Abbreviations
DFRC, Derivatization followed by reductive cleavage; GC-MS, gas spectrometry–mass spectrometer; G, guaiacyl; S, syringyl; SIM, selected ion monitoring.
1 Introduction
Lignin is one of the major components of woody plant cell walls, and it gives rigidity to plants and allows water conduction. Lignin molecules in angiosperms are composed of guaiacyl (G) and syringyl (S) units, which are derived from coniferyl alcohol and sinapyl alcohol, respectively [6]. A difference in the ratio of G to S units in different morphological regions of some angiosperms has been shown by UV microscopy [2,3,10], bromination-TEM-EDXA [13], and the chemical characterization of various tissue fractions [1,7]. In addition, the selective radio labeling of specific units in lignin and visualization of the labeled units by microautoradiography is useful for studying the formation and structure of lignin with respect to its location in wood tissues [11,12,17]. These studies demonstrated that G lignin is deposited mainly in the early stage of xylem differentiation on vessel walls and the compound middle lamella of the fiber wall, while S lignin is deposited on fiber secondary cell walls in the later stage of differentiation.
Recent studies suggest that the precursor of syringyl lignin, sinapyl alcohol, is biosynthesized via feruloyl–CoA thioester, coniferaldehyde, and sinapaldehyde without forming sinapic acid [4,5,8,14]. However, we previously demonstrated that exogenous ferulic acid was incorporated into G and S lignin, and exogenous sinapic acid was also incorporated into S lignin in robinia [9,15]. This suggests that sinapic acid is an intermediate of syringyl lignin. Sinapyl alcohol is formed from ferulic acid via the aromatic ring modifying reaction (the hydroxylation and the methylation) and the reducing reactions (the reduction of acids to alcohols), whereas it formed from sinapic acid only via the reducing reductions. It is important to clarify the differences in the regions where sinapic and ferulic acid are incorporated in differentiating xylem in order to elucidate the enzymatic systems responsible for modifying the aromatic ring and reducing the side chain.
In this study, we prepared different stage of continuous sections of newly formed xylem from robinia fed labeled ferulic and sinapic acids, to reveal where these compounds are incorporated into S lignin in the differentiating xylem. We estimated the distribution of the labeled lignin by using the derivatization followed by reductive cleavage method (DFRC), followed by gas chromatography–mass spectrometry (GC-MS). The difference in the in situ incorporation of the labeled precursors is discussed in the regulation of sinapyl alcohol biosynthesis in the differentiating xylem.
2 Material and methods
2.1 Synthesis of labeled precursors
[8-13C, 3-OCD3]-ferulic acid and [8-13C, 3,5-OCD3]-sinapic acid (Fig. 1) were synthesized as described previously [9,15].
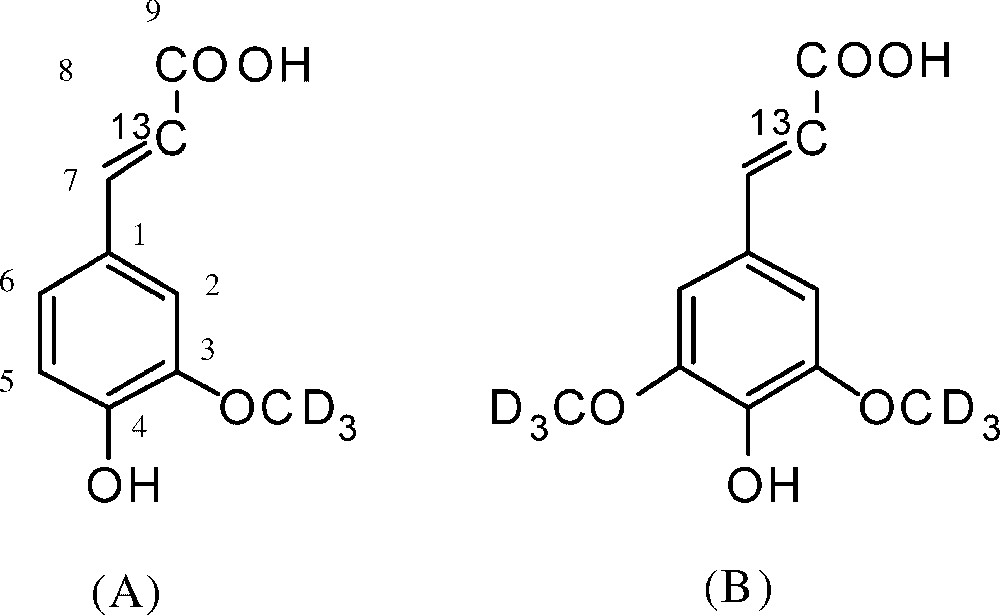
Precursors labeled with stable isotopes. (A) Ferulic acid-[8-13C, 3-OCD3]. (B) Sinapic acid-[8-13C, 3, 5-OCD3].
2.2 Plant material and administration of precursors
The upper parts of 2-year-old shoots of robinia (Robinia pseudoacacia L.) trees that were growing on the campus of the Nagoya University were cut off in July 2000. A small depression was made at the top of the remaining stem and was filled with 30 ml of a 2-mM aqueous solution of each precursor dissolved in a phosphate buffer (pH. 7.01, 25 °C). After 9 days, each shoot was harvested and was soaked in 80% ethanol. And then 50-μm-thick tangential sections were cut from the surface of the bark-free xylem with a sliding microtome. All section was treated by ethanol-benzene (1:2) for 8 h to remove the labeled precursor and low molecular phenolic compounds. The analyses of lignin by the DFRC method were performed using these sections, as shown in Fig. 2 and described previously [15].
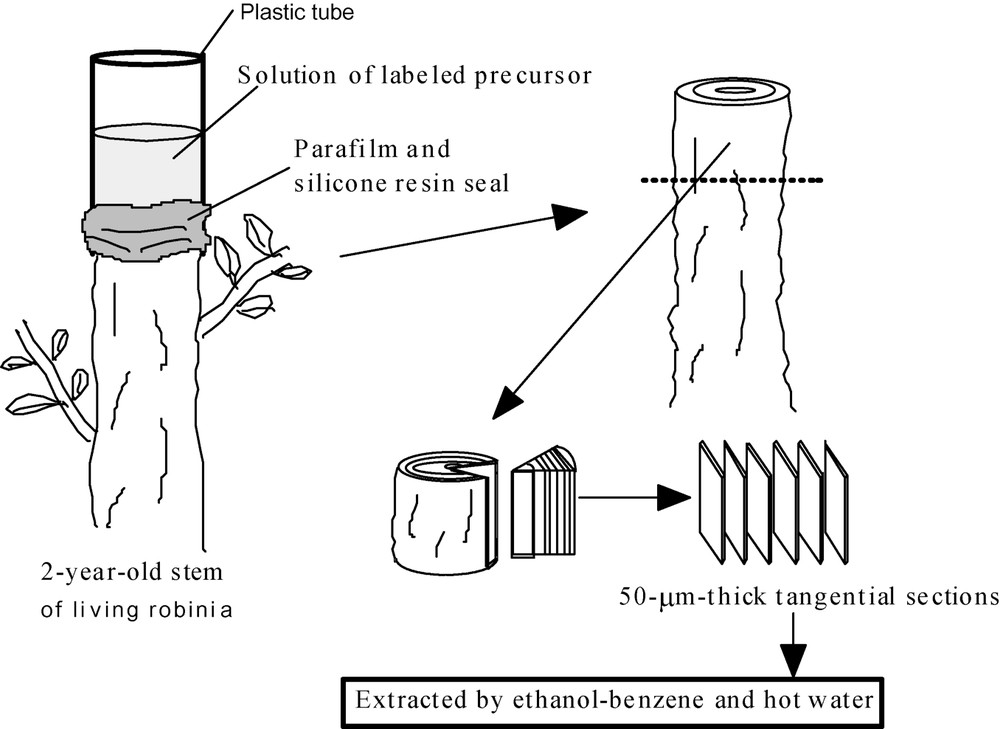
Administration of labeled precursors and sample preparation.
2.3 Analysis of lignin
To degrade and detect the labeled lignin, each section (approximately 1 mg) was applied to the DFRC method [16] followed by GC-MS, with some modification as followed; AcBr stock solution: acetyl bromide/acetic acid (1:4, v/v); Acidic reduction solvent: dioxane/acetic acid/water (5:4:1, v/v/v). To wood section was added the AcBr stock solution (0.5 ml). The mixture was gently stirred at 50 °C for 3 h. The solvent was completely removed by blowing down of N2 gas. The residue was dissolved in the acidic reduction solvent (0.5 ml). Zinc powder (10 mg) was added to a well-stirred solution. Stirring was continued for 30 min. To the mixture were added dichloromethane (1 ml), a saturated ammonium chloride solution (1 ml), and an internal standard (docosane in dichloromethane). The pH of the aqueous phase was adjusted to less than 3 by adding diluted HCl. The aqueous phase was extracted twice more with dichloromethane. The combined dichloromethane fractions were dried over anhydrous Na2SO4, and evaporated in vacuo. The residue was acetylated for 40 min in 1.5 ml of dichloromethane containing 0.2 ml of acetic anhydride and 0.2 ml of pyridine. All volatile components were removed completely by co-evaporation with ethanol in vacuo.
