1 Introduction
Plasmodium infection starts when an infected Anopheles mosquito, while probing the host in search of a blood source, ejects parasites into the dermis. At this stage of its life cycle, the parasite is a sporozoite, an elongated and actively motile cell whose final destination is the liver. Inside hepatocytes, the parasite multiplies and transforms into the erythrocyte-invasive form, which initiates the parasite replication cycles inside erythrocytes and the clinical symptoms of the disease.
The so-called pre-erythrocytic stages of Plasmodium, i.e., the sporozoite and the liver stages, have long been recognized as attractive targets for anti-malarial vaccines. As was first discovered more than 60 years ago using an avian Plasmodium model of infection, and is now well established in rodent [1] and primate mammalian models as well as in humans [2], injection of several thousands of irradiated sporozoites induces a robust and lasting immune response in the host that can protect against subsequent injection of virulent sporozoites. Since irradiated sporozoites normally reach hepatocytes, but fail to develop into erythrocyte-infecting forms (they fail to undergo normal nuclear divisions), such a live, attenuated vaccine would aim at preventing erythrocyte infection and the onset of the disease. However, such a vaccine remains experimental, because thousