1 Introduction
Secondary walls of dicotyledons are composites made of cellulose and glucuronoxylans (GX) often associated with a reinforcement of lignins [1]. When consolidated, they represent permanent constructions that are hardly destructible. They are found in stone cells, sclereids and wood components in which they ensure a role of support, protection and conduction, all properties characteristic of land plants. The actual wall texture is impossible to visualize by a direct observation with the transmission electron microscope (TEM). Since the pioneer studies of N. Parameswaran and his group [2,3], the development of techniques of wall decrustation [4–9] has allowed us to expose the actual cellulose framework of the secondary walls and at the same time to decipher some modalities of their construction.
The present paper is devoted to the helicoidal organization of secondary walls. We show that the construction of consolidated cell walls implies a phase, if a brief one, of cholesteric liquid-crystal-like ordering. A simple hypothesis proposes that GX could be implied both in the assembly of the cholesteric composite and in its hardening by lignin incrustation.
2 The basic helicoidal pattern of consolidated walls is liquid-crystal-like
It is known that numerous cell walls are constructed according to a helicoidal pattern, the so-called helicoidal plywood [4]. Basically, it is made of regular series of planes in which the direction of the cellulose microfibrils is changed by a regular angle, as stairs of a spiral staircase, with an axis perpendicular to the cell surface (Fig. 1A). In fact, a great flexibility exists and alterations of the basic model are often relevant to differentiation [4,8,9] (Fig. 1B).
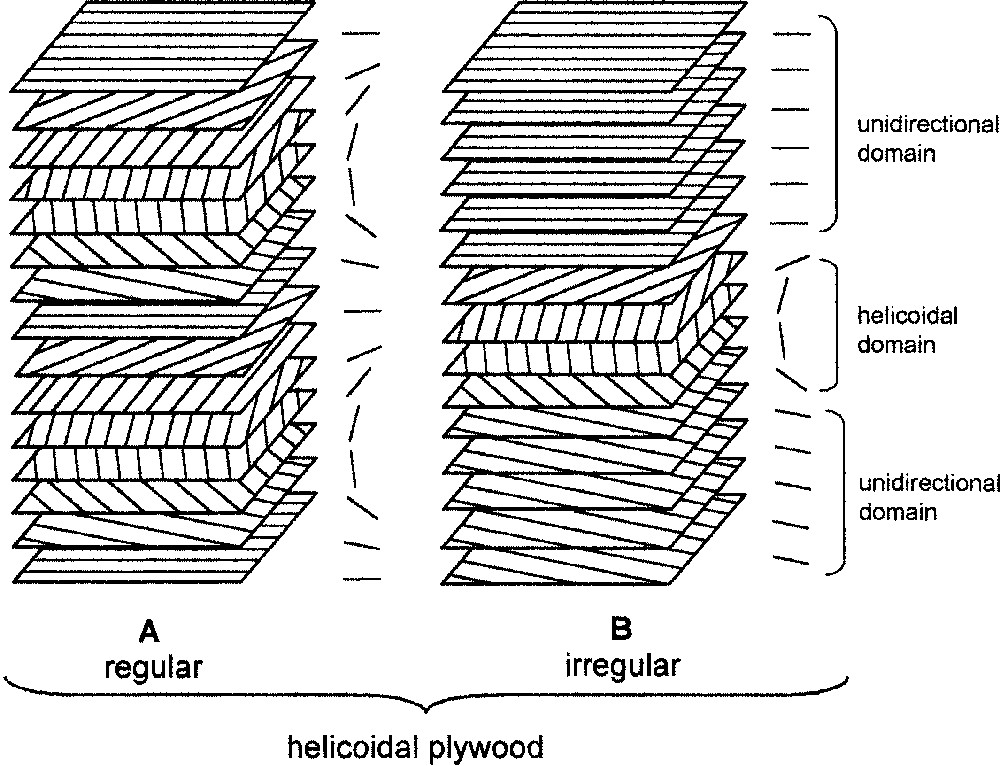
Schematic organization of helicoidal structures. A: Regular plywood; B: irregular plywood with transient helicoidal domain.
We illustrate several examples of stony cells: the endocarp of cherry and walnut forming a coherent tissue protecting the seed (Figs. 2–5) and stone cells of pear that constitute groups of cells within the fruit pulp (Fig. 4). Whatever the example, the thickness of the wall is very important, reaching 10 μm, and the cell lumen is dramatically reduced at maturity. The cells are spherical close structures, being either regularly polyhedral (Fig. 2) or polylobate resembling a jigsaw puzzle (Fig. 3). Moreover, numerous pit canals, the role of which is to maintain the symplastic transport, open gaps and produce a recess during wall construction, all factors that are evidently the origin of constraints during wall assembly.
