human ES cells are similar 〚19〛. For reasons either of practicability or of ethics, it has not been possible to demonstrate that the putative ES cells from any of these species is able to form germ-line chimaeras. In some cases, particularly for human ES cells, their differentiation both in vivo in xenotransplants and in vitro via embryoid bodies and explanted monolayer culture is similar to mouse ES cells and is the best evidence for their status. Note, however, that the mouse EPL cells were able to go extensive in vitro differentiation but were noticeably different in their embryoid body behaviour.
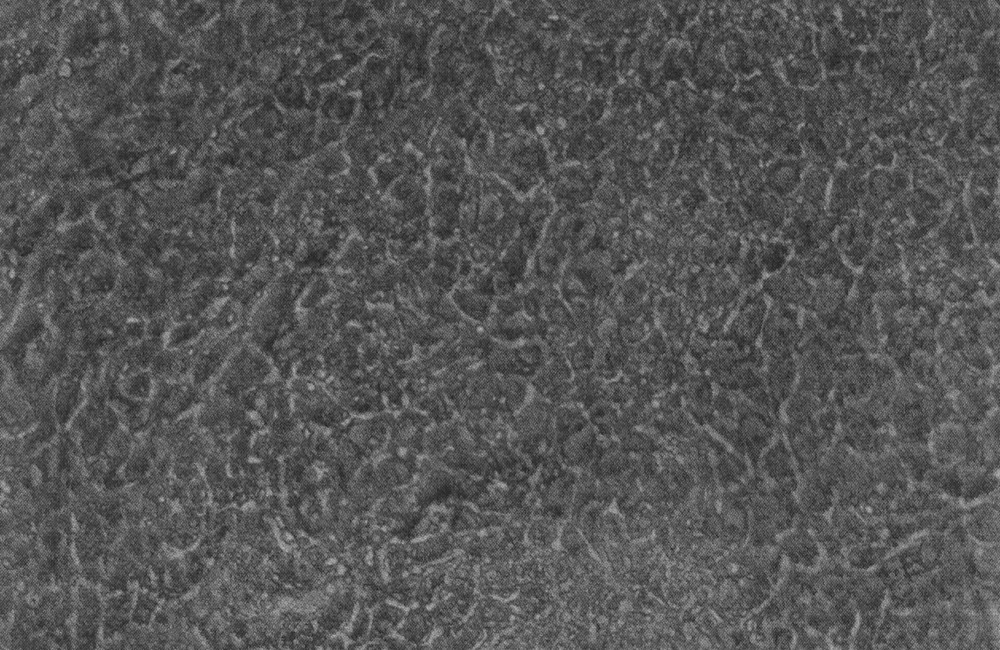
Putative ES cells isolated by explantation of a bovine blastocyst.
Of mammalian species from which efforts have been made to isolate ES cells, the rat is an exception in as much as the early development of its cells is very similar to that of mouse cells. Despite a great deal of effort, it has never been shown that ‘gold-standard’ ES cell lines have ever been derived from the rat. Many groups have produced lines that share some of the characteristics of mouse lines; they express the cell surface marker SSEA1, alkaline phosphatase, Oct4; our studies show that they will form teratomas when placed under the kidney capsule of nude host mice. Cells lines grown from these tumours include beating muscle and neurons. It is noticeable that some lines show both Oct4 and Rex1 (typical of ES cells) but others show only Oct4 as reported for EPL lines (Fig. 3). Morphologically, these cells have a looser, more rounded phenotype than the mouse. The cell lines are, however, very difficult to maintain beyond 20 passages and at no time have chimaeras been obtained. This is not, as in the hamster, due to difficulties in the establishment of pseudopregnancy and the transfer and subsequent development of the manipulated preimplantation embryos. All these techniques are well established for the rat and rates of development of control or injected blastocysts are high.
