1 Introduction
May be the first historical example of suspected co-evolution is the synchronism between the circadian behaviours of a human parasite and its mosquito vector. This phenomenon prompted Manson, in 1877, to suspect, for the first time, that mosquitoes were involved in the transmission of a disease. Human lymphatic filariasis, a globally public health problem, is caused by two species of parasitic worms, the pantropical Wuchereria bancrofti and the Asiatic Brugia malayi (Fig. 1). Adult worms live in the lymph channels, where they cause the chronic disfiguring elephantiasis. They live up to ten years and females produce millions of larval forms or microfilariae (mff) that dwell several months in the blood system. Nocturnal periodicity occurs when mff only circulate in the host's peripheral blood during the night (Fig. 2A), at the time when night-biting mosquito vectors (rural Anopheles or urban Culex) may ingest them (Fig. 2C). During the day, the parasites stop in the lungs [1]. Nocturnal periodicity of W. bancrofti is observed in all tropical and subtropical countries, except for islands in the South Pacific and for the Nicobar archipelago, in the Indian Ocean (Fig. 2B), with diurnal, crepuscular vectors (Aedes, Fig. 2D). These mff are said diurnally subperiodic: they are always present in the bloodstream, but their density shows some increase in the daytime. From the first observation of subperiodicity, in 1895, many interpretations have been given, and for the majority of scholars subperiodicity was considered to be a degenerated form of periodicity. The other parasitic species, B. malayi, also exhibits two forms of periodicity [2]: a nocturnally periodic form transmitted by Anopheles, and a nocturnally subperiodic form (Fig. 2B), transmitted by swamp-forest Mansonia mosquitoes (Fig. 2E).
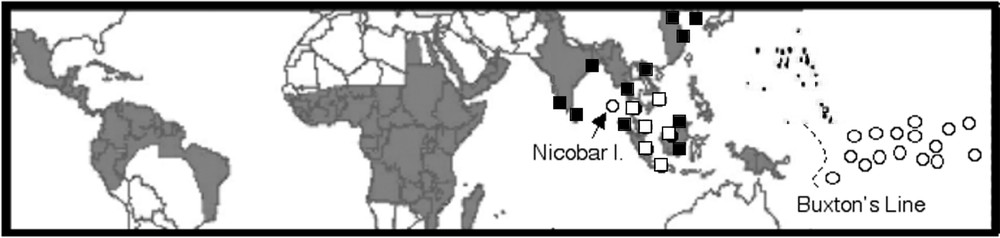
Distribution map of lymphatic filariasis. Global distribution of lymphatic filariasis endemic countries. Nocturnal periodic (dark background) and diurnal subperiodic (white circles) W. bancrofti. Nocturnal periodic (black squares) and nocturnal subperiodic (white squares) B. malayi. The Buxton's Line, between Vanuatu and Fiji archipelagos, is the eastern limit of Anopheles (and malaria) distribution, and, except for Nicobar Islands, the western limit of diurnal subperiodic Bancroftian filariasis (modified from WHO-GAELF [29]).