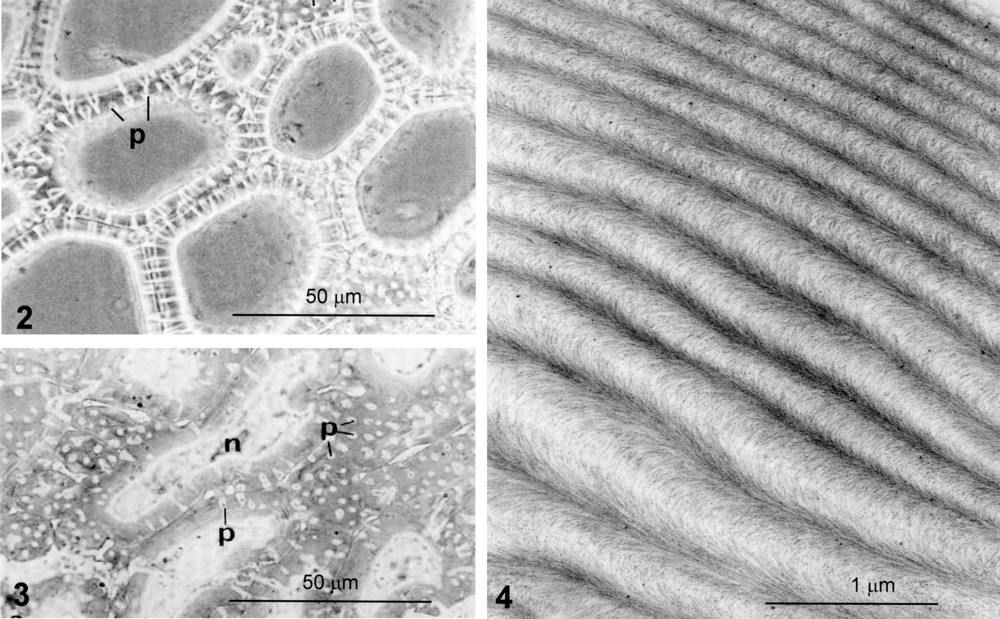
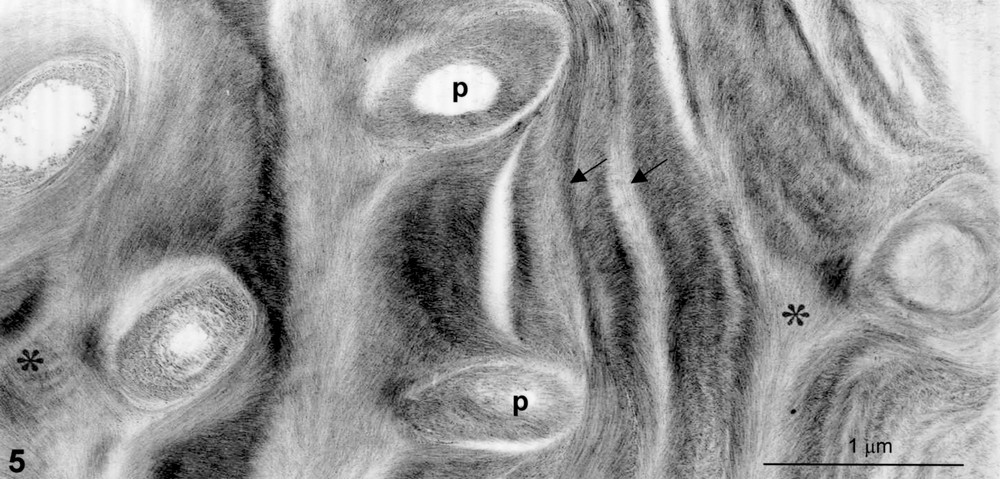
Cherry endocarp in the course of secondary-wall thickening and lignification. Freehand section. Phase contrast. Polygonal cells forming a coherent tissue. The walls are thick with numerous pit canals, p, front or profile view. From [6].
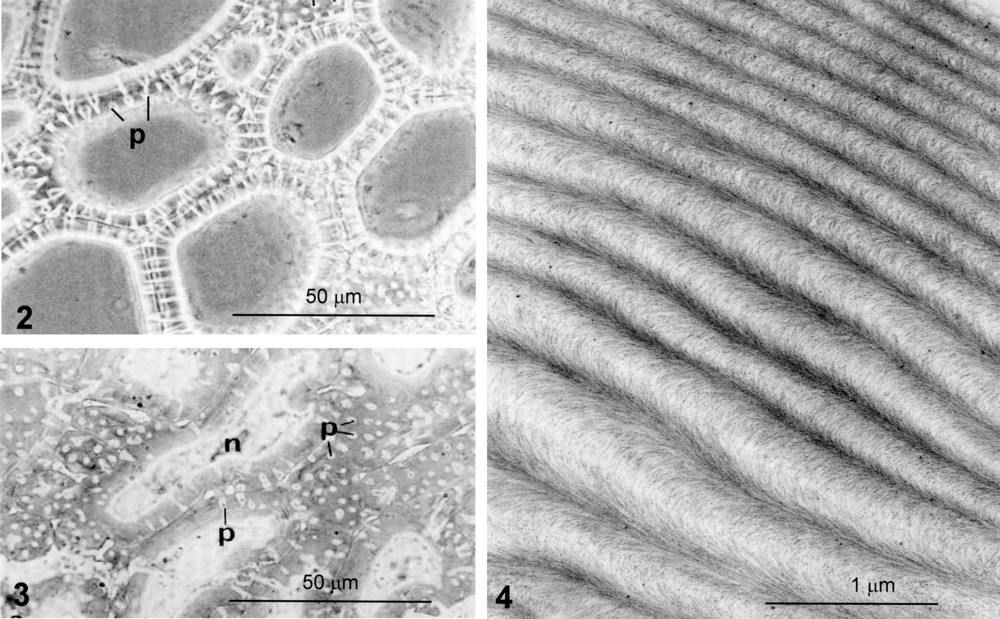
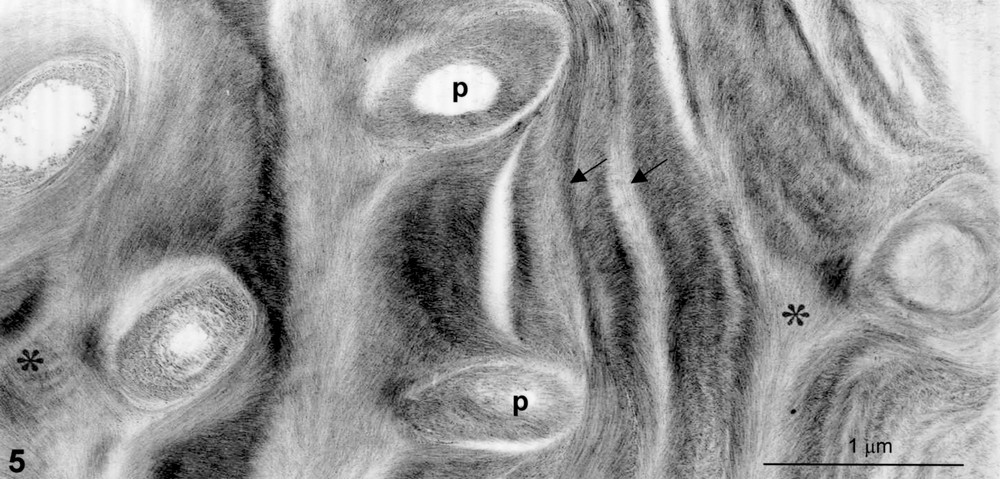
Walnut endocarp in the course of secondary wall thickening and lignification. Polylobed shaped cells, bulged and associated like the pieces of a puzzle; p, pit canal; n, nucleus. From [7].
