Version française abrégée
Les réserves marines ont fait l'objet de nombreuses études, basées sur la comparaison de la biomasse et de la densité d'espèces cibles entre des zones protégées et non protégées. Les poissons, de par leur intérêt commercial, ont souvent fait l'objet de ce type d'étude à l'aide de méthodes non destructrices, comme le comptage visuel. Les sparidés sont très répandus dans la Méditerranée nord-occidentale et constituent une ressource importante d'un point de vue commercial pour les pêcheries côtières. Le sar commun constitue, avec le sar à tête noire, Diplodus vulgaris (Saint-Hilaire, 1817) une des espèces les plus abondantes de l'infralittoral rocheux et de l'herbier de posidonies. Il fait partie du groupe des poissons nectobenthiques mésophages, caractérisés par des déplacements verticaux réduits et un potentiel de mouvements horizontaux important. Les adultes sont hermaphrodites protandres. Les individus sont mâles à leur première maturité sexuelle (environ 3 ans) et deviennent femelles vers l'âge de 5 ans. La reproduction a lieu au printemps, avec un maximum en avril sur les côtes catalanes françaises. Les zones de pontes ne sont pas précisément connues et semblent être différentes de l'habitat adulte. Après un mois de durée de vie larvaire pélagique, les larves recrutent sur des petits fonds, où elles y restent plusieurs mois. Au-delà de 5 cm de longueur, les juvéniles quittent les zones de nurseries pour intégrer la population adulte. L'objectif de cette étude était de décrire les structures démographiques et génétiques des populations de sar commun et d'évaluer l'impact de la protection sur les populations de la réserve marine de Cerbère–Banyuls, située sur la côte catalane française en Méditerranée nord-occidentale.
Les échantillons de sars communs ont été collectés dans deux sites des côtes méditerranéennes françaises proches de l'Espagne. Le premier site est localisé dans la réserve marine de Cerbère–Banyuls (650 ha), créée en 1974, et qui est constituée de deux zones : une zone partiellement protégée (585 ha) (PPA) et une zone totalement protégée (65 ha) (TPA). La législation dans la réserve autorise la pêche récréative à la ligne, mais réglemente l'activité de pêche artisanale (cinq à huit autorisations par an). La chasse sous-marine est strictement interdite. Dans la TPA, toutes les activités sont interdites, à l'exception des plongées scientifiques. Aucun poisson n'a été capturé dans cette zone, faute d'autorisation. Le site choisi dans la réserve (PPA) est situé à proximité d'un cap, sur des fonds rocheux de 20 à 30 m de profondeur. Le second site d'étude est situé à l'extérieur de la réserve (UPA). Il a été sélectionné dans une zone (cap Béar), dont les caractéristiques physiques sont proches de celles du site PPA et où la pression de pêche est importante. Les pêcheurs professionnels utilisent les mêmes techniques que celles employées dans la PPA. La pêche à la ligne amateur et la chasse sous-marine sont des activités courantes sur cette côte rocheuse. Depuis plusieurs années, un championnat national de chasse sous-marine est organisé chaque année sur cette zone. Les deux sites d'étude sont séparés d'environ 5 km. Dans le cadre de notre échantillonnage, tous les poissons ont été capturés de nuit au fusil harpon, entre février et mai 1996. Le temps de plongée et la profondeur ont été standardisés dans les deux sites. Chaque poisson a été mesuré (longueur standard), sexé puis disséqué pour extraire les otolithes (sagitta), le foie et un morceau de muscle. Les otolithes ont été inclus dans la résine, poncés et colorés au bleu de toluidine pour visualiser les stries annuelles. La validation de l'estimation de l'âge a été réalisée par deux méthodes (évolution de l'accroissement marginal et injection de tétracycline). Les échantillons ont été analysés par électrophorèse sur gels d'amidon horizontaux et gels d'acétate. Les fréquences alléliques et les F-statistics (FIS et FST) ont été calculés à partir du logiciel GENETIX 4.0. La « significativité » de l'écart à l'équilibre de Hardy–Weinberg et de la différenciation génétique a été évaluée par les tests exacts de Fisher (GENEPOP 3.1d).