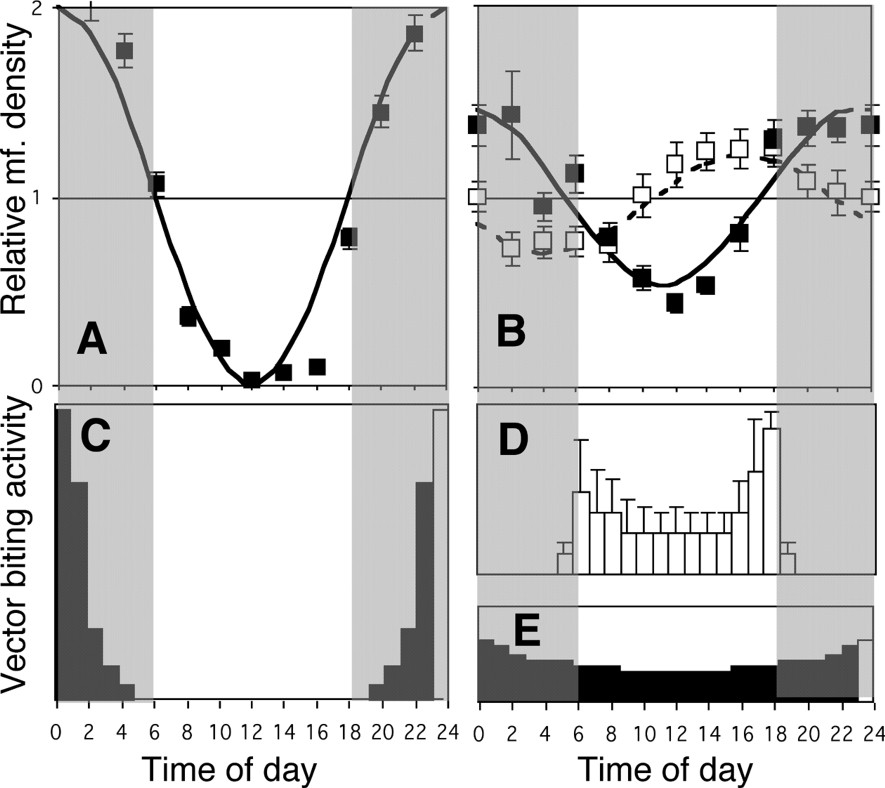
Periodicity, subperiodicity and cycle of vectors A–B. Observed bihourly mff relative densities (squares, with standard error), fitted by a cosine function (Eq. (1)). (A) Nocturnal periodic W. bancrofti (Philippines [35]): curve with parameters a=1 and k=0 h. (B) Diurnal subperiodic W. bancrofti (Tahiti [30]; white squares, interrupted curve, a=0.26, k=15.7 h), and nocturnal subperiodic B. malayi (Philippines [35]; black squares, continuous curve, a=0.46, k=23.1 h). C–E. Sketch of the biting cycles of main vectors of filariasis. (C) Vectors of nocturnal periodic filariasis: Anopheles and Culex. (D) Vectors of diurnal subperiodic filariasis: Aedes mosquitoes (subgenera Stegomyia and Ochlerotatus). The pattern of aggressiveness is highly variable (bars), depending on many factors. (E) Vectors of nocturnal subperiodic filariasis: Mansonia mosquitoes (in swamp forests).
This work, based on data from 83 individual biorhythms in seven localities (Table 1) is intended to understand the status of the different forms of subperiodicity. They are scattered over a distance of 11 000 km, between Marquesas and Nicobar Islands. The observed mean peak hours (hereafter expressed in decimals: 01:06 p.m. = 13.10 h) range from 14.46 h to 23.08 h. Is this circadian polymorphism due, as observed in schistosomes [3], to different mutations, locally selected to maximise the contact between parasites and their downstream hosts (i.e. vectors in the case of mff)?
List of the population samples of subperiodic filariasis
Sp.* | Code | Locality | N | Mean peak hour (SE) | Mean amplitude (SE) |
Wb | SA | Am. Samoa [30] | 4 | 14.77 (1.17) | 0.55 (0.110) |
Wb | TA | Tahiti [31] | 10 | 15.75 (0.93) | 0.38 (0.054) |
Wb | TO | Tonga [32] | 10** | 16.24 (0.99) | 0.36 (0.046) |
Wb | WA | Wallis [33] | 3 | 17.38 (0.91) | 0.28 (0.041) |
Wb | NI | Nicobar [34] | 7 | 17.92 (1.65) | 0.33 (0.045) |
Bm | MA | Malaysia [35] | 20 | 21.89 (0.86) | 0.38 (0.042) |
Bm | PH | Philippines [36] | 29 | 23.14 (0.48) | 0.58 (0.060) |
* Wb: Wuchereria bancrofti; Bm: Brugia malayi.