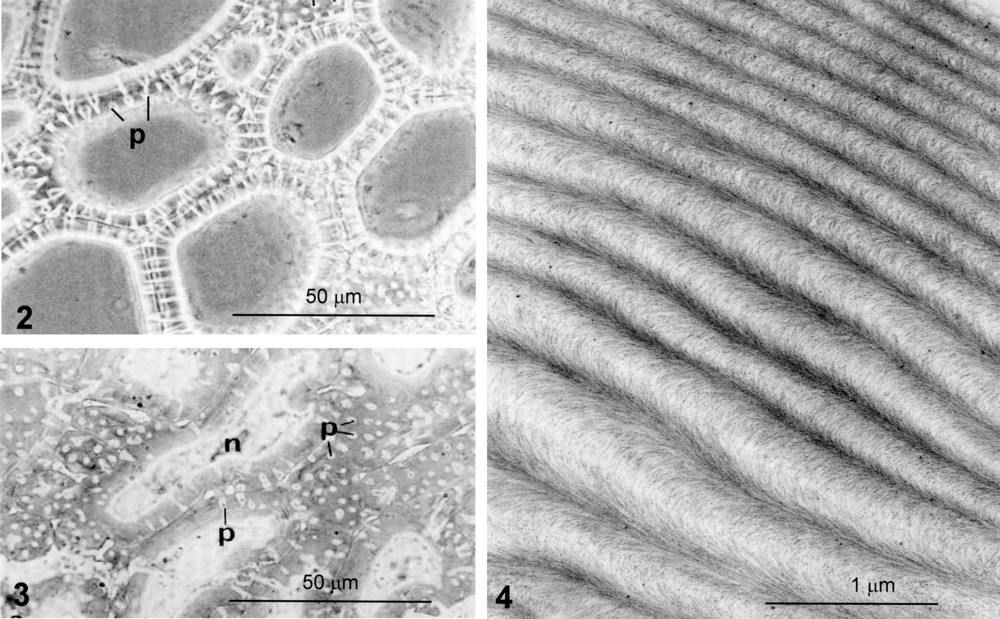
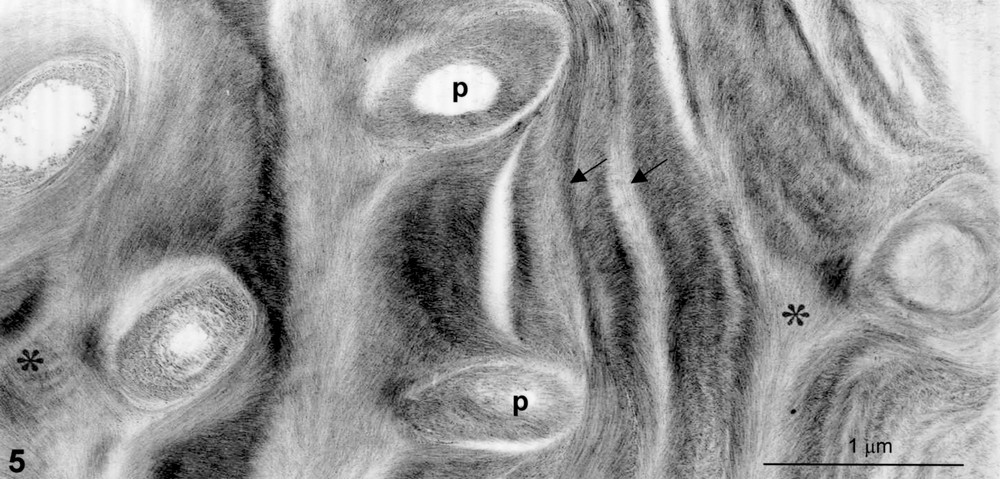
Helicoidal pattern in stone cells of pear. PATAg test of polysaccharides following extraction of lignin and hemicellulose matrix. Bow-shaped arcs that are regular and monotonous.
