1 Introduction
Caffeine (1,3,7-trimethylxanthine or 1,3,7-trimethyl-2,6-dioxopurine or 3,7-dihydro-1,3,7-trimethyl-lH-purine-2,6-dione), is the most widely consumed behaviourally active and stimulant substance in the world, almost all coming from dietary sources [1–5]. Several studies showed that daily caffeine intake from coffee, tea or soft drinks increases alertness and activity in subjects and improves cognitive functions, including vigilance, learning, memory, and mood state. It was also shown that job performance was restored in individuals when bored or fatigued and after night work and sleep deprivation [2,6–11]. However, important individual variations in the tolerance to caffeine effects on sleep and waking were reported, attributed not only to study design but to the subjects' age, sex, physiology, and consumption habits [12]. Increase in sleep onset latency, sleep disruptions and the effects on wakening the following day were studied after nocturnal caffeine intake as a model of insomnia, showing contrasting effects depending on the dose administered [12], but consistent individual differences in sensitivity to caffeine were shown [13].
The mechanisms underlying the differences in the sensitivity to the effects of caffeine on sleep is not well known. It is suggested that these differences reflect both interindividual variations as well as day-to-day variability in the subject's response [14–16]. Another explanation may be the development of tolerance on sleep at the level of caffeine consumption. This proposal is supported by the observation that caffeine tends to have less effect on the sleep of the heavy consumer [17], and that no major differences were observed in plasma caffeine levels in relation with individual sensitivity after the ingestion of a 300-mg dose [13]. However, it may be possible that metabolic polymorphism of caffeine elimination, attributed to genetic or/and environmental origins, modify caffeine pharmacokinetics and elimination and thus its psycho stimulant effects [18].
Therefore, this study has been undertaken to compare pharmacokinetic and electro-physiological responses to caffeine in two different groups of four healthy volunteers: sensitive (S) and non-sensitive (control: C) subjects to caffeine psycho stimulant effects.
The reversed-phase HPLC method was developed to measure plasma caffeine concentrations and thus to determine caffeine pharmacokinetic parameters.
The assessment of vigilance level and brain activity was provided by a topographic quantitative EEG measurements.
2 Materials and methods
2.1 Subjects
This study was carried out at the Faculty of Medicine (Biophysics Laboratory) and at the Central University Hospital (Functional Nervous System Exploration Department) Sousse (Tunisia).
The Ethical Committee of the Faculty of Medicine approved the study and each volunteer gave his informed consent. Volunteers were not paid and were recruited from the staff of the Faculty of Medicine through the university newspaper. An initial interview of the volunteers enabled the selection of the subjects sensitive to psycho stimulant caffeine effects. Two groups of four subjects were constituted, based on their self-rated insomniac (S) and non-insomniac (C) nocturnal behaviour after caffeine intake. All volunteers were healthy males with neither psychiatric history nor taking psychotropic medicines, and were regular consumers of coffee. The mean ages (± SD) of C and S subjects were (ranged: 29–45 years) and (ranged: 31–48 years), respectively. Body weights were and and body mass index (BMI) were and for C and S group, respectively.
All recruited subjects were not allowed to consume caffeine-containing beverages for at least 36 h before and during the test. After an overnight fast, the subjects came at 8:00 in the morning to the laboratory hospital. An ambulatory electroencephalographic (EEG) recording started at 8:30 for the measurement of brain activity during a period of 6 h. At 9:00, each subject ingested 140 mg caffeine dissolved in 150 ml of warm water. A light post-dose meal containing fresh vegetables and a piece of bread was provided to volunteers.
2.2 Methods
The selected healthy volunteers filled a questionnaire where the total consumption of coffee, tea, chocolate and cola beverages intake was recorded.
They were questioned on their perception of their sensitivity to caffeine taken late in the afternoon, particularly whether or not they experienced nocturnal insomnia, a delay to sleep onset, any other effects or no effect at all.