La longueur standard des individus varie de 13,1 à 29,2 cm dans le site non protégé (UPA : 18,4−SE=3,7−n=61) et de 15,3 à 27,0 cm dans la réserve (PPA : 19,6−SE=2,6−n=70). La distribution des âges montre que les poissons sont nés entre 1988 et 1993 (respectivement des cohortes de huit à trois ans). Les distributions de la longueur standard et de l'âge sont unimodales dans les deux sites. Les individus sont significativement plus grand et plus vieux dans la réserve (tests de Mann–Whitney, longueur standard : Z=−3,51−p<0,001 ; âge : Z=−4,39−p<0,001). De plus, les femelles sont plus nombreuses que les mâles dans les deux sites, mais la distribution des femelles en fonction de l'âge est différente au sein et à l'extérieur de la réserve (Pearson χ2 ; femelles : χ2=21,37−p=0,001 ; mâles : χ2=1,16−p=0,88). Le mode de distribution des femelles est centré sur les cohortes de quatre (1992) et six ans (1990), respectivement pour les sites UPA et PPA. La cohorte 4 ans, qui représente plus de 30 % dans l'UPA, est quasiment absente dans la réserve (PPA). Inversement, les cohortes 6 ans et 7 ans sont proportionnellement plus faibles à l'extérieur de la réserve. Les analyses génétiques ont porté sur 26 locus analysés dont 16 sont apparus polymorphes à 99 % (13 locus polymorphes à 95 %). Les principaux paramètres étudiés montrent de faibles variations entre les deux sites. Aucun écart à l'équilibre de Hardy–Weinberg n'a été observé dans les deux sites. La valeur de FST multilocus (0,007−p=0,04) est significative et est essentiellement due à un seul locus (GDA∗ : FST=0,054−p=0,0003). Tous les autres locus génèrent des valeurs de FST non significatives.
La structure démographique et la distribution du sexe en fonction de l'âge chez le sar commun sont affectées par la protection offerte par la réserve marine. La structure génétique des individus des deux sites montre une différenciation génétique induite par un seul locus. Cependant, ceci ne semble pas suffisant pour conclure à une forte différenciation entre ces deux sites. Des études précédentes, réalisées dans cette même réserve, ont montré des résultats contradictoires. La première étude, réalisée en 1980 par comptage visuel, a montré que les poissons étaient plus gros et plus nombreux dans la réserve (TPA) qu'à l'extérieur (cap Béar). Cet effet serait dû principalement à une interdiction de la chasse sous-marine, mise en place dès la création de la réserve en 1974. Dix ans plus tard, une seconde étude a été réalisée sur les mêmes sites, avec des résultats parfois inverses. Deux hypothèses peuvent expliquer ces résultats contradictoires. (i) La première fait intervenir une dynamique complexe, qui serait différente de celle classiquement proposée, qui prédit que les individus âgés ou de grande taille sont plus nombreux dans la réserve. Des études comparant l'abondance et la densité de populations d'oursins à plusieurs années d'intervalle aboutissent également à ce type de résultats contradictoires. (ii) La seconde envisage un biais dans l'échantillonnage. L'estimation par comptage visuel dépend du comportement de chaque espèce, alors que la capture des poissons de nuit permet un effort d'échantillonnage indépendant du comportement. Sans étude comparative et au vu des résultats contradictoires obtenus sur les oursins, il n'est pas possible de valider l'une ou l'autre de ces hypothèses.
La présence d'individus plus grands et plus vieux dans les réserves s'explique par la réduction de l'effort de pêche. Ces observations ont été signalées chez d'autres espèces de poissons et d'invertébrés. Dans notre étude, la distribution des tailles individuelles est positivement corrélée avec le degré de protection. Le modèle classique décrit l'influence des réserves marines sur les paramètres démographiques des populations. Ces changements démographiques ont pour conséquences, d'une part, une augmentation de l'âge à la maturité sexuelle dans la réserve et, d'autre part, une augmentation de l'exportation (adultes et/ou progénitures vers l'extérieur de la réserve). Nous n'avons pas observé de modification de l'âge à la première maturité sexuelle. En revanche, l'âge d'inversion sexuelle de mâle à femelle, qui se situe aux alentour de cinq à six ans chez le sar commun, est modifié à l'extérieur de la réserve, puisque nous observons une forte proportion de femelles de quatre ans. Ces résultats complètent le modèle proposé classiquement dans la littérature. Le fait qu'un signal au niveau démographique soit détecté est en faveur d'un échange réduit d'individus entre la réserve et l'extérieur. Néanmoins, à ce stade, nous ne pouvons pas conclure à une absence complète d'échange d'œufs et de larves par les courants. Pour tester cette hypothèse, nous avons utilisé les marqueurs génétiques. La structure génétique est différente entre les individus de la réserve et ceux de l'extérieur. Cette différence n'est supportée que par un seul locus (GDA∗), ce qui limite notre confiance dans cette différenciation. Dans notre étude, nous avons volontairement pris un nombre de locus polymorphes conséquent (16 locus) pour nous rapprocher de la sensibilité des marqueurs moléculaires. Si nous considérons que les marqueurs allozymiques sont fiables, la similitude génétique que nous observons indique un flux génique (migration d'adultes et/ou exportation d'œufs et de larves par les courants) suffisant pour maintenir les mêmes fréquences alléliques entre les deux sites. Alternativement, si le flux génique est réellement réduit, la similitude génétique peut résulter d'une séparation récente des deux sites. Néanmoins, la première hypothèse semble la plus parcimonieuse, car le sar commun présente de fortes capacités de dispersion par la phase larvaire. L'effet « réserve » mis en évidence semble donc réellement un effet au niveau démographique.
La compréhension de l'effet « réserve » nécessite une bonne connaissance des communautés de poissons, mais pas uniquement en termes de densité et de biomasse obtenues par comptage visuel. Les présents résultats suggèrent qu'il faut tenir compte des particularités du cycle biologique de chaque espèce cible, à savoir l'âge, le changement de sexe, etc. D'autres facteurs pouvant influencer la dynamique des espèces cibles, comme la courantologie et la pression de pêche, sont à prendre en considération, mais ne sont pas toujours disponibles dans toutes les aires marines protégées. Ces résultats confirment le potentiel des réserves marines en tant qu'outil de gestion des espèces sensibles à la « surpêche ». En association avec les méthodes non destructrices, de nouvelles approches, telles que les marqueurs acoustiques, pourraient accroı̂tre nos connaissances sur l'effet « réserve » et les mécanismes d'exportation d'adultes.
1 Introduction
Many studies have highlighted the effects of marine reserves by comparing target species biomass and density within and outside a reserve. These studies have involved fish populations because of their commercial interest and are based on visual census methods [1–4]. With respect to invertebrate populations, studies have focused on echinoids and bivalves [5–7], and gastropods [8,9]. To date, the ‘reserve effect’ on the white sea bream, Diplodus sargus (Linnaeus, 1758), was investigated in 1980 and 1994–1995 and has become a model for studies of benthic fishes on rocky coasts [10,11].
Sea bream fishes (family Sparidae) are widespread in the northwestern Mediterranean Sea and constitute an important fishery resource along its coasts for their high value. The white sea bream, Diplodus sargus (Linnaeus, 1758), constitutes with Diplodus vulgaris (Saint-Hilaire, 1817) one of the most important fishes of infra-littoral rocky bottoms and Posidonia oceanica beds (Linnaeus) Delile. It belongs to the group of necto-benthic mesophage fishes, which are characterised by a home range with low vertical movement (from shallow water down to >50 m deep), but high-potential horizontal movement [2]. Despite their importance, no quantitative information is available on the dynamics of sparid fish populations on rocky shores, except for a few studies on the microhabitat requirements at settlement [12,13]. Adult fishes are protandrous hermaphrodites. Fishes are male at the first sexual maturity (three years old) and then become female at five years old [14]. The reproduction occurs during spring (maximum spawning in April on the French Catalan coasts) in areas that are not precisely known. The increase of capture by fishermen (trawling) seems to indicate that fishes leave the rocky coasts to migrate offshore to form several spawning groups. After one month of pelagic larval drift [15], post-larvae settle in very shallow benthic areas, where they remain for several months [13]. After reaching 4.5 to 5.5 cm in length, juveniles disperse outside the nursery areas and integrate into the adult population [16].
The aim of the present study was to describe the demographic and genetic structures of Diplodus sargus populations and evaluate the impact of protection on populations living inside and outside the marine reserve of Cerbère–Banyuls, located in French Catalonia, Mediterranean Sea.
2 Material and methods
Samples of white sea bream were collected in two sites on the French Mediterranean coasts near Spain. The first site is located in the Cerbère–Banyuls marine reserve (650 ha) created in 1974, with two zones: a Partially Protected Area (PPA) (585 ha) and a Totally Protected Area (TPA) (65 ha, created in 1979). The second site in an unprotected area (UPA). These two sites are separated by 5 km and provide two levels of protection for the fish populations. The site selected in the PPA is located near a rocky cap with the following physical characteristics: rocky bottom, 20–30 m deep. The marine reserve legislation allows amateur angling, but limits the commercial fishing activity (gill nets) (5–8 accreditations per year). Spear fishing is prohibited. In the TPA, all activities are prohibited, but scientific study. No fish was caught in this zone. The UPA site has been selected in an area (Cape Béar) with equivalent physical characteristics than PPA site and subject to fishing pressure outside the reserve. There are no fishing restrictions at the UPA site. Professional fishermen (more than 10 fishing boats) use the same gear as in the PPA (gill nets). Amateur angling and spear fishing are common activities on this rocky coast. A national championship of spear fishing is organized every year (about 100 participants in few kilometres) in the UPA site. Bell [1] ever mentioned the same level of intense recreational fishing activity when he studied the fish communities in the Cerbère–Banyuls marine reserve.
All fishes were collected by spear fishing at night between February and May 1996. Time and depth of dives were standardized in both sites. Each fish was measured to the nearest millimetre (standard length) and then dissected in order to remove otoliths (sagitta), the liver and a piece of muscle (1 or 2 g) and determine the sex. Otoliths were embedded in resin and sanded with sandpaper. The otoliths were coloured with toluidine blue to visualise annual rings. The validation of the age determination was carried out using two methods: marginal increment and tetracycline injection [17]. For genetic analysis, tissues (liver and muscle) were stored in liquid nitrogen (−196 °C) until electrophoresis. Each piece of tissue was homogenized in an equal volume of Tris/EDTA/NADP buffer (pH 6.8). The homogenate was centrifuged at 15 000 for 30 min at 4 °C. The supernatant was stored at −80 °C. The samples were then processed by routine electrophoresis on horizontal starch [17–19] and cellulose acetate plates (Titan III, Helena Laboratory). Twenty-six loci were analysed (muscle: CK∗, GAPD-2∗, PT-1∗, PT-2∗, PT-3∗, HPD-2∗, GPI-2∗, PGM∗, EST-1∗, AAT-1∗, LDH-1∗, ME∗, αGPD∗; liver: ADH∗, GAPD-1∗, LDH-2∗, SDH∗, ADA∗, MDH∗, 6PGD∗, EST-3∗, AAT-2∗, GDA∗, IDH∗, SOD∗, XO∗) with 16 polymorphic loci (99%). Allele frequencies and F-statistics (FIS and FST estimated by Weir and Cockerham, [20]) were computed with GENETIX 4.0 software. The significance of deviations from Hardy–Weinberg equilibrium and of genetic differentiation was assessed by Fisher's exact test (GENEPOP 3.1d software) [21].