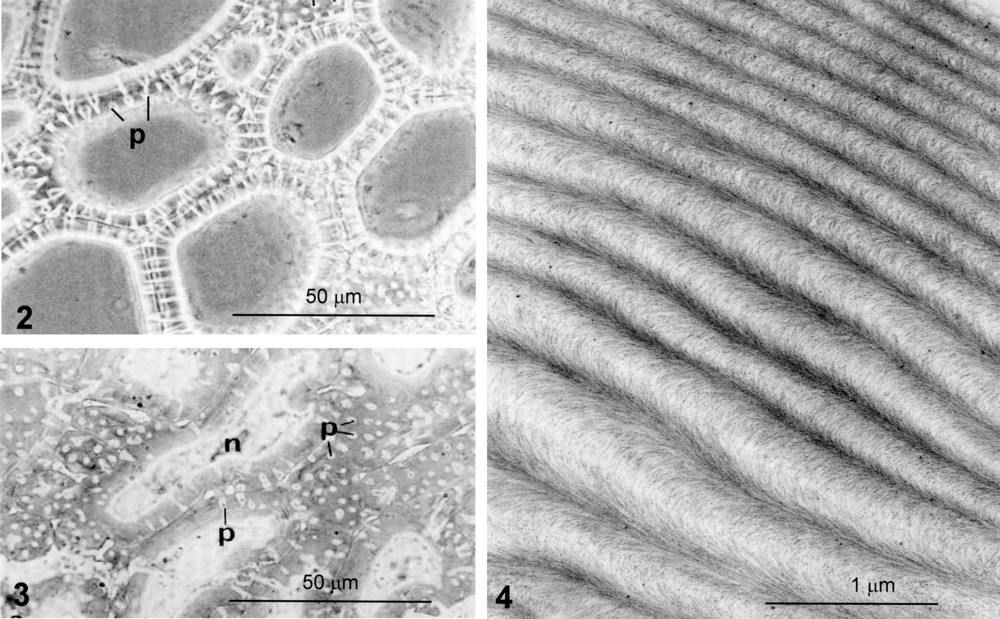
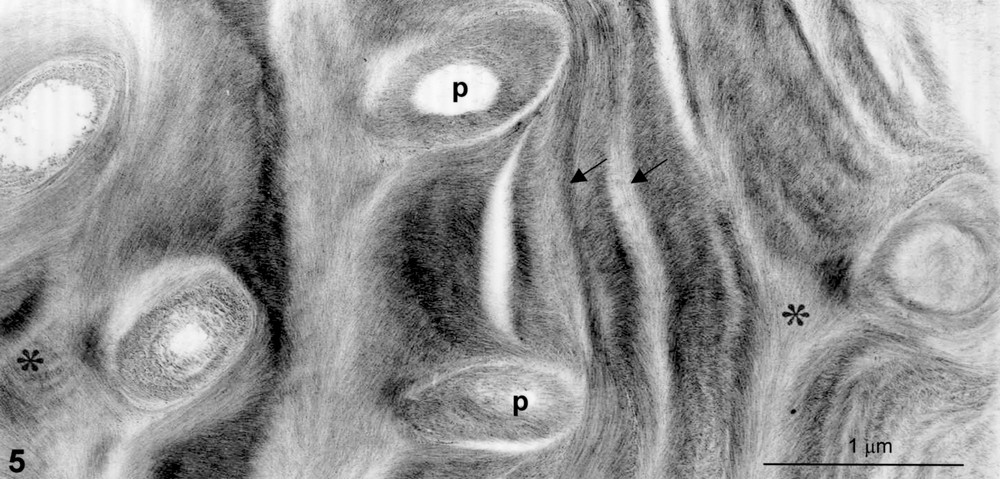
Helicoidal pattern in cherry endocarp. Zone rich in pit canals, p. Defects are visible: distortion (arrows), vortices in pits, saddle-like disclination (stars).
Fig. 4 provides the aspect of a typical helicoidal pattern where regular and monotonous nested arcs are seen, the thickness of the arc depending on the sectioning plane. The final number of arcs is variable, the maximum being up to 80–100 in stone cells. Such construction is relevant to a regular helicoidal structure (Fig. 1A) [4,9,10]. Besides these regular plywoods, apparent imperfect helicoidal arrays are also often encountered, especially in zones where pit canals are abundant. In these areas, one can recognize characteristic figures of distortions, vortices and disclinations such as saddle-like figures (Fig. 5). All these defects are diagnostic of a liquid-crystal behaviour submitted to strains. As it has been discussed from other biological models, the defects that develop here provide information on the liquid-crystal phase that could operate during wall construction [11,12].
On the whole, both the helicoidal texture and the occurrence of characteristic defects strongly suggest that the wall ordering is relevant to a cholesteric liquid-crystal assembly, if only briefly, that is rapidly and strongly consolidated by lignification [6,7]. It is clear that the constraints imposed by the spherical shape and the occurrence of pits are not an obstacle to the construction of an actual cellulosic helicoidal system.
3 Localization of glucuronoxylans reveals their possible role in helicoidal construction
At maturity, the cell wall of stony cells contains about 30% of cellulose, 30% of GX and 40% of lignins. An immunodetection of GX was performed in the early stages, before lignification. An overview at the light microscope of cherry endocarp clearly shows an intense labelling in endocarp cells, whereas the neighbouring mesocarp cells, the cell walls of which are thin and not helicoidal in organization, are unlabelled (Fig. 6). Examination at higher magnification with the TEM of a contact endocarp/mesocarp confirms that the GX are present only in the endocarp (Fig. 7). They are regularly distributed within the whole thickness of the secondary helicoidal wall and are absent in the compound ‘middle lamella–primary wall’ and in pit canals (Fig. 8).