An approximate estimate of daily caffeine consumption was calculated using the following caffeine contents: instant coffee 85 mg/cup; filter coffee 100 mg/cup; tea 65 mg/cup; decaffeinated coffee 3 mg/cup; cola 18 mg/6 floz; chocolate 1 mg/g [1,19,20].
Tobacco consumption was also evaluated and for smokers, the number of cigarettes smoked daily was recorded.
Plasma samples (5 ml) were collected directly in an EDTA tubes before caffeine administration () and after 0.5, 1, 2, 4, 6 and 24 h post-dose.
2.3 Plasma HPLC analysis of caffeine concentrations
Ammonium sulfate (600 mg) was added to 1 ml plasma immediately after its collection from the subjects. After 1 minute vigorous shaking (Wortex), a liquid–liquid extraction was applied to the samples using 6 ml of a solution containing ethyl acetate and isopropyl alcohol (8/1; v/v). After vigorous shaking for 2 min, the sample was centrifuged at 6000 g during 20 min. Five millilitres of the organic phase was collected, dried at 60 °C under a flow of nitrogen gas and the residue was dissolved in 100 μl KH2PO4, the solvent used as the initial mobile HPLC phase. HPLC separation (HP 1500, Hewlett Packard; Waldbronn, Germany) was performed using a C18 reversed-phase column (Vydac; Hesperia, USA) maintained at 33 °C with a direct injection of 20 μl of the prepared sample. A gradient was used with two mobile phases A and B.
The mobile phase A was a buffer KH2PO4 (15 mM, pH 4.9) and the mobile phase B was acetonitrile. The elution program was chosen as follows: B was set at 5% for the first 8 min, then increased linearly to 10% at 11 min, then to 15% at 15 min and reached the 60% from 15 to 25 min.
Plasma caffeine concentrations were calculated by CHEMSTATION software, using a caffeine calibration curve established by plotting the peak area ratio versus internal standard concentrations and the best relationship was determined by a least-square linear regression analysis. The correlation coefficient () of the calibration function obtained was higher than 0.999. The coefficients of variations of replicate analysis of samples containing 2.5, 5 and 10 μg/ml were respectively 2.80, 2.33 and 1.52%.
2.4 Measurement of caffeine pharmacokinetics
Caffeine pharmacokinetic parameters such as maximum plasma caffeine concentration () and time to reach maximum concentration () were determined directly by visual inspection of the plasma caffeine concentrations curves. Caffeine half-life () was calculated by mono-compartmental analyses using least squares regression analysis.
2.5 Electroencephalographic measurements
The assessments of vigilance level and brain activity responses to caffeine were provided by topographical quantitative electroencephalogram (EEG), which records electrical activity in the outermost layers of the cerebral cortex via electrodes attached to the scalp. The frequency, amplitude and distribution of waveforms were monitored. The EEG was recorded from 8 bipolar derivations silver/silver chloride gel-filled scalp electrodes. Centro-occipital right and left derivations (C4-O2 and C3-O1) were used for this study. During the EEG recording, subjects for both groups were placed in the same conditions (laboratory room) and they were free on their movements. EEG signals were amplified with an OXFORD MEDILOG 9000 apparatus and all EEG signals were scanned every 32 seconds and analyzed with Fast Fourier Transformation during 6 h after caffeine ingestion. In the resulting spectrum, six frequency bands were defined: delta1 (0.7–2 Hz), delta2 (2–4 Hz), theta (4–8 Hz), alpha (8–12 Hz), beta1 (12–18 Hz) and beta2 (18–30 Hz). Percentage of absolute power values (μv2) was calculated for the frequency bands and the entire spectrum (0.7–30 Hz) before (H0) and after 1 h (H1), 2 h (H2), 3 h (H3), 4 h (H4), 5 h (H5) and 6 h (H6) of caffeine intake.
2.6 Statistical analysis
The Mann–Whitney U-test was used for comparisons of data with a normal distribution. Analyses of variance (ANOVA) and T test were used for inter-group comparison of EEG mean values.
3 Results
3.1 Caffeine consumption
The estimation of daily caffeine consumption from the questionnaire was significantly different in the two groups (), the mean total caffeine consumption reported by C subjects was () and that of S subjects was ().