3 Results
3.1 Age and size
The standard length of individuals ranged from 13.1 to 29.2 cm in the unprotected area (UPA: mean=18.4, SE=3.7, n=61) and from 15.3 to 27.0 cm in partially protected area (PPA: mean=19.6, SE=2.6, n=70). The age distribution showed that individuals were born between 1988 and 1993, corresponding to cohorts 8+ and 3+, respectively. The standard length and age frequency distributions are unimodal in both sites (Fig. 1) and individuals are significantly larger and older in the PPA (Mann–Whitney tests, standard length: Z=−3.51, p<0.001; age: Z=−4.39, p<0.001). Additionally, females are more numerous than males in both sites but the frequency distribution as a function of age is different for females within and outside the marine reserve (Pearson χ2; female: χ2=21.37−p=0.001; male: χ2=1.16−p=0.88) (Fig. 2). The mode of female distribution is the cohort 4+ (1992) and 6+ (1990) for the UPA site and the PPA site, respectively. Moreover, the cohort 4+, which represents more than 30% of the females in the UPA site, is almost non-existent in the PPA site (less than 2%). Inversely, the cohorts 6+ and 7+ are proportionally lower in the UPA site.
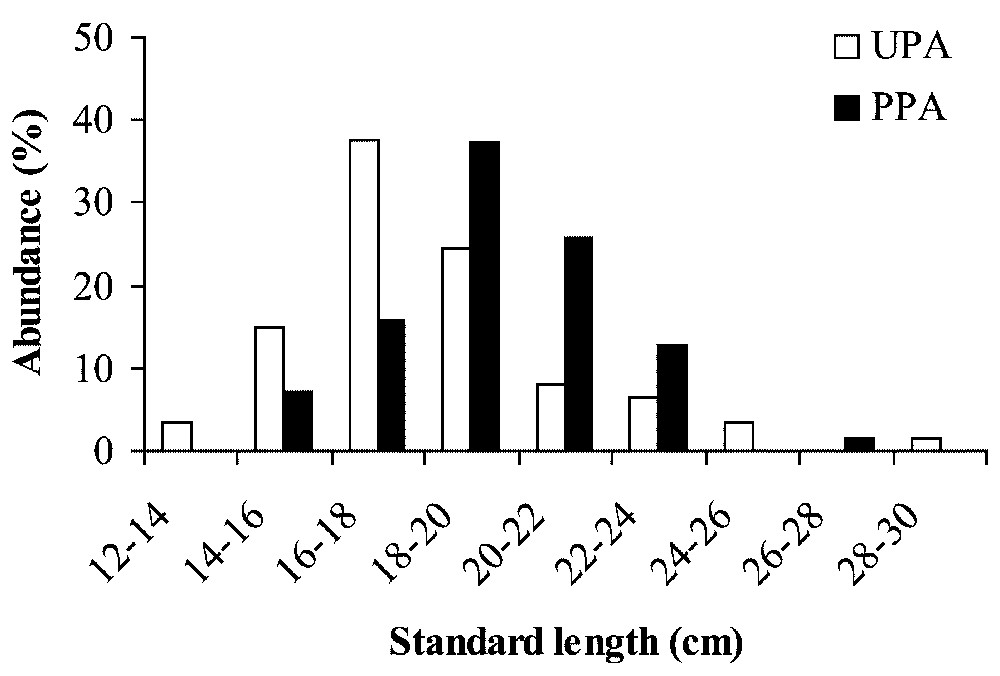
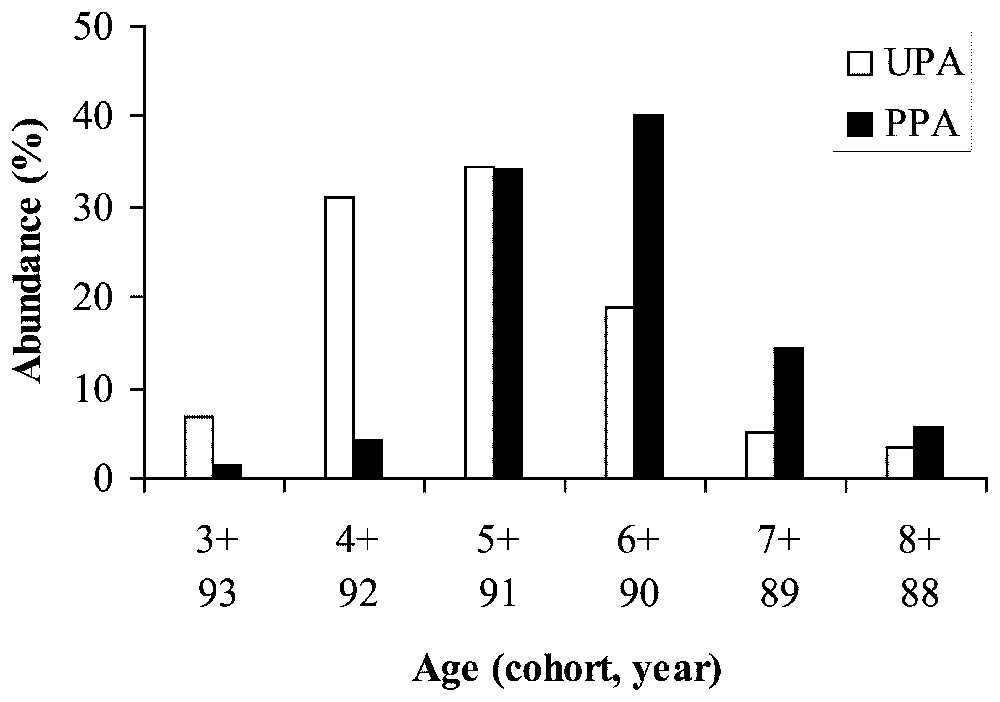
Abundance (%) as a function of size class (standard length in cm) and age of Diplodus sargus populations in an unprotected area (UPA) and a partially protected area (PPA).