Acetylated DFRC products were analyzed by GC-MS. Mass spectra were recorded at 70 eV with a GCMS-OP2010 (Shimadzu, Kyoto, Japan), with a fused silica capillary column (DB-1,
3 Results and discussion
Fig. 3 shows the distribution of the DFRC degradation products (GDFRC, SDFRC) in the robinia given labeled ferulic acid. DFRC method only produces these products from 8-O-
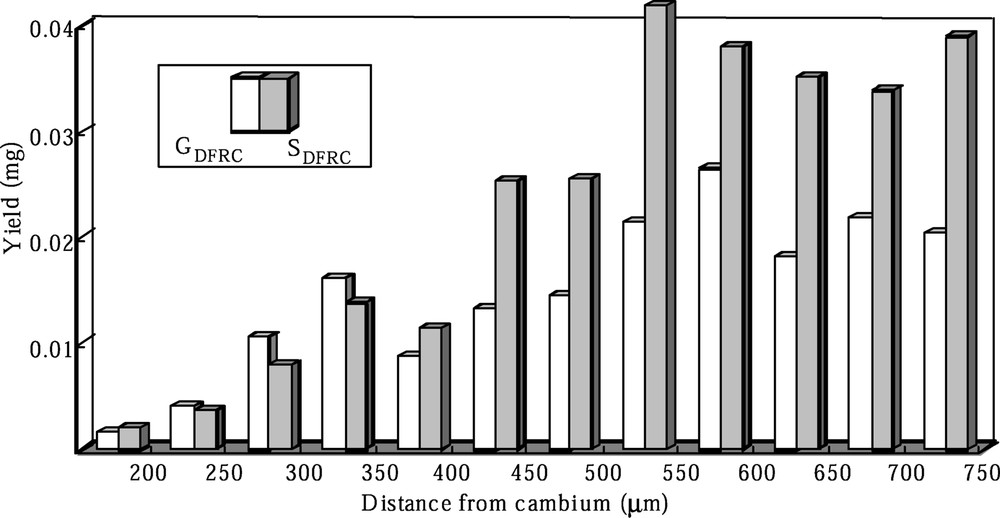
Distribution of the DFRC products (GDFRC, SDFRC) in the robinia given labeled ferulic acid.
Fig. 4 shows the partial mass spectra of each DFRC product (GDFRC and SDFRC) in controls (A) and robinia fed with labeled ferulic (B) or sinapic (C) acid. Each figure shows where the labeled precursor was incorporated into lignin. The molecular ion peaks for GDFRC and SDFRC monomers are at
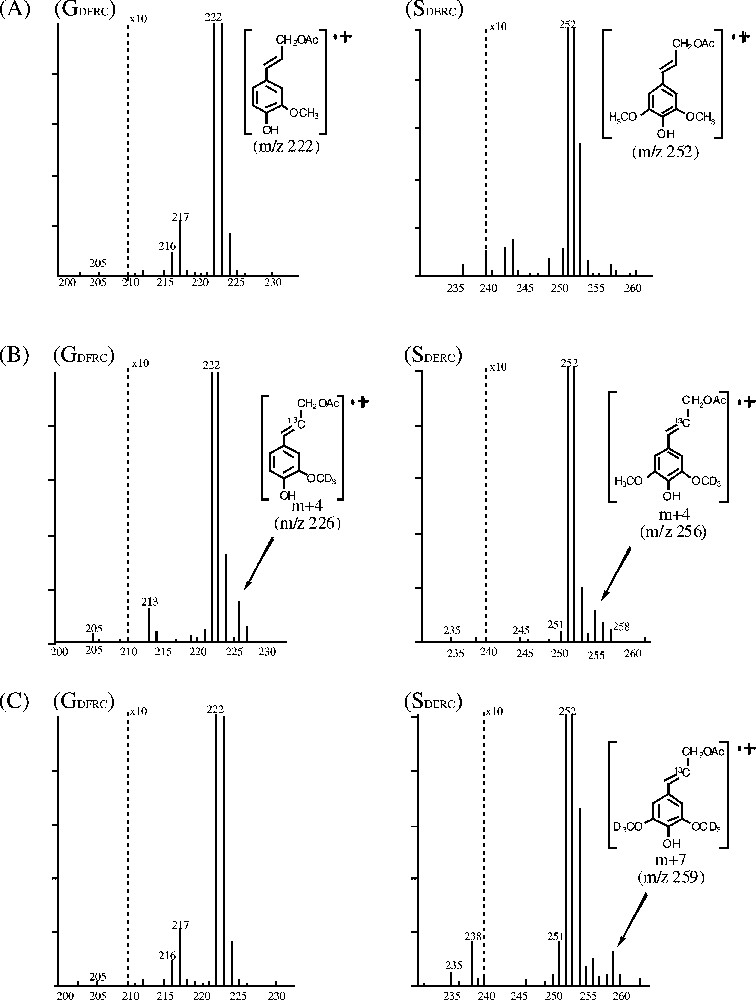
Partial mass spectra of each DFRC product in robinia. (A) Control, no feeding; (B) fed with labeled ferulic acid; (C) fed with labeled sinapic acid.