Caffeine intake was provided essentially from coffee (87.4%), while tea represented 12.5% of total consumption.
3.2 Cigarette smoking
There was no subject smoking in the insomniac S group, while in the C group they were all smokers with a mean number of 28 cigarettes per day (range of 20–35).
3.3 Caffeine plasma concentration and pharmacokinetics
In all subjects, caffeine concentrations before its administration were below the detection limit (30 ng/ml). The average caffeine plasma concentration reached a maximum about 30 min after caffeine administration with a mean concentration of 7.80 μg/ml and decreased after 4 h to a mean value of 4.45 μg/ml.
Pharmacokinetic measures (, and ) for each group are shown in Table 1. There are wide variations for all volunteers in mean plasma caffeine concentration, ranging from 4.45 to 10.67 μg/ml. The average calculated for insomniac subjects (S) was and for non-insomniac ones (C) and these values are significantly different ().
Caffeine pharmacokinetic measures ( and )
Plasma caffeine kinetics | Sensitive subjects [S] | Non-sensitive subjects [C] |
(μg/ml) ± SD | 9.1±2.871 | 7.29±2.60 |
(range: 4.45–10.67) | (range: 5.04–10.34) | |
Half-life (h) ± SD | 7.17±0.781 | 3.81±0.14 |
(range: 6.85–8.04) | (range: 3.80–3.96) |
1 Significant differences between S and C .
For all the subjects, is reached 30 min after caffeine ingestion, except for one volunteer in the C group, where was reached 120 min post-dose.
Caffeine half-life values calculated from the peak value up to the last measurement performed after 24 h were significantly greater in the S group () when compared with the C group () ().
Caffeine plasma kinetics for S and C groups is shown in Fig. 1. While the curves were similar on the first 6 h after caffeine administration, there is a significant increased elimination later in the C group so that the last measurements obtained after 24 h were significantly different in the C and the S groups, respectively ( and ; ). This higher caffeine clearance explained a two-fold faster caffeine half-life in the C group when compared with the S group.
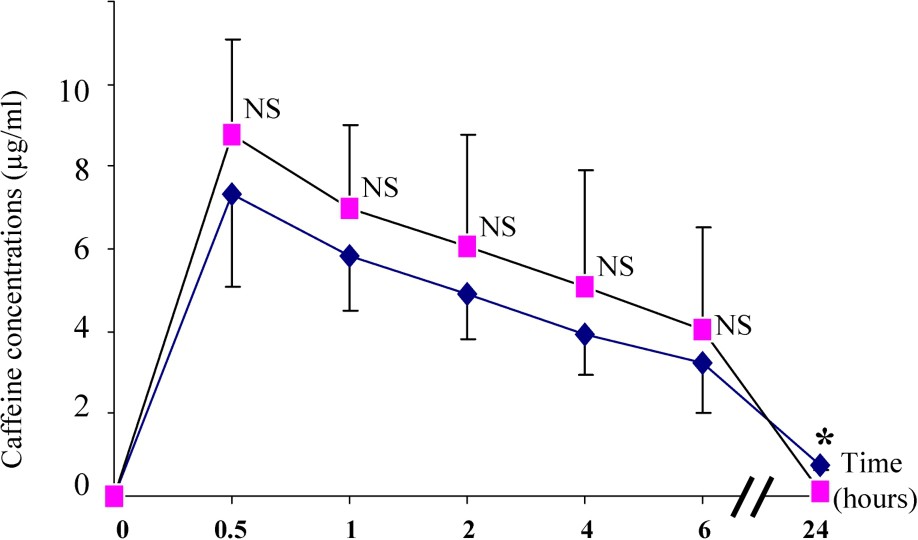
Plasma caffeine concentrations in S (♦–♦) and C (■–■) subjects. Differences between the S and C groups: NS (non-significant) and * (p<0.05).
3.4 Electro physiologic results
Spectral analyses of total EEGs measurements performed on all subjects showed that there is an increase in beta and alpha frequency power after caffeine ingestion compared to baselines (pre-dose), whereas theta and delta power were respectively reduced (Table 2).