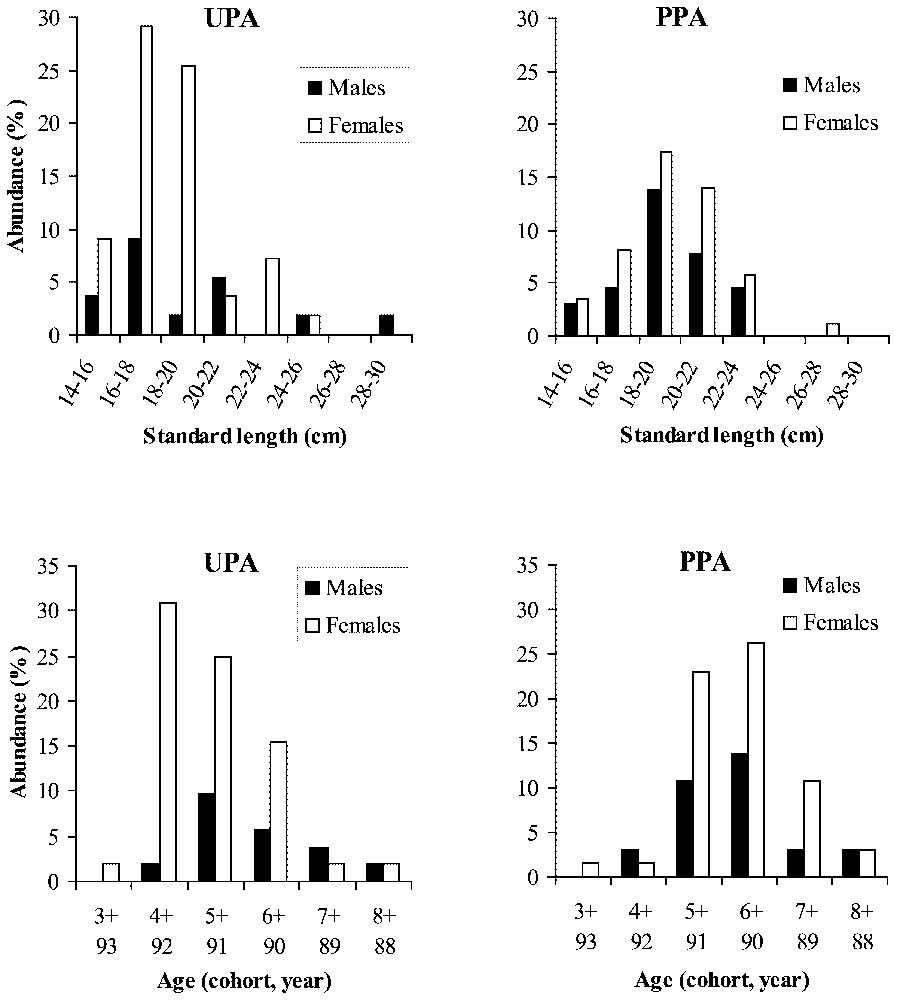
Male and female abundance (%) as a function of size class (standard length in cm) and age of Diplodus sargus populations in an unprotected area (UPA) and a partially protected area (PPA).
3.2 Genetic survey
Electrophoresis performed on 22 enzymes gave a total of 26 loci with 16 and 13 loci showing allelic variation at 99 and 95%, respectively (Table 1). Principal genetic parameters (frequency of the most common allele, observed heterozygosity per locus and multilocus, polymorphism (95%) and mean number of alleles) showed only small variation between the two sites (Table 1). No significant deviation from the Hardy–Weinberg equilibrium was observed for both sites (UPA: FIS=0.069, p=0.96; PPA: FIS=0.083, p=0.68). The FST(0.007, p=0.04) was significant essentially due to only one locus (GDA∗: FST=0.054, p=0.0003). All others loci generate an FST value not significantly different from zero.
Frequency of the most common allele frequencies (100) of all polymorphic loci found in two sites of white sea bream, including enzyme code number (EC), and buffer and tissue with best resolution. Genetic variability data includes mean number of alleles, percentage of polymorphic loci (P0.05) and observed heterozygosity (Hobs) (TC 8.0: tris-citrate, pH = 8.0; TC 6.7: tris-citrate, pH = 6.7; TBE: tris-borate–EDTA ; Pasteur et al. [18])
Polymorphic enzymes | EC No. | Locus | Buffer | Tissue | Unprotected | Partially | ||
area | protected area | |||||||
Freq. | H obs | Freq. | H obs | |||||
Adenosine desaminase | 3.5.4.4. | ADA∗ | TC 8.0 | Liver | 0.48 | 0.67 | 0.45 | 0.57 |
Glucose phosphate isomerase | 5.3.1.9. | GPI-2∗ | TBE | Muscle | 0.96 | 0.06 | 0.91 | 0.11 |
Malate dehydrogenase | 1.1.1.37. | MDH∗ | TC 8.0 | Liver | 0.65 | 0.43 | 0.73 | 0.35 |
6-Phosphogluconate dehydrogenase | 1.1.1.44. | 6PGD∗ | TC 6.7 | Liver | 0.82 | 0.26 | 0.81 | 0.34 |
Phosphoglucomutase | 5.4.2.2. | PGM∗ | TBE | Muscle | 0.75 | 0.29 | 0.81 | 0.33 |
Esterases | 3.1.1.1. | EST-1∗ | TBE | Muscle | 0.94 | 0.08 | 0.89 | 0.18 |
EST-3∗ | Liver | 0.41 | 0.62 | 0.36 | 0.57 | |||
Aspartate amino tranferase | 2.6.1.1. | AAT-1∗ | TBE | Muscle | 0.97 | 0.06 | 0.96 | 0.07 |
AAT-2∗ | TBE | Liver | 0.63 | 0.41 | 0.72 | 0.33 | ||
Guanine desaminase | 3.5.4.3. | GDA∗ | TC 6.7 | Liver | 0.43 | 0.69 | 0.25 | 0.67 |
Isocitrate dehydrogenase | 1.1.1.42. | IDH∗ | TC 6.7 | Liver | 0.79 | 0.31 | 0.83 | 0.31 |
Xanthine oxydoreductase | 1.2.1.37. | XO∗ | TBE | Liver | 0.62 | 0.49 | 0.66 | 0.44 |
Malic enzyme | 1.1.1.40. | ME∗ | TBE | Muscle | 0.72 | 0.36 | 0.71 | 0.37 |
α-Glycerophosphate dehydrogenase | 1.1.1.8. | α GPD∗ | TBE | Muscle | 0.93 | 0.13 | 0.95 | 0.10 |
Lactate dehydrogenase | 1.1.1.27. | LDH∗ | TBE | Muscle | 0.98 | 0.03 | 1.00 | 0.00 |
Superoxydase | 1.15.1.1. | SOD∗ | TC 6.7 | Liver | 0.98 | 0.03 | 0.97 | 0.04 |
Mean no. alleles | 3.06 | 2.94 | ||||||
P0.05(%) | 0.70 | 0.76 | ||||||
Hobs multilocus | 0.29 | 0.28 |
4 Discussion