** 10 biorhythms on the same subject during 15 months.
Previous researches [4,5] suggested that subperiodicity is a mixture of periodic, out-of-phase biorhythms. This encourages taking into account individual host biorhythms rather than their hourly summation, which underestimates the low microfilaraemia individuals. Here we propose that subperiodicity is determined by a single mutation.
2 Methods
To obtain comparable data, all the observed chronological series are divided by the mean of the series. The resulting standardised series, of mean 1, are fitted, using maximum likelihood [6], by a two-parameter cosine function:
(1) |
The sum (or mean) of several individual rhythms with the same period at the same time is a cosine function with the same period.
Geometrically, a cosine function can be represented by a vector in polar coordinates of length a and of direction k.
3 Hypothesis
We put forward the hypothesis that the diversity of the global biorhythms observed in the different populations of subperiodic filariasis is ruled by two co-dominant alleles, D (for ‘day’) and d, which can combine into three biorhythm genotypes DD, Dd and dd. The predominant nocturnal periodicity, assumed to be genetically pure, is correctly fitted () by a cosine function (Fig. 2A) with amplitude and peak-hour (as usual, square brackets feature phenotypes). The other two elementary biorhythms described by and have unknown and peak hours. We also assume that the frequencies of the three elementary genotypes follow a Hardy–Weinberg law. A population biorhythm is the frequency-weighted sum of the three elementary biorhythms. It is proven that this sum is also a cosine function, of known parameters and :
(2) |
4 Geometrical model
This equation can be solved geometrically (Fig. 3A), replacing cosine functions y by vectors [7]. For a given population biorhythm, defined by the pair of values (vector ), the solution is a curve (Fig. 3B) linking every p-value (from 0 to 1) to a pair of peak hours . This geometrical procedure is reproduced in a model program, which is available. To test our hypothesis, at least three solution curves corresponding to three different populations have to be plotted. The types of expected results from the model are shown in Fig. 3C–E. Our hypotheses would be supported if all the curves intersect at the same point. The pattern in Fig. 3E corresponds to dominance.