Mean percentage ± SD of six power frequency bands at different times after caffeine ingestion
Band | % Power frequency ± SD | |||||
Hour | ||||||
H0 | H1 | H2 | H3 | H4 | H5 | |
Delta1 | 63.0 ± 13.0 | 61.9 ± 12.9 | 58.0 ± 11.2 | 56.0 ± 15.7 | 50.9 ± 12.7 | 58.6 ± 11.0 |
Delta2 | 14.9 ± 1.7 | 12.3 ± 7.2 | 12.2 ± 2.6 | 11.4 ± 3.6 | 10.9 ± 4.5 | 11.6 ± 5.41 |
Theta | 7.2 ± 1.3 | 6.9 ± 3.0 | 5.8 ± 2.0 | 6.3 ± 2.0 | 5.7 ± 1.7 | 7.4 ± 2.2 |
Alpha | 2.7 ± 1.2 | 3.0 ± 4.5 | 4.6 ± 2.2 | 5.1 ± 4.2 | 5.5 ± 2.8 | 5.0 ± 4.7 |
Beta1 | 4.7 ± 2.9 | 4.6 ± 2.1 | 5.8 ± 2.2 | 6.1 ± 2.6 | 6.7 ± 3.1 | 5.5 ± 2.7 |
Beta2 | 13.9 ± 4.4 | 20.3 ± 16.7 | 22.3 ± 10.9 | 22.9 ± 21.3 | 22.9 ± 13.7 | 22.1 ± 23.71 |
1 with .
As shown in Fig. 2, caffeine reduced delta frequency and increased beta power for all time points.
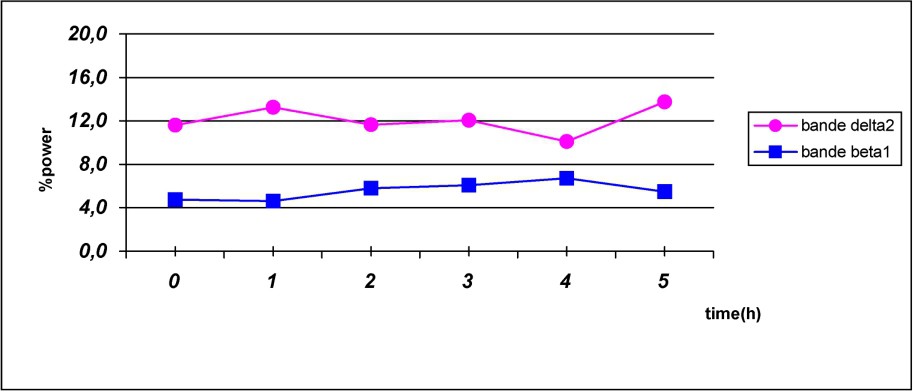
Fast- and slow-frequency bands evolution following caffeine intake.
A difference in the effect of caffeine on brain activity between two groups was observed on the fast band and in the slow band. This difference is significant between delta and beta frequencies measured before and at five hour after caffeine intake ( between H0 and H5). According to the ANOVA test, there is no other significant difference between two groups in beta and delta frequency evolution at all time points ().
In both groups, Fig. 3 shows that beta power increase just after caffeine intake, reach a maximum on the next three hours, then rapidly decrease to return to baseline value in the C group. However, high level of beta power is maintained over the five hours after caffeine ingestion in S subjects.

Mean values of power beta frequency in S (1) (■–■), and C group (2) (♦–♦) after caffeine dosage. p<0.05 at H5.
The decrease of delta power was initially similar in both groups as shown in Fig. 4, but it persists more in the S group when compared to C group.