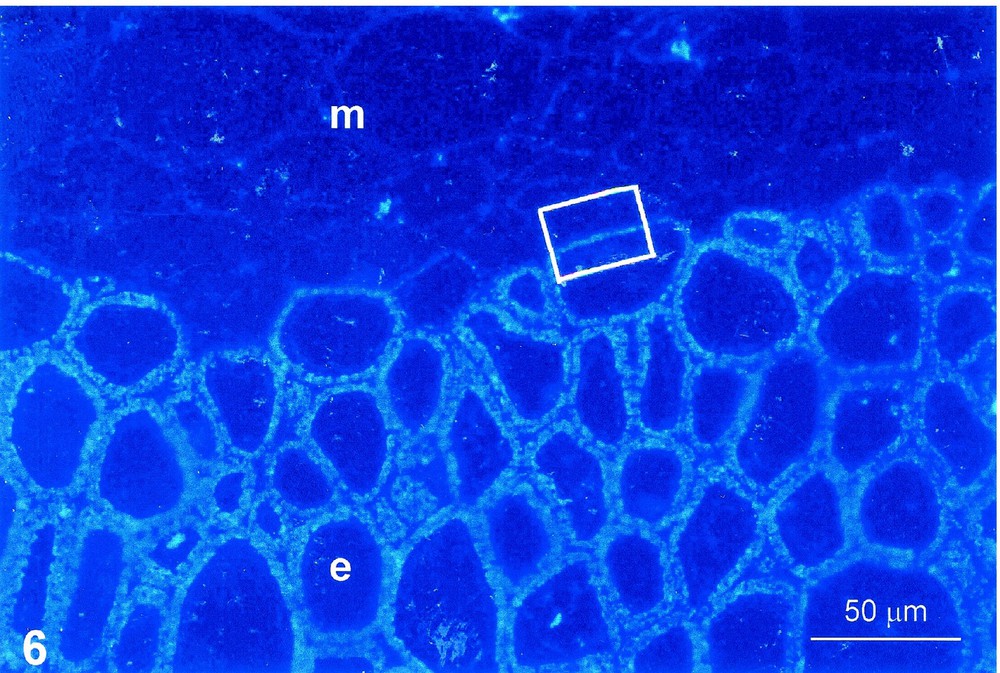
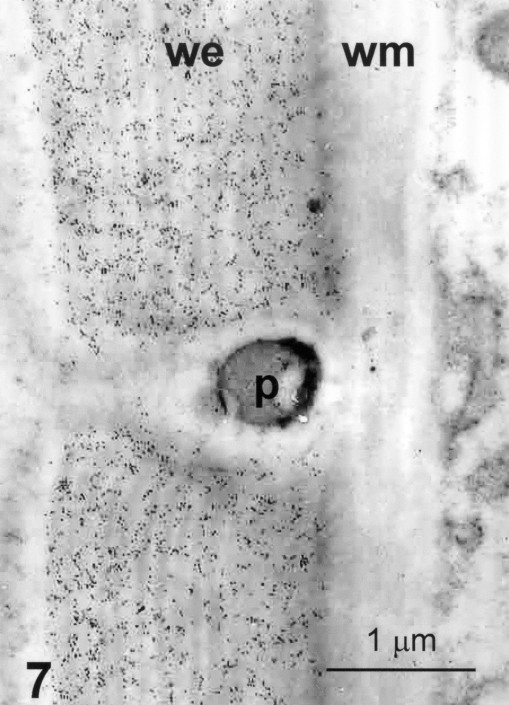
Immunodetection of GX. Cherry endocarp, 30 days post anthesis. Semithin section, anti-GX antibody, colloidal gold–silver intensification, UV epipolarisation. Cell walls in the course of secondary thickening, not yet lignified. Intense labelling of GX in secondary walls of endocarp, e. No labelling in mesocarp, m.
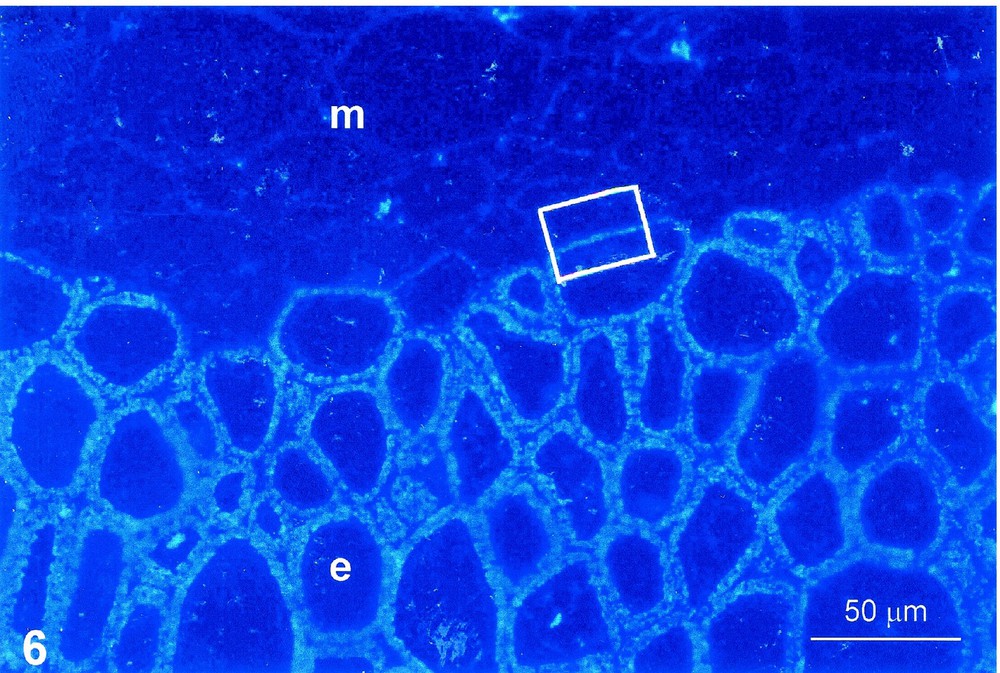
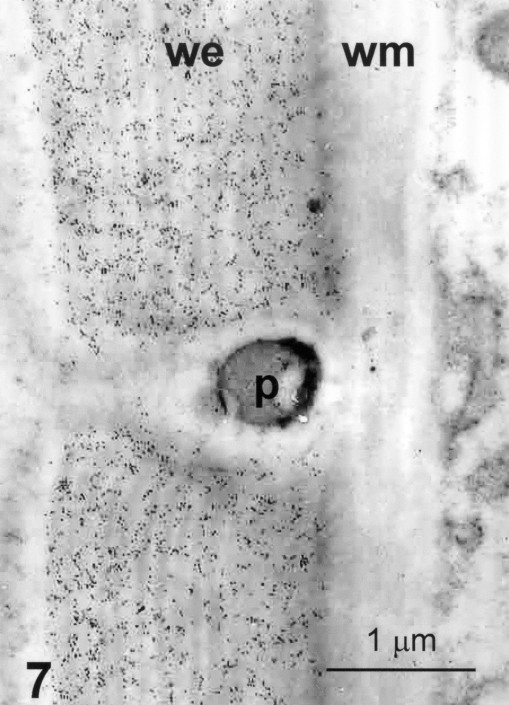
Immunodetection of GX. Same material. Ultrathin section. Anti-GX antibody, colloidal gold. Detail of the square area of Fig. 6. Homogenous labelling within the whole thickness of the endocarp secondary wall, we. The wall of adjacent mesocarp cell, wm, and the middle lamella are not labelled; p, pit canal.
