1 Introduction
Vocal sharing or convergence has been shown to reflect social affinities in several species of birds (Australian magpie [1]; indigo buntings [2]; nightingales [3]) and cetaceans (bottlenose dolphins [4,5]; killer whales [6,7]). In European starlings [8,9] and male bottlenose dolphins [10,11], vocal sharing reflects spatial associations between social partners. Both learning common structures and structural convergence are implied in all these species. Forced grouping of unfamiliar budgerigars induced convergence in contact calls [1,12].
Social influence on call usage is well known in primates [13–17]. Although flexibility of structures and production learning capacities have been described in details in many species at lower phylogenetical levels (birds: review in [18]; cetaceans: [5,11,19–21]), vocal signals are thought to be fixed at birth in primates [13,22,23]. Nevertheless, studies of chimpanzees and Japanese macaques have illustrated variability in call production related to dialects [24,25], vocal adjustment [26,27] or conditioning by caregivers [28]. More recently, trill plasticity in adult pygmy marmosets was evidenced after changes in the social environment and during the development of infant calls [29–31].
Snowdon suggested that, if flexibility is possible, (i) it is more likely to occur in affiliative vocalizations and (ii) it may be very subtle and require the use of precise measurement methods [30]. Our first step in a long-term study on vocal communication and social organization in Campbell's monkeys, was to test the hypothesis that, as found in birds and cetaceans, the vocal structures of preferential social partners would be more similar than those of non-associated group members. We focused on calls known for their implication in social interactions (cohesion–contact calls [32]). To compare frequency modulations we used an index of similarity derived from those used successfully for cetaceans and birds [6,33–35].
2 Methods
2.1 Subjects and housing conditions
The group studied included one adult male (Gavroche, 12.5 years old) and the members of two matrilines: matriline 1 (mother: Lisa 14.5; daughters: Plume 6.5, Lowina 5.5, Maricopa 4, Chilula 3) and matriline 2 (sisters: Shawnee 5.5, Tilamook 3, Bela 1.5). Only Gavroche and Lisa had been wild caught (separately) in Sierra Leone as young animals. All the others were born in captivity and were housed together since birth. Gavroche (with Lisa) had joined the group in 1998. Recordings of cohesion–contact calls could be obtained from six females (Lowina, Maricopa, Chilula, Shawnee, Tilamook, Bela) displaying ‘complete’ structures (see below). The two older females were less ‘vocal’ and produced ‘broken’ calls (see below). The monkeys were housed in an indoor (21 m2×3 m)–outdoor (21 m2×4 m) enclosure. The animals were kept in the indoor part during group observations in order to ensure more easily the identification of partners.
2.2 Data collection and analysis
2.2.1 Social interactions
Focal animal sampling was used, during direct observations using a voice recorder, to collect information on social relationships [36]: one animal was followed for 5 min and every dyadic interaction was recorded. Observations covered a period of 12 continuous days in April 1999 with three sessions a day (at feeding time the morning and the afternoon and at midday). Equal observation time was performed for each focal animal (120 min) and this yielded data on 5159 non-vocal and 249 vocal interactions.
Interactions were divided into the following six categories: pacific interactions (approach, contact, sniffing), social play, avoidance (in response to an approach, avoidance of physical contact), aggression, directed gazes, vocal exchange of cohesion–contact calls (for more details, see Lemasson et al., submitted).
Nonparametric statistics (Chi square or binomial tests – for expected frequencies <5 –, with Bonferroni correction) were used to construct the sociograms (for more details, see Fig. 4).

Sociograms and vocal sharing. Matriline 1 monkeys are on the left of each sociogram, matriline 2 are on the right. First two letters of names: abbreviations used in sociograms (vroche, sa, ume, wina, ricopa, ilula, awnee, lamook, la). The six females whose calls were recorded are in bold type. The arrows are directed from the emitter to the receiver of a behaviour; their thickness is correlated to the frequency of the interaction. Only interactions occurring more often than expected by chance are represented (chi square tests for pacific interactions, directed gazes and binomial tests for social play, vocal exchanges, aggression, avoidance with Bonferroni correction: α=0.05/8). Variants for each female are indicated as exponents.