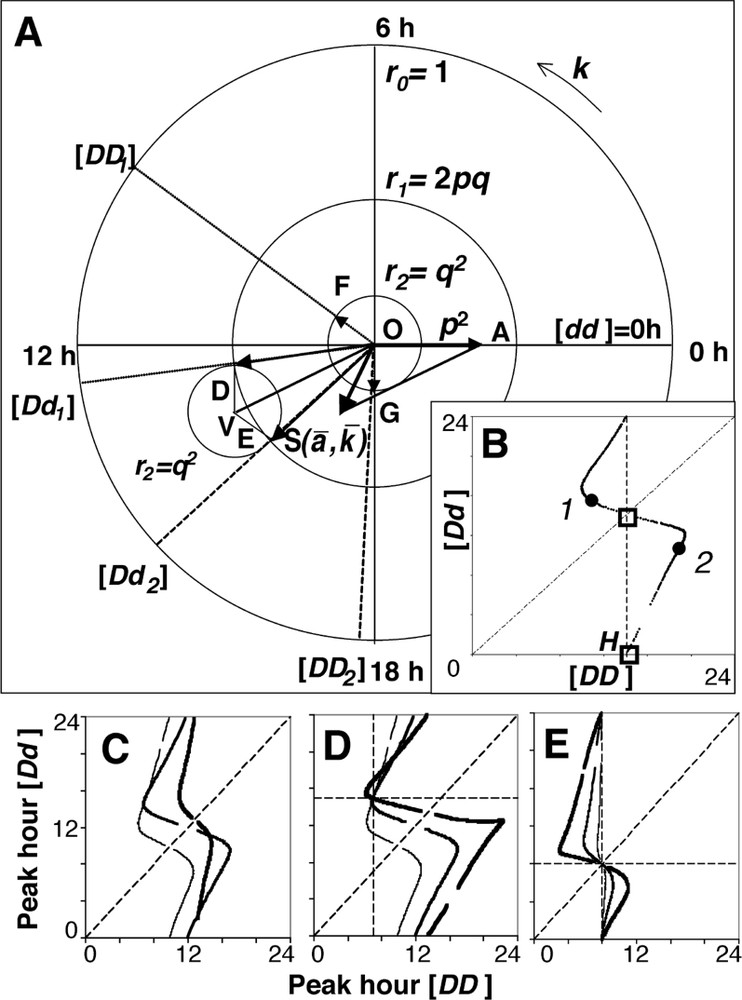
Geometrical approach. (A) Geometric solution of Eq. (2). For a given population biorhythm vector and a given p-value, generally two solution pairs of peak hours and satisfy Eq. (2). OASV, OFVE and OGVD are parallelograms. (B) These solution points (1 and 2) can be plotted on a graph with [DD] and [Dd] coordinates. We developed a model to reproduce the successive stages of this geometric procedure. For a given pair of values and , the program calculates, while incrementing p from 0 to 1 (0.01), all the solutions ([DD],[Dd]) and plots the resulting points drawing a sinuous curve. C–E. Types of results expected from the model (virtual solution curves). (C) No common intersection: the two-allele hypothesis is invalidated. (D) Common intersection: two alleles following the Hardy–Weinberg hypothesis are supported (three phenotypes). (E) Common intersection on the bisector line and for [Dd]=0 h. Ambiguous (D- or d-)dominance (two phenotypes).
5 Results: diallelism and dominance
We find that the seven solution curves, corresponding to the seven studied population biorhythms, can be considered to be intersecting at two points (Fig. 4). Our hypothesis of two common alleles following a Hardy–Weinberg law for all the studied parasites populations is supported. Both intersection points correspond to dominance, i.e. combination of only two phenotypes, (hereafter ) and . An ambiguousness is left concerning the heterozygous phenotype, the value of which is either equal to (D-dominance) or to (d-dominance). As we cannot mathematically distinguish either solution, we had to test their ecological implications.
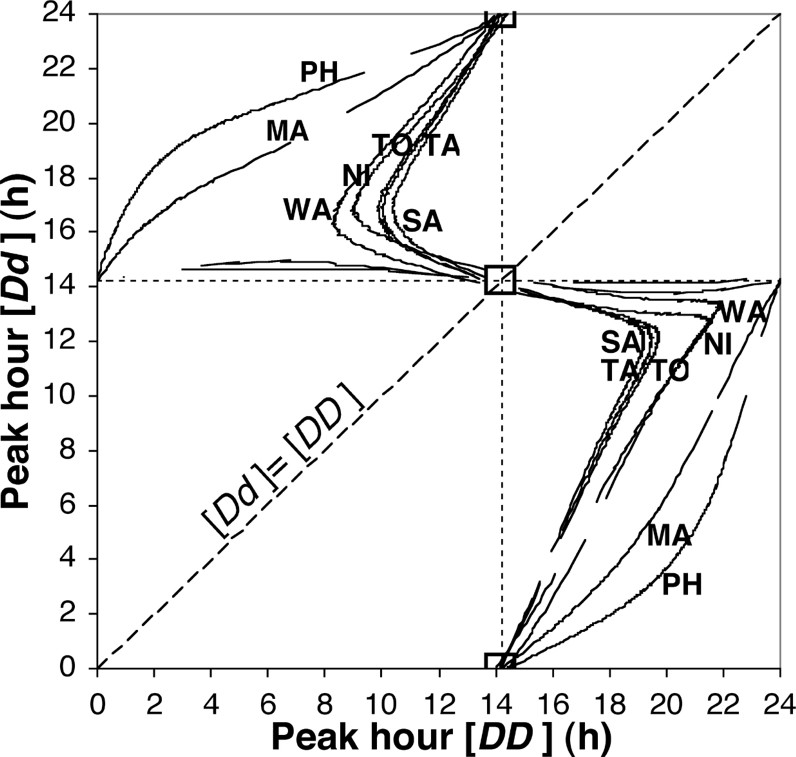
Geometrical solution. The seven solution curves corresponding to the observed population samples all intersect on the bisector line. In fact, they all cross a square with each side equal to one hour. The probability that this event is due to chance is 3×10−17. The biallelic Hardy–Weinberg hypothesis is supported. All the populations can be considered to be a combination of two phenotypes: [D]=H=14 h and [d]=0 h, but an ambiguity is left concerning the [Dd] heterozygous peak hour: [Dd]=[D] or [Dd]=[d]. Symbols: cf. Table 1.