The comparison of standard length frequencies, sex distribution with age and genetic structure of white sea bream populations in protected and unprotected areas shows that the demographic structure and the sex distribution of D. sargus are affected by the protection afforded by the marine reserve. The genetic structure of both sites shows a differentiation induced by only one locus (GDA∗). This is not sufficient to conclude that strong differentiation exists between these two sites.
Previous studies of fish populations in the marine reserve of Cerbère–Banyuls, including the white sea bream, show contradictory results. Bell [1] conducted the first study between the totally protected area (TPA) and unprotected area (Cape Béar) by visual census in 1980. This author showed that the density and the size of fish populations were significantly higher in the marine reserve, especially for sparid fishes (Diplodus spp.). The author considered that angling and netting prohibitions alone would not induce a significant effect within 14 months between their introduction and his study. The increased density and larger size structure of vulnerable fishes in TPA of the reserve may, therefore, be largely due to the longer term prohibition of spear fishing. Ten years later, Dufour et al. [22] did not observe significant difference in density and size between the marine reserve and the same unprotected site (Cape Béar). The authors, in fact, sometimes observed an opposite effect (medium-sized individuals more abundant outside the reserve in deep water). This absence of reserve effect may be explained by two hypotheses. (i) Complex population dynamics that are different from the process described by Sánchez Lizaso et al. [23] showing that the decrease in fishing mortality should lead to a shift towards larger and older individuals. Many studies comparing the abundance and density of sea urchin populations show variable results. Sala and Zabala [24] monitored the abundance of Paracentrotus lividus within and outside the Medes Islands marine reserve (NE Spain) for three years and reported a pattern of lower abundance in the reserve. Later, a similar survey did not found any significance difference in density and mean size [25]. (ii) A bias in the sampling methods used in these studies (visual census in Dufour et al., [22], spear fishing by divers at night in our study). Firstly, the visual census method depends on the behaviour of fishes in relation to the diver. Avoidance phenomena such as flight and hiding places can occur during the visual census and bias the biomass results [26]. In contrast, the method of spear fishing by night dives can be easily standardized in terms of duration and depth. The sampling effort is in this case independent of fish behaviour, as fishes are sleeping and the diver inspects each hole in the rocky substrate with a submarine light. Without a comparative analyse of these methods and considering contradictory results for sea urchin, we cannot evaluate these alternative hypotheses.