2.2.2 Sound recordings and analysis
Calls were recorded during 17 30-min sessions in May 1999 with a unidirectional microphone (SENNHEISER MKH815) linked to a stereophonic DAT-recorder (TASCAM DA-P1). Sonograms were computed using an Amiga microcomputer program for sound analysis and synthesis [37]. The calls used for spectrographic analysis were digitised at a 24-kHz sampling rate with an 8-bit sample size. The spectrographic analysis was done using Fast-Fourier Transformations (FFT) with sizes of 256 points for each analysed time window. Resulting spectrograms had a time resolution of 2.49 ms and frequency resolution of 100 Hz.
The cohesion–contact call of Campbell's monkey is composed of first, a low-frequency part and second, a higher pitched whistle, with a large frequency modulation (Fig. 1). These two parts can be produced either separately or associated (C. pogonias [32]; Lemasson et al., in prep.). Moreover, the shapes of the frequency modulation of the second part can be either ‘complete’ or ‘broken’ (Fig. 1) and a further study indicates that the production of these two variants is context-related (Lemasson et al., in preparation). Complete calls were produced approaching a group member and, in adults, only females produced these calls. Therefore, we selected here only the complete calls, which reduced our sample size. A total of 102 calls were selected (mean=17±7 per individual). We focused on the analysis of the second part of the complete call, which was shown to support the highest level of intra- and inter-individual variability while the first part, although present in the calls of all animals, did not show such clear variation patterns (Lemasson and Hausberger, submitted). Given the duration and patterns of variation of these calls (Lemasson and Hausberger, submitted), it appeared that comparisons of sonograms required a more integrative method than simple measurements of frequency or duration parameters, classically used in primates [26,29]. A ‘similarity index’ has been used successfully to distinguish between intra- and inter-population levels in various species of birds and cetaceans [6,35,38] and in particular for European starlings' whistles [34]. Both dolphins' and starlings' whistles show the same type of modulated structure as the calls we were dealing with here. The index software used here was written by Adret-Hausberger [34], derived from Miller [33], and adapted for our species using a custom software (Richard, unpublished). The ‘similarity index’ was calculated by comparing the frequency contours of each pair of sonograms. The program automatically looked for the best superposition along the frequency and duration axis providing a ratio of ‘overlap’ between both contours. Comparisons were made both at the intra- and the inter-individual levels, every call was compared to all other calls (examples are illustrated in Fig. 2a). Other methods, like cross-correlations, were tested but revealed unable to give clear evaluations of similarities/dissimilarities between calls.

Sonograms of ‘broken’ (left) and ‘complete’ (right) cohesion–contact call.
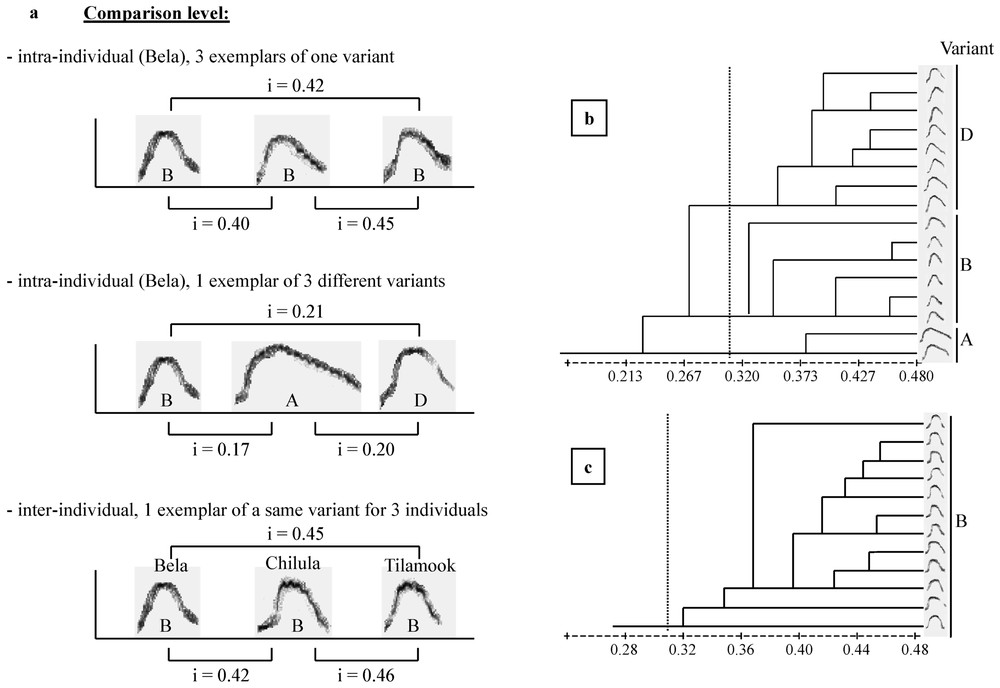
Indices and cluster analysis. (a) Examples of pairwise index values resulting from comparisons both at intra- and inter-individual and at intra- and inter-variant levels. (b, c) Cluster analysis based on dendrogram construction classifying the structures emitted by each individual. Two examples of dendrograms: (b) Bela presented three variants (A, B, D); (c) Chilula presented a single variant (B). The dotted line indicate the threshold index=0.31.