6 Simulation model: parasitic allele selection by vector cycles
We developed the simulation model SelDom (for ‘select dominance’) taking into account the three biting-cycles of the main potential vectors (Fig. 2C–E)), assuming that each host receives five infective bites per day (figure voluntarily exaggerated), i.e. 10 000 bites per parasitic generation.
6.1 Description of the algorithm
- • Choose the type of vector hourly biting-cycle.
- • Starting with an arbitrary p-value, the three initial genotype hourly frequencies are calculated.
- •
For 1000 parasite generations (5000 years):
- ○ for each one of 10 000 vectors, the biting time is drawn from the chosen vector activity histogram and the corresponding genotype at this time is drawn;
- ○ the sums of the drawn genotypes allow calculation of a new p-value and new Hardy–Weinberg genotype hourly frequencies for the next generation;
- ○ if an allele is eliminated ( or ), the program stops.
If both alleles coexist , the vector activity cycle is supposed to be selectively neutral for the considered alleles.
6.2 Results of the simulation
Each type of dominance and of vector was run 100 times.
Mansonia transmission allows ‘indefinite’ (in fact 1000 generations) coexistence of both alleles, whichever kind of dominance may be.
If d were dominant, the D-allele would disappear in 41 generations (205 years) in the case of Aedes transmission, like in Polynesia. This region was inhabited 3000 years ago and the first European navigators, such as the Dutchman Abel Tasman (1643) noted presence of elephantiasis [8]. As the D-allele still exists, the d-dominant hypothesis has to be rejected. In case of Anopheles transmission, the D-allele would disappear in 750 generations.
On the other hand, if D were dominant, then both alleles would coexist indefinitely in the case of Aedes transmission, whereas D-allele would disappear in only four generations in case of Anopheles or Culex transmission. Thus D-dominance is supported.
Moreover, simulations show that a strictly daily periodic (i.e. pure DD) population could only occur in case of transmission by imaginary, ‘morning’ mosquito vectors. Actually, only nocturnal mosquito vectors, due to their narrow activity spectrum, play a selective role and can irreversibly eliminate the D-allele.
7 Discussion
The biallelic finding sheds new light on several theoretical and practical aspects of vector-parasite temporal relations.