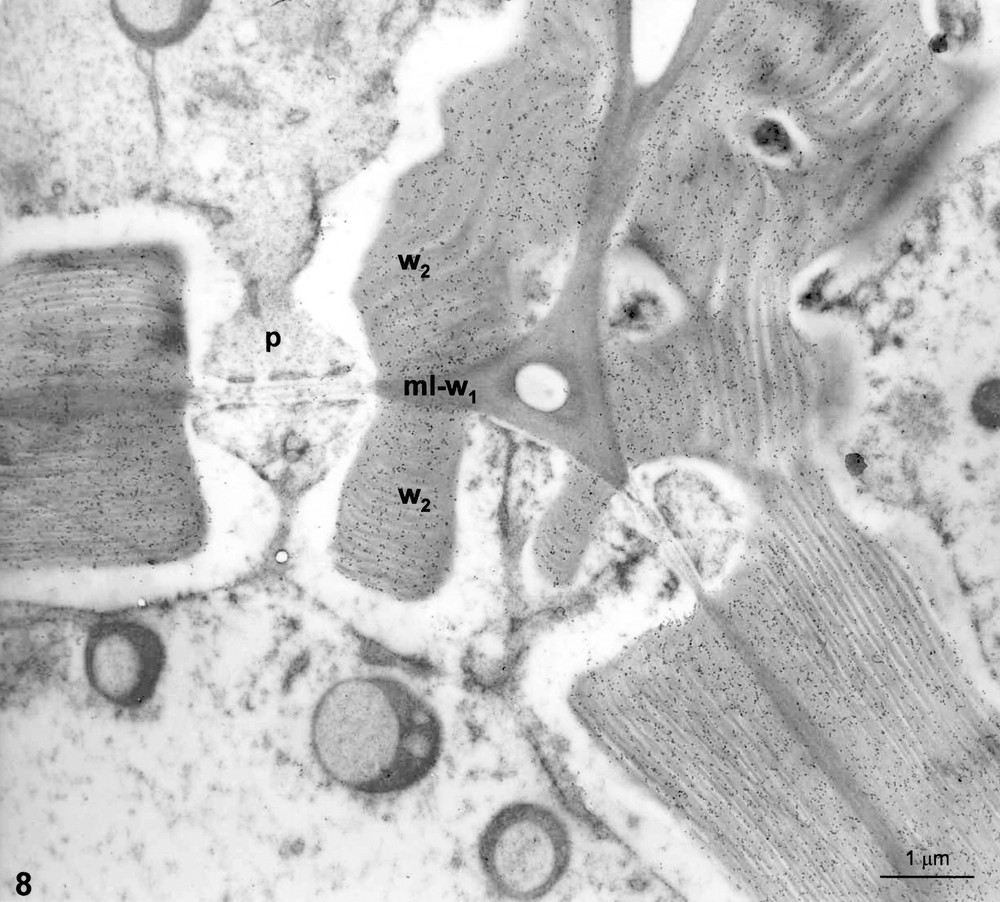
Immunodetection of GX. Same technique as for Fig. 7. Walls in the course of thickening and lignification. Intense and regular labelling on secondary walls, w2, on helicoidal structures, both in regular and distorted regions. Note that no labelling occurs on middle lamella–primary walls, ml–w1, and pit canals, p.
Therefore, in secondary cell walls, a narrow correlation exists between the occurrence of GX and the occurrence of helicoidal organization. Cellulose and GX are clearly synchronically deposited when the helicoid is built, before lignification.
The case of cell walls of wood fibres provides another interesting example of secondary wall organization. Classically, it is known that the wall structure of fibres is typically three-layered (S1, S2 and S3 layers). Fine observations revealed the occurrence of helicoidal transition domains giving rise to a V-shape (Fig. 9). Such constructions correspond to an irregular plywood (Fig. 1B) [5,6,13]. An affinity labelling of GX by means of xylanase–gold complexes [5] revealed a preferential localization of GX in the transition zone between S1 et S2 layers, precisely in the zone where the transient helicoid occurs (Fig. 10). Moreover, a surface labelling of extracted cellulose microfibrils revealed the existence of an acid coat bonded onto the microfibrils, thus indicating a tight coating of the cellulose microfibrils by the GX [7,13] (Fig. 11). The acidic surfactant is likely responsible for the spacing pattern of the carbohydrate components by preventing the aggregation of the parallel-aligned microfibrils (Fig. 12A).