We used the UPGMA clustering algorithm to classify the structures produced by each individual (Fig. 2b and c) based on similarity indices (software NTSYS-pc, SAHN clustering program [39]). The use of this method for sound classification has increased over years given its success in songbirds [12,18,38,40,41]. On the basis of the cluster analysis, groups of calls emerged, defining variants (Fig. 2b). A threshold of i=0.31 appeared, which differentiated these variants.
Pairwise indices were then averaged to obtain a mean similarity value for intra- and inter-individual comparisons. Three levels could be discriminated:
- – intra-individual comparisons revealed that each individual had clearly separated variants (mean index within variants=0.39±0.06, mean index between variants=0.28±0.07, Fig. 3);
- – variants can be shared by more than one animal as revealed by inter-individual comparisons (mean index within variants=0.34±0.02 [vary from 0.31 to 0.38]);
- – different variants were clearly divergent (mean index between variants=0.21±0.05; vary from 0.09 to 0.30).

Distribution of variants in individuals. Horizontal lines: the six variants (A, B, C, D, E, F) presented by the females; columns: the different structures for each female. An example of a call produced by the considered female is given in the cases with the mean index and standard deviation at the intra-individual level. Empty squares show that this variant was not recorded for this animal. For example, variant A is present in the recordings of Bela and Shawnee, but was not recorded for Tilamook, Chilula, Lowina and Maricopa.
Spearman correlations were used to test correlations between intra-variant mean index values and frequencies of occurrence of some category of behaviours.
3 Results
As mentioned in the method part, we could define thresholds that corresponded to different levels of variation. One to three different variants were found per individual, some of them being present in the repertoire of different individuals (Fig. 3). Four variants were shared by at least two individuals: these were variants A (Bela+Shawnee), B (Chilula+Tilamook+Bela+Shawnee), C (Shawnee+Maricopa), D (Bela+Lowina). Only two variants were not shared and they both belonged to Lowina (E, F). All the other individuals shared at least one variant with one other or with several other group members.
Most individuals that shared vocal structures were also preferential social partners (Fig. 4). The two sisters sharing variant A often interacted vocally and pacifically, played together and observed each other frequently. The four females, belonging to two matrilines, sharing variant B, often interacted pacifically (Bela–Shawnee, Tilamook–Shawnee), vocally (Shawnee–Bela–Tilamook), observed one another (Shawnee–Bela–Tilamook) and played together (Tilamook–Chilula–Bela, Shawnee–Bela). The other two convergent pairs (Lowina–Bela, Shawnee–Maricopa) interacted neither pacifically nor agonistically. The more vocally divergent female, Lowina, was totally excluded from the rest of the group during pacific interactions, vocal exchanges and social play (Fig. 4). Furthermore, she aggressed Tilamook and was often aggressed by the adult male. Despite these observations, no significant correlation could be recorded between the index values and the frequency of occurrence of these behaviours except for avoidances. Our six females were not statistically involved in the avoidance network because of the large dominance of the adult female Plume but a significant negative correlation was nevertheless noticed between index values and frequencies of avoidance (Spearman correlation, rs=−0.579, p<0.05).