7.1 Mathematical relations
7.1.1 Relation between amplitude and peak hour of parasitic populations
Knowing H (= 14 h) and setting , one can calculate the theoretical mean amplitude as a function of the mean peak hour :
(3) |
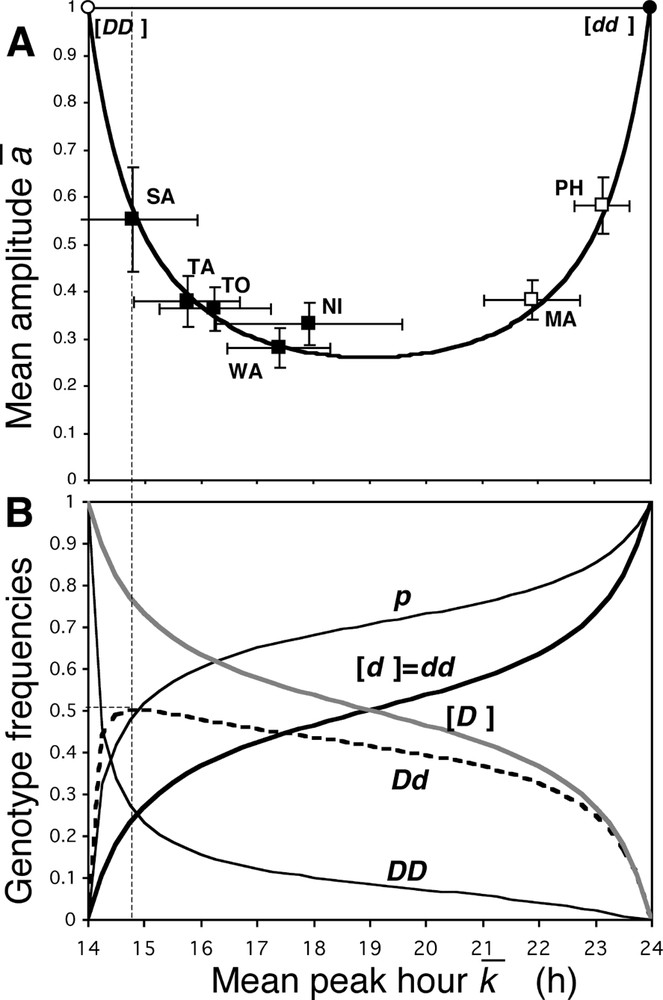
Mean amplitude and allele frequency as a function of the mean peak hour. (A) Theoretical (thick line; Eq. (3)) and observed (squares with standard errors; black squares; W. bancrofti; white squares: B. malayi) relation between the mean amplitude and the mean peak hour. (B) The thin line represents the theoretical frequency of the nocturnal recessive d-allele as a function of the mean peak hour (Eq. (4)). Other curves represent corresponding genotype and phenotype frequencies. Interrupted line corresponds to . Symbols: cf. Table 1.
7.1.2 Relation between d-allele frequency and peak hour of parasitic populations
One can also calculate the theoretical frequency of the d-allele as a function of the mean peak hour :
(4) |
7.2 Comments
7.2.1 Biorhythmic diversity explained
The 14 h-dominant and midnight-recessive allele hypothesis accounts for the diversity of biorhythms among the different W. bancrofti populations of Pacific and Indian Oceans (Fig. 5A). Moreover, it applies to the two parasite species responsible for lymphatic filariasis (W. bancrofti and B. malayi), which, although related, belong to two different genera. The D-allele is probably older than B. malayi speciation, and should also be found in older parasite species of Asiatic wild animals (carnivores and monkeys). In comparison with other circadian rhythms, and considering the large predominance of the nocturnal forms of filariae, we provisionally assume that the midnight gene is the wild genotype.
7.2.2 Possibility of a pure DD diurnal periodic population
In all the studied subperiodic populations, the frequency of the midnight d-allele is greater than (75% of parasites harbour at least one d-allele), corresponding to the theoretical peak hour (Fig. 5B). Although this point is very close to the pure (diurnally periodic) DD situation, it is unlikely to reach it through ‘bottlenecking’ because (a) the number of imported worms has to be very small (a group of 10 worms has a probability less than 10−4 to be only DD) and (b) such a small population of sexed worms has negligible chances to maintain itself. Thus the pure DD situation corresponds to a repulsion limit, whereas the pure dd situation corresponds to an absorption limit.
7.3 A scenario of parasite dissemination and speciation
The geographical success of W. bancrofti over B. malayi (which progressed gradually, with the expansion of agriculture) is probably due to Malayo-Polynesian horticulturists and sailors [8–10], who transported both alleles from swamp-forest estuaries of Southeast Asia over long distances, around 6000 years ago. They carried in their canoes Asiatic mosquitoes, ancestors of Ae. polynesiensis, the diurnal-crepuscular cycle of which allowed to maintain both alleles (Fig. 2D), and the eggs of which can endure desiccation and salinity [11]. Even with this efficient vector, filariasis would never establish in Polynesia without the D-allele. As they did not bring any convenient animal host, they selected W. bancrofti, a parasite, which, contrary to B. malayi, infected only man. In regions where nocturnal vectors were predominant (rice fields, urbanised areas), the D-allele was quickly eliminated and irreversible nocturnal periodicity emerged. This explains the large dissemination of this species in and beyond Asia.