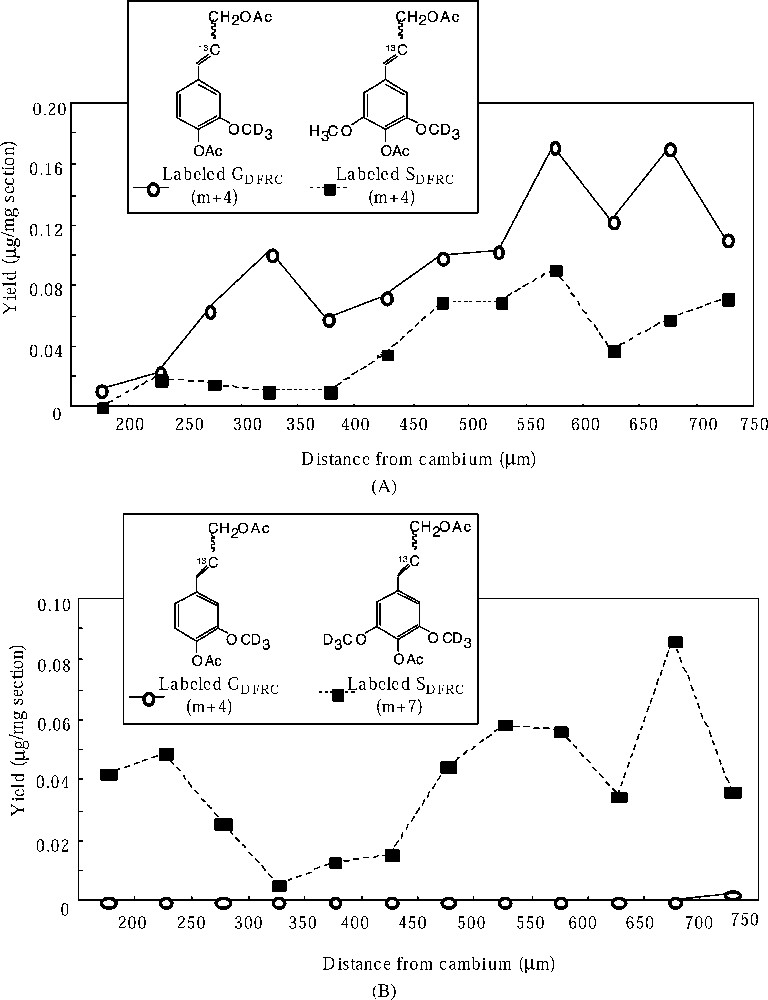
Distribution of the yield of labeled DFRC products. (A) Labeled GDFRC (m+4) and SDFRC (m+4) in robinia fed with labeled ferulic acid. (B) Labeled GDFRC (m+4) and SDFRC (m+7) in robinia fed with labeled sinapic acid.
Labeled ferulic acid has begun to incorporate into G lignin in the early stages of cell-wall lignification and the incorporations increased as cell-wall lignification proceeded. And the labeled ferulic acid is also incorporated into S lignin during the late stage of lignification. These localizations corresponded with the heterogeneous deposition of lignin moieties. Labeled sinapic acid was incorporated into S lignin in the early and late stages of lignification. However, the deposition of S lignin occurred mainly in the later stage of lignification. Thus, the incorporation of labeled sinapic acid into syringyl lignin in the early stage would be abnormal conversion.
These results indicated that the exogenous ferulic acid was incorporated in the normal lignin biosynthetic process, including the regulation of coniferyl and sinapyl alcohol biosynthesis, whereas the exogenous sinapic acid was not. The conversion of ferulic acid to sinapyl alcohol goes via the aromatic ring modifying reaction (the hydroxylation and the methylation) and the reducing reactions (the reduction of acids to alcohols). The conversion of sinapic acid to sinapyl alcohol goes only via the reducing reactions (the reduction of acids to alcohols). Therefore, the regulation of the syringyl lignin biosynthetic process would occur in the aromatic ring modifying reaction (the conversion from guaiacyl to syringyl moiety) in the differentiating xylem.
4 Conclusions
In robinia, sinapyl alcohols are generated mainly in the later stage in the differentiating xylem. For the regulation of the sinapyl alcohol biosynthesis, the aromatic ring-modifying reactions including the hydroxylation and methylation (the conversion from guaiacyl to syringyl moiety) are more important than the reducing reactions (the reduction from sinapic acid to sinapyl alcohol).
Acknowledgment
This study was partly supported by Grant-in-Aids for Scientific Research (Nos. 14360097 and 15255016) from JSPS and for Bio Design Program from the Ministry of Agriculture, Forestry, and Fisheries of Japan.
Vous devez vous connecter pour continuer.
S'authentifier