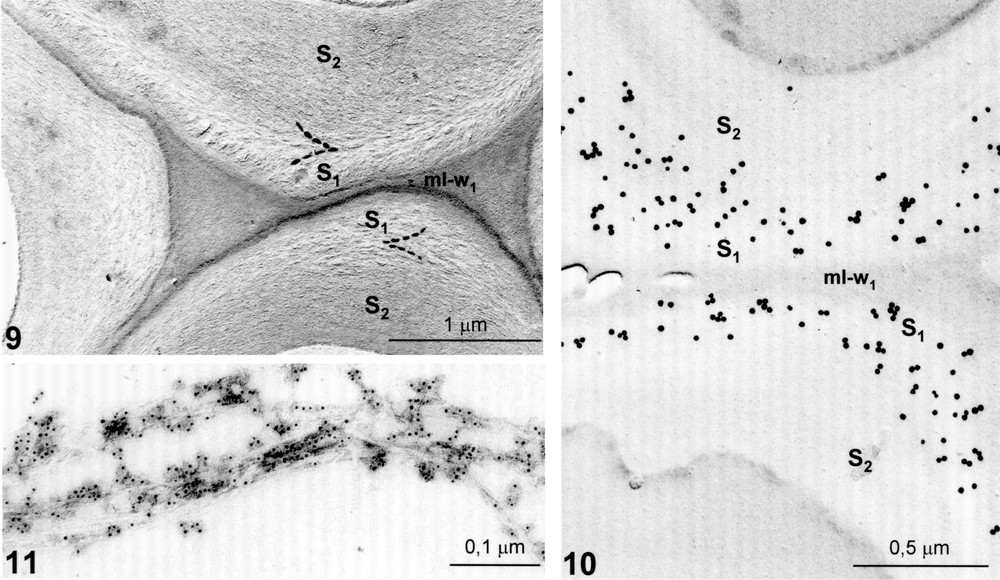
Cell wall of linden tree fibre. Chlorite delignification. DMSO extraction. Shadowing on thin section. Transitory helicoid between two blocked zones S1 and S2 of the secondary wall with a V-shaped appearance (dotted lines); ml–w1, middle lamella–primary wall. From [5].
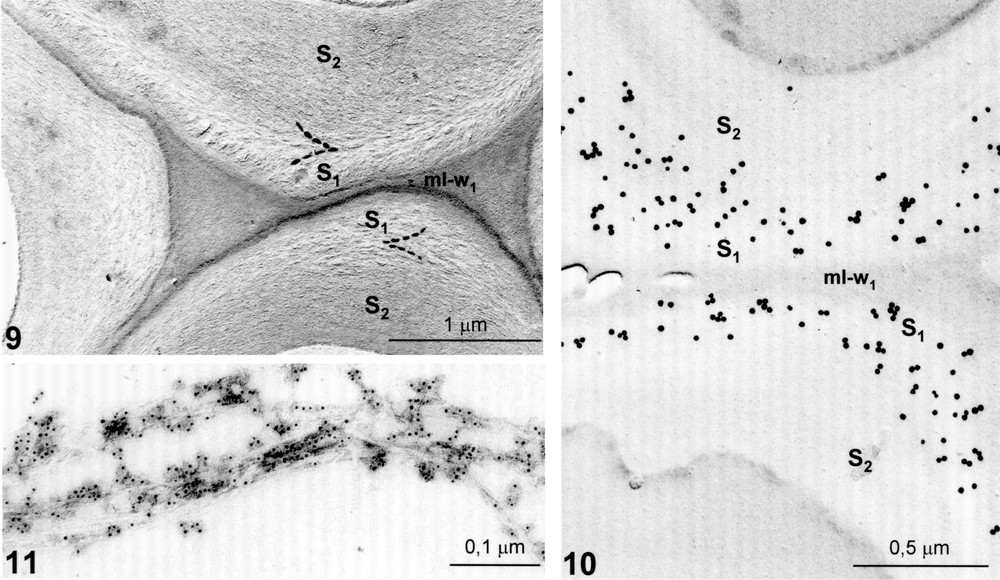
Localization of GX by means of xylanase–gold labelling. Platinum shadowing. Preferential localization of GX at the boundary between S1 and S2; ml–w1, middle lamella–primary wall. From [5].
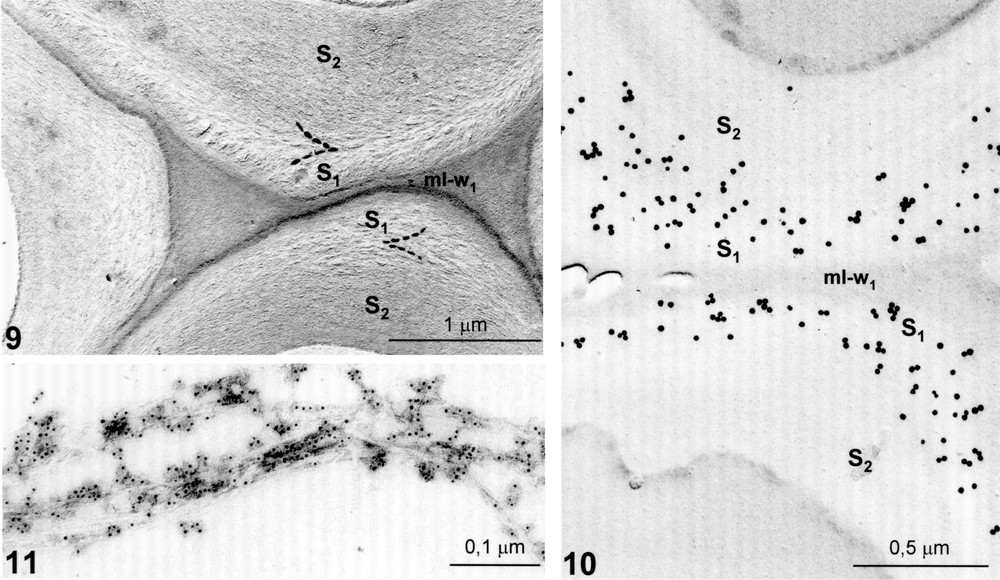
Decoration of cellulose microfibrils with cationic gold particles revealing GX surfactant. Stripped microfibrils dissociated by ultrasonic treatment.