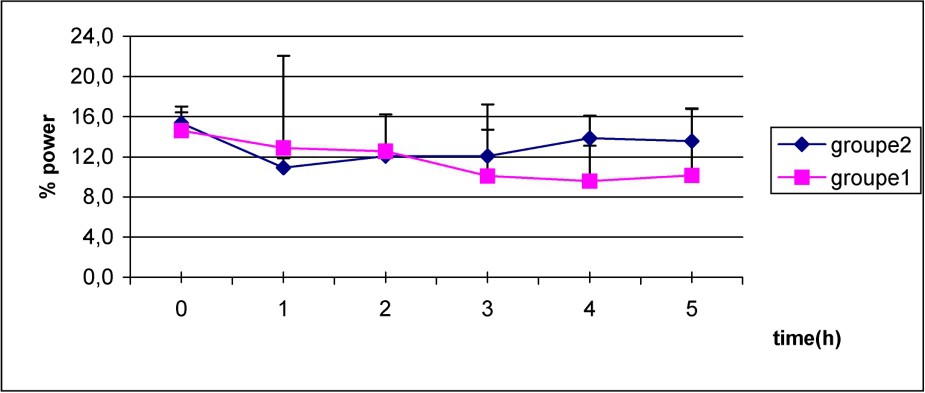
Mean values of power delta frequency in S group (1) (■–■), and C group (2) (♦–♦) after caffeine dosage. Non-significant differences.
4 Discussion
This study showed that healthy subjects without sleep disorders after consuming caffeine-containing beverages in the late afternoon exhibit pharmacokinetics and electroencephalographic different responses from those reporting disturbed sleep quality after caffeine ingestion.
The differences observed in caffeine pharmacokinetic parameters could explain the sleep disturbances reported. It is the case for the significant higher maximum plasma caffeine concentration observed in S subjects when compared with C subjects . There is however no difference in , showing that absorption kinetics are not delayed. One subject showed a higher value at 120 min, but previous studies have shown that peak plasma caffeine concentrations may occur between 15 and 120 min after oral ingestion [21–23]. This wide variation may be due to delayed gastric emptying and other unknown individual environmental and physiological variations.
The intolerant or the sensitive S subjects showed also significant variations in caffeine half-life. Caffeine elimination is slower in subjects with sleep disturbances compared to tolerant subjects. Intolerant subjects have elimination half-lives between 6.5 to 8 h and as a consequence, significant plasma caffeine concentrations may persist in these subjects until night when caffeine is consumed in the late afternoon, delaying sleep onset and the quality of sleep.
These findings are consistent with previous studies [24,25] showing that caffeine half-life was longer and plasma concentration higher in poor sleepers.
Except for a dose-dependent pharmacokinetics in human, caffeine ingestion had no inducing effect on caffeine clearance [18]. Caffeine metabolism involves several simultaneous pathways, the first step being demethylation into dimethylxanthines: paraxanthine, theobromine and theophylline. The most important metabolic pathway in human is the successive demethylation of caffeine into paraxanthine and 1-methylxanthine while the mechanism of the formation of 5-acetylamino-6-formylamino-3-methyluracil (AFMU) is still unknown [11]. These pathways involve the activity of hepatic cytochrome P450, CYP1A2 enzyme. CYP1A2 is genetically polymorphic enzyme, influenced by a variety of factors, including individual behaviour, such as smoking habits, which is shown to induce CYP1A2 enzyme activity [26,27]. Because the demethylation into paraxanthine selectively catalyzed by CYP1A2 is the rate-limiting step in caffeine metabolism, individual variability [28] as well as its inhibition or stimulation will determine the rate of caffeine clearance in humans [11].