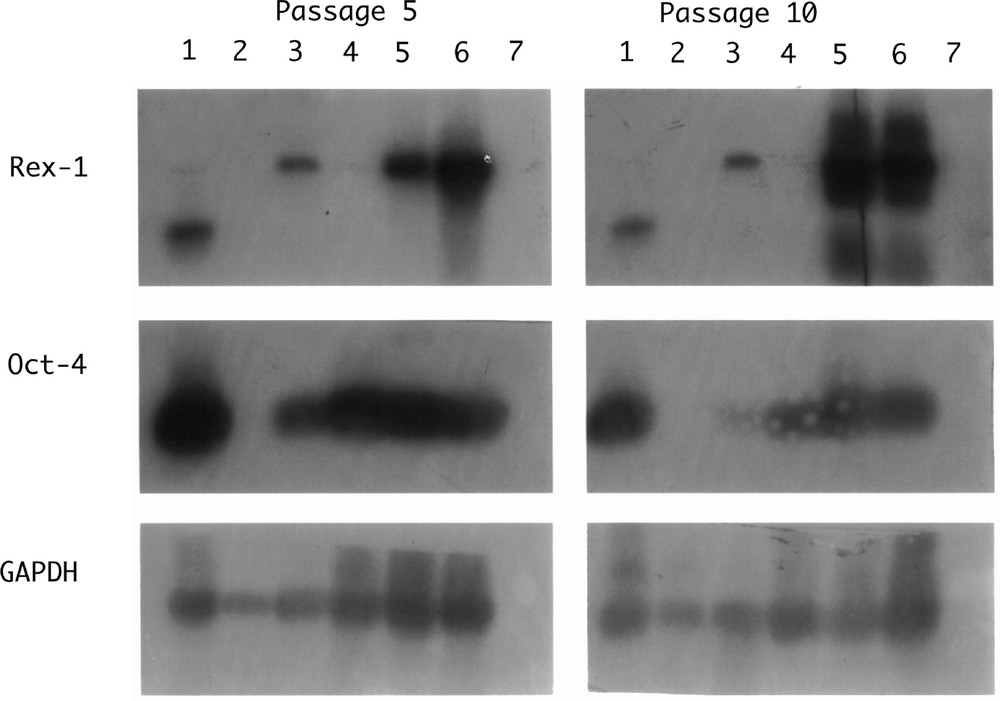
Southern Blot hybridisation of RT–PCR products from rat cell lines. Lane 1: IMT11 (mouse ES cell control); lane 2: rat fibroblasts; lane 3: RL-1; lane 4: RL-2; lane 5: RL-5; lane 6: RL-6; lane 7: no RNA control.
One of the questions to ask is whether mouse ES cells represent a cell type normally found in the early embryo or whether they are effectively an artefact of culture? In the normal embryo, the cells are entrained in a developmental time course, but in culture, the developmental time has stopped. It would seem likely that the stem cell behaviour of unlimited regenerative capacity of the stem cell population should have had its counterpart in the normal embryo. The most likely source would therefore seem to be the epiblast of the 4.5–5.5-day embryo. At this time, there can be extremely rapid cell cycles and catch-up growth size regulation. This speculation fits with the observations of Brook and Gardner as the embryo stage for efficient ES cell isolation and also with those of Rathgen et al. that ES cells are different from the ectodermal epithelium. Nevertheless, it is not unlikely that ES cells in culture, in an environment and medium quite dissimilar to that within the normal developing embryo and cut off from developmental signals, are off the normal pathway, but can readily revert onto it.
One of the important lines of evidence that were used to compare EC cells with early embryo cell lineages was the 2d gel studies of nascently labelled proteins of Lovell-Badge 〚20〛 – this approach of using as wide a display of gene action as possible to define cellular phenotype was effectively a proteomics approach, but without the modern downstream postgenomic information. Lovell-Badge’s interesting conclusions were that the ICM and EC cells did not match well, but that the best match for EC cells was found in the 5-day embryo ectoderm, that is an uncavitated ectodermal cell mass prior to epithelial formation. Interestingly, too – especially in the light of the subsequent studies that demonstrated isolation of pluripotential (EG) cells 〚21〛 – was the observation that primordial germ cells from the 11.5-day germinal ridge matched well, except for the absence of a specific set of polypeptides. In this postgenomic era, tools have now become available to readily identify polypeptides separated on such gels to their loci of origin and to investigate genome-wide transcription patterns. Rapid and sensitive microarray analysis will provide the tool to identify ES cell transcriptional phenotypes and follow the transcriptional changes underlying cellular differentiation. Such studies should, moreover, allow comparisons between ES cell and normal embryo tissues and answer some of the questions raised above. Contrast and comparison between cell lines both within and between species should also allow an approach to identification and validation of cell lines where direct test of pluripotentiality and normality is otherwise difficult. If present ideas of using ES cells as the basis of cellular therapies become realisable, such quality control will become necessary criteria of acceptability.