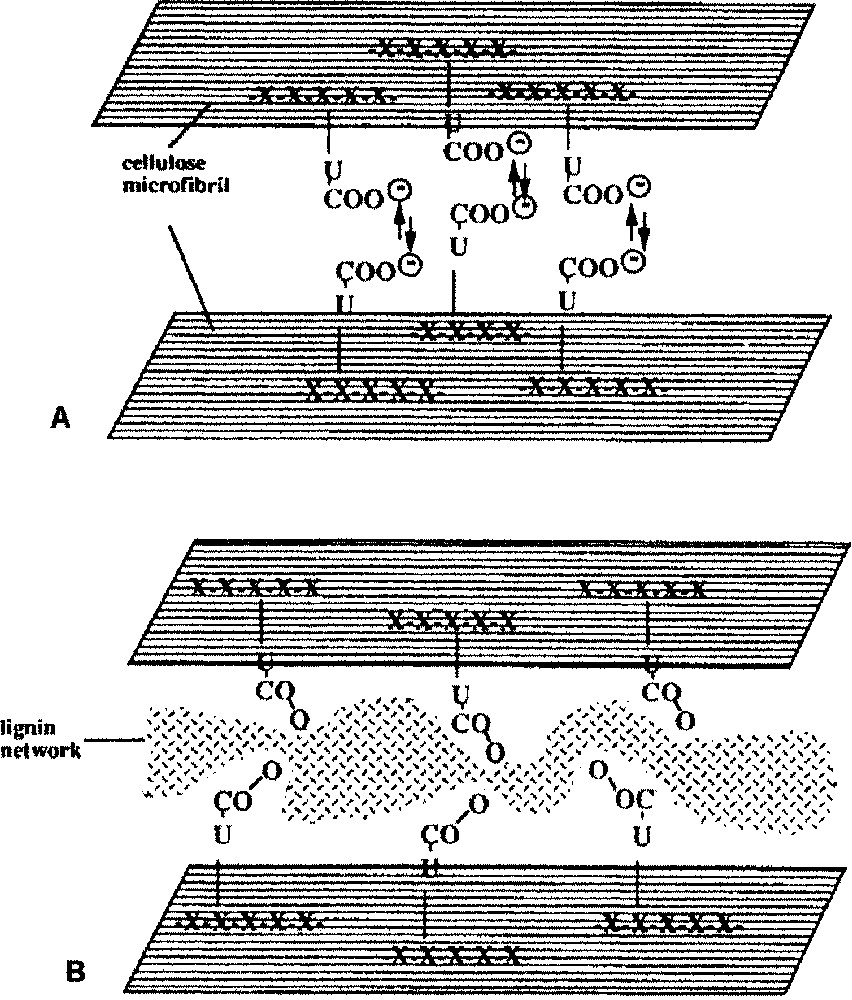
The glucuronoxylan–cellulose composite: possible successive steps of assembly and consolidation. A, Positioning and spacing pattern of the coated microfibrils, due to electrostatic repulsion of the acid coat; B, further consolidation by lignin intercalation.
Such co-crystallization of cellulose and GX led us to hypothesize that GX could direct the cellulose microfibrils in a helicoidal array [13]. This was a point that had to be explored.
4 Glucuronoxylans as twisting agents in helicoidal array
Experiments were tempted with quince slime, i.e. a helicoidal non-consolidated cell wall. This constitutes another paradigm model, since the walls remain completely fluid and cholesteric [6,14–16]. Attempts of in vitro reassociation of isolated cellulose/GX components from aqueous solutions were performed [14]. A spontaneous reassociation into cholesteric-like order was obtained from totally dispersed suspensions. Its structure was similar to the in situ native organization. When the system was deprived of GX, the cellulose flocculated, thus indicating the antifloc role of GX during assembly [15]. Glucuronoxylans possess surface charges and molecular structure (flexible side chains) that regulate fibrillogenesis as a selective moderator [17] and favour the formation of a cholesteric assembly. They could play a role of ‘twisting agent’, ‘helper molecules’, ‘molecular organizers’ or ‘lubricating agents’ driving the cellulose microfibrils into helicoidal rotation [16,18,19]. Fig. 12A shows how, in the early construction of cell wall, the face to face carboxylic groups of the GX could generate electrostatic repelling forces that prevent the aggregation of the microfibrils and favour their parallel alignment.
5 Concluding remarks
The foregoing data have focussed on the role of GX in the early assembly of helicoidal walls. It was tempting to consider their involvement in the following events, i.e. when lignins are progressively inserted in the pre-existing network at cell maturity. The cellulose/GX composite is a charged and highly anisotropic construction forming a sort of ‘host structure’ for lignin precursors. The incrustation of lignin can be compared to a form of intercalation chemistry where exogenous monomers can be intercalated, taking advantage of the gaps due to the internal electrostatic repelling, as documented for advanced industrial materials [20]. Here, lignin precursors are inserted and polymerised in the gaps extended between microfibrils, thus stiffening the xylan cellulose composite and safeguarding its initial geometry (Fig. 12B). In such a model, the GXs seem to play a key role in the successive steps of the morphogenesis of lignified secondary cell walls. Other plant systems have evidently cell walls constructed with other dominant hemicelluloses (arabinoxylans of grasses, glucomannans). The question remains open to understand whether they also could play a preeminent role in wall construction.