4 Discussion
The comparison of frequency modulations of cohesion-contact calls using an index of similarity revealed that each individual Campbell's monkey could present one to three different variants. These variants could be shared with one or more group members. This sharing occurred mainly within a social network of play, and included especially one matriline where social interactions were most abundant. One animal (Lowina) that tended to show few interactions and some aggressiveness displayed divergent vocal structures. Moreover, we observed that females that avoided one another less often displayed more similar structures. We were not surprised to discover a correlation with such a behavioural category given the characteristics of social life of arboreal guenons. As closely related species, Campbell's monkeys display a ‘monitor-adjust’ social system with few physical interactions, in particular rare aggressions, but a lot of survey with gaze and avoidance ([42]; Lemasson et al., submitted).
Although these data are still preliminary, given the low number of animals and limited time span of the study, one can wonder how such a sharing could be explained. Two possibilities can be proposed.
- (1) Call similarity may be due to a convergent morphology and/or way of producing sounds. Hauser thus found similarities in the coo calls of matriline members of rhesus macaques that could be explained by either by genetic transmission of morphology resulting in given ways of opening the mouth or social learning [43]. Such an influence of mouth opening on call structure has been shown in birds [44,45].
Although we cannot exclude this hypothesis with these preliminary data, especially as matriline members are particularly involved, this would not explain convergence between matrilines. Moreover, as Campbell's monkeys produce their cohesion–contact calls with a closed mouth, other aspects of call production would have to be assessed.
- (2) Variant sharing may be the result of copying and plasticity in call production. Although social influence on vocal usage has been described in a variety of contexts and species of primates [13–17,46,47], flexibility in vocal structure does not seem to be common. Most observed changes can be attributed to maturation, such as body weight changes and modification of the phonatory organ [48–50]. Recent studies however suggest that some degree of flexibility may exist in primate vocal structures. Some studies of primates present evidence that experience in specific social contexts can modify calls. Female capped gibbons, in the absence of males, produce the male as well as their own contribution to duets [51]. Contact call parameters co-vary with the nature of the contact (tactile, visual, auditory) between pairs in common marmosets [52]. Human caregivers succeeded in conditioning Japanese macaques to modify their vocalizations in a feeding context [28]. Dialects have been described in chimpanzees [25] and Japanese macaques [24]. Nevertheless, despite the fact that primates have got the cognitive capacity to use a very complex vocal communication system, as, after exceptional training, great apes have acquired artificial codes that presented some similarities to human language [53,54], very few studies illustrate vocal convergence in non-human primates. Male chimpanzees match the acoustic characteristics of each other's pant hoots when calling together [26]. Japanese macaques match the frequency parameters of their coo calls to playback signals [27]. The most detailed studies concern captive callitrichids, which change their vocal structures when a new group is introduced in their neighbourhood [29,30,55] and after pairing [31].
As mentioned by Snowdon, the methods used in order to compare calls may be determinant in finding subtle similarities or divergences [30]. In our study, the use of an index of similarity was essential in describing and classifying variants. Such a method is especially appropriate for calls that have a clear frequency modulation beyond a certain duration (e.g., whistles of bottlenose dolphins [56] or European starlings [9]).
Only a long-term study on the group dynamics, observations during ontogeny and comparisons between groups will tell us what are the determinants of these variant sharing (Lemasson et al., in preparation). If vocal copying is involved, although on subtle details of the calls, this may add to the existing evidences of a relation between social affinities and call convergence found at other phylogenetical levels: birds [1,8,12], dolphins [10,11] and humans [57] and finally break a gap in continuity of developmental processes from birds to humans [58].
Acknowledgements
We thank A. Gautier-Hion for useful discussions, J.-P. Richard for having adapted the index software and A. Cloarec for correcting English. This project received financial support from the CNRS program ‘Origine de l'homme, du langage et des langues’.