7.4 A mutation common to several distant filarial genera
Extensive and methodical periodicity data on the nocturnal subperiodic Dirofilaria immitis, the dog heartworm [12], usually transmitted by nocturnal Culex, are also in close accordance with the midnight-2 p.m. allele hypothesis (, ). Dog should be an excellent animal model for conducting genetic studies on filarial periodicity.
The simulation model shows that a pure, DD, diurnal periodic population can only be selected by virtual ‘morning’ mosquito vectors. The African fly Chrysops silacea (Diptera: Tabanidae) exhibits a strictly diurnal activity cycle, with peak hour at 10 a.m. [13]. It is the main vector of the ‘eye worm’ Loa loa, a subcutaneous filaria of man described as diurnally periodic, the peak hour of which is found to be close to (, [14]). Moreover, in Duke's remarkable hybridisation experiments [15] with a monkey nocturnal strain, the parental and F1 strains are in accordance with a dominant allele determining a peak hour close to H (Student's test; ).
Extensive studies are presently conducted on Wolbachia pipientis, a bacterial endosymbiont, vertically transmitted, associated with arthropods and filarial nematodes. In filarial nematodes, W. pipientis has been shown to play an important role in the biology of the host and in the immuno-pathology of filariasis. Among the numerous filarial species found infected, W. bancrofti, B. malayi, and D. immitis have been shown to harbour these bacteria, but their absence in L. loa has been recorded in three recent studies [16]. Is this special feature related to the peculiar circadian behaviour observed in L. loa?
If the circadian genes of four biologically remote genera were homologous, this would confer on the D-allele a surprising seniority [17].
7.5 Comparison of appointment times in schistosomes and filariae
As regards the circadian emergence of free cercariae produced by aquatic snails [18], schistosomes seem to have a much greater genetic diversity than filariae. This is due to the shorter lifetime (some hours, as against several months for mff [19]), which demands a greater ‘precision’ to hit the downstream (mammal for cercariae, mosquito for mff) host. The standard deviation around peak emergence is close to 1 h, compared with 5 h for periodic mff.
The fact that each microfilaria has its clock set to midnight or to 2 p.m. suggests that each biorhythm, although largely autonomous and self-entertaining, sets itself to a different host time indicator [20,21]. The active phase (immobilisation in the lungs) starts at about 7 a.m. for nocturnal parasites, and at 9 p.m. for the diurnal ones.
8 Concluding remarks
The circadian per gene of Drosophila, encodes a protein of more than 1200 amino acids [22–25]. The filarial nematode gene containing this unique 14 h-mutation should be close to the gene encoding the timing protein LIN-42 of the nematode Caenorhabditis elegans [26], the genome of which has been completely sequenced [27]. This finding should accelerate the sequencing of filarial nematodes, mainly B. malayi, by the Filarial Genome Network. In-depth research on the biogenetic bases and localisation of this apparently stable circadian gene should be conducted.
A global programme for elimination of lymphatic filariasis (GPELF) was officially launched by WHO in 2000 [28]. Nowadays, genetic control against the vector-borne diseases is regarded seriously. One may speculate that, in the extensive regions (more than 90% of exposed population) where the midnight allele is pure, replacing it by the diurnal allele would drastically and durably reduce the transmission.
Acknowledgments
We thank Odile Bain (MNHN), Roger Webber (LSTMH), Gareth Witten (Capetown University), Ovide Arino, John Measey and Vincent Robert (IRD), for comments and discussion on an earlier draft of the manuscript. In homage to William A. Robinson, scholar in Polynesian navigation and filariasis and founder of the ‘Louis Malardé Institute’ the Tahitian Fare Mariri (House of Filariasis).