The present study showed that caffeine metabolism was faster among non-sensitive subjects and this was presumably due to polymorphism of this enzyme and more particular to cigarette smoking in this group which are all smokers' subjects (mean of 28 cigarettes/day). In fact, several studies have been carried out on the effect of tobacco on CYP1A2 activity. Kalow et al. [26], Murphy et al. [29] and Pelkonen et al. [30] found that smokers presented an increase of CYP1A2 activity compared to that of non-smokers and ex-smokers. As a consequence, smoking cigarettes tends to increase the demethylation rate of caffeine [17,30]. It has been shown that a 12-week smoking cessation leads to a 269% increased plasma caffeine levels [31]. Our subjects were studied after an overnight fast and smoking abstinence and they were allowed to resume cigarette smoking at 3:00 pm, 6 h after caffeine administration. This period is considered not long enough to normalize the enzyme-inducing effect of cigarette smoking observed after 4-day abstinence [32]. However, we are not aware of any studies investigating shorter period of time. This study shows similar caffeine kinetics on the first 6 h after the administration in both groups followed by an increased plasma clearance on the next 6–24-h period in the C group when smoking was resumed. Although, the important individual variations observed would need a larger number of subjects, these preliminary results suggest for the first time that smoking overnight abstinence and resuming smoking appear to inhibit and induce respectively the hepatic cytochrome activity in only 15–18-h time delay.
Caffeine effects on brain activity given by EEG power spectra, showed an increase of power fast wave with a decrease of power slow wave after caffeine ingestion compared to baselines (Table 1, Fig. 2).
These EEG changes are consistent with previous studies showing that caffeine increases vigilance level. These results are in agreement with those reported by Landolt et al. [33], showing that a lower dose of 100 mg caffeine administered at bedtime and compared to placebo prolonged sleep latency and reduced slow-wave activity, mainly at the lowest delta band in healthy young students. With a caffeine ingestion of 200 mg taken in the morning, they reported rather small effects on sleep and similar effects on the spectral power density of the sleep EEG in the subsequent night, although of smaller magnitude [34].
Following caffeine ingestion, EEG spectra power did not significantly differ between the two groups. This lack of effect is explained by a non-significant difference due to a too small number of subjects. However, in spite of the absence of difference in EEG between the two groups on caffeine administration, subjective insomnia and prolonged sleep latency reported in S subjects after caffeine consumption in the evening were in accordance with EEG measurements (Fig. 3). In addition, these EEG measurements also show that the effects of caffeine on brain activity or vigilance level were more potent and prolonged in the subjects of the S group than those of the C group.
These EEG results may be affected by smoking as power values of theta was decreased and fast wave alpha power increased on repeated nicotine inhalation [35]. This study showed that EEG measurements were different between smokers submitted to an overnight smoking abstinence, non-smokers and smokers required to smoke. In our study performed on smokers after an overnight abstinence and non-smokers, an increased power values of theta in abstaining smokers compared to the non-smokers and a decreased of power values alpha may be additional to the effect of caffeine. However, in young non-smoking subjects, caffeine was only shown to reduce the power density in the lowest delta band in contrast to the changes during physiological sleep were both delta and theta bands are concerned [33]. In case of insomnia, more beta and less alpha frequencies are observed [13].
These S subjects characterized by a poor caffeine metabolism, exhibit longer caffeine elimination and have, as a consequence, a greater exposure to the stimulant effect of caffeine. They are thus more sensitive to the psycho stimulant effects of caffeine. In this study, the intolerant subjects exhibit a slow caffeine metabolism and a prolonged caffeine effect on the 5 h after the ingestion (Figs. 3 and 4). Some variation in the sensitivity to the effects of coffee and caffeine on sleep could be attributable to tolerance but, as discussed by Fredholm et al. [5], this mechanism is not yet clearly established.
In the S subjects, caffeine plasma concentrations persists longer due to a reduced metabolism, elimination and excretion and may reach a significant level at bedtime, contributing to prolonged sleep latency and sleep disorders. It is shown that caffeine intolerant subjects consume less caffeine compared with the other subjects. Intolerant subjects may reduce their caffeine consumption due to their susceptibility to caffeine effects.
Finally, the results of the present study suggest that, in addition to the total caffeine intake, plasma caffeine concentrations and elimination are affected by CYP1A2 enzyme system, which appears to explain some of the difference in the sensitivity to caffeine on sleep in sensitive subjects. In these individuals, caffeine consumption must be reduced, especially in the late afternoon or in the evening, to avoid sleep disturbances.
Acknowledgements
We wish to express our gratitude to Prof. Bougahttas Naceur (Chronobiology Laboratory of the Medicine Faculty, Monastir, Tunisia).