The presence of the larger and older individuals in marine reserves is explained by the reduction of fish mortality. A higher proportion of larger and older individuals in protected areas has already been found for various fish species in the same Mediterranean reserve [1], and in other marine reserves [3,27–32]. Similar results were found for invertebrates [5,7,8,31]. Protected areas usually lead to increases in density, biomass, diversity and/or longevity relative to populations subject to fishing pressure [33,34]. In our study, the distribution of individual size, thus the biomass and the longevity, are positively and significantly related to the protection level of D. sargus. Sánchez Lizaso et al. [23] described the possible changes in population dynamics of exploited species in marine reserves. The decrease in fishing mortality induces an increase in survival, which allows an increase in several demographic factors: mean size, age, density and biomass. These demographic changes have two potential outcomes: (i) an increase in the age at maturity for fishes living in the marine reserve and (ii) an increase in the exportation of adults and/or offspring from the marine reserve. We observed that females were smaller and younger outside the marine reserve than females caught inside the marine reserve (Fig. 2). But the white sea bream is a protandrous hermaphrodite fish, which is male at the first maturity (three years old) and becomes female at 5–6 years old. This situation is observed in the marine reserve, but outside, 4-year-old females have the highest frequency. We did not observe any increase of the age at maturity in the marine reserve. Nevertheless, we can consider that fishes become female younger outside than inside the marine reserve. It seems that the sexual inversion in females presents a plasticity allowing individuals to become female earlier outside than inside the marine reserve. The mechanisms of sexual inversion have ever been studied in hermaphrodite fishes and show a high complexity. In proterogyne species, the disappearance of dominant males induces the sexual inversion of the bigger female [35]. But this strategy is modified by the presence of other adjacent harems as in the angel fish, Centropyge ferrugatus (Randall and Burgess, 1972) [35,36]. In the case of hermaphrodite fishes, our data are the first to describe a decrease of age at sexual inversion in an exploited fish population. The sex ratio could be regulated by density dependent phenomena in relation to the proportion of each sex in the population. This mechanism complements the model proposed by Sánchez Lizaso et al. [23]. The significant signal in term of demographic structure in our study indicates that the migration of adult fishes between the marine reserve and adjacent areas is restricted. Nevertheless, at this stage, we cannot conclude that there is a complete lack of exchange by eggs and larvae using currents. To test the hypothesis of a lack of exchange, we used genetic markers (allozymes).
The genetic structure is different inside and outside the marine reserve, but this differentiation is supported by only one locus (GDA∗), which limits our confidence in the concluding genetic differentiation. In the context of the controversy of genetic method (allozyme vs DNA), several authors suggest that the polymorphism may be not as well detected using allozyme electrophoresis as using molecular markers such as RAPD or microsatellites. Allendorf and Seeb [37] have tested for differences in the patterns of divergence among different types of markers (allozymes, nuclear DNA and mitochondrial DNA) in four spawning populations of sockeye salmon (Oncorhynchus nerka). They found concordance among markers in the amount of genetic variation within and among populations. They concluded that it is important to examine many loci when estimating genetic differentiation and patterns of genetic exchange among populations. Recent work on fishes shows that allozymes may, in some cases, be more discriminating than molecular markers [38]. In this study, we have voluntarily worked with a large number of loci (16 polymorphic loci), which allows detection of polymorphism equivalent to molecular methods [39]. If we consider that allozymes are reliable, then in the absence of exchange, both populations would have to evolve differently in terms of genetic structure, essentially due to genetic drift and/or different selective pressures within and outside the marine reserve (fishing pressure, food abundance, demographic structure, etc.). In our case, the differentiation is minor, because it depends only on one locus. The genetic similarity means that gene flow (adult migration and/or exportation of eggs and larvae by currents) is sufficient to maintain the same allele frequencies between the marine reserve and the unprotected site located about 5 km away. Alternatively, if the gene flow is restricted, the genetic similarity may result from the recent separation between both sites due to the recent status of protection in the marine reserve, which was created only 18 years ago. The divergence time may have been too short to generate differences in allelic frequency. Nevertheless, the first hypothesis seems to be the most parsimonious one, because the white sea bream has great dispersal capabilities during all its life cycle (larval stage of one month, high mobility of adult). Our data on the white sea bream show that fishes inside and outside the marine reserve are very similar genetically, which means that the ‘reserve effect’ is truly a demographic one, not the result of genetic differences.
5 Conclusion
An understanding of the reserve effect requires the knowledge of fish communities not only in term of density and biomass of target species that are obtained by visual census. The present results suggest that we have to consider the life cycle particularities of target species, i.e. the age estimation, the variability of sex change or the recruitment not considered in this study. This information is not always available from visual census and requires the catch of fishes. We are faced with the problem of the catch in a marine protected area. Others factors, as currents, fishing pressures and, more generally, all pressures influencing directly or indirectly the target species are certainly important, but are not available in each marine protected areas. The results confirm the potential of marine reserves as an effective mean of managing rocky reef species, especially those vulnerable to over-fishing. In combination with non-destructive methods (visual census by scuba diving), new approaches such as acoustic tracking can increase our knowledge of reserve effects [40]. This technique could allow us to understand the mechanism of biomass export from the marine reserve with the study of adult home range.
Acknowledgements
This study was financially supported by the Regional Council of Languedoc–Roussillon (France). I thank J.-L. Binche and A. Cazeilles (marine reserve of Cerbère–Banyuls) for providing help and technical assistance. I thank Drs S. Planes and R. Galzin for their helpful comments on the first draft of the manuscript. E.B. Taylor helped in improving the English in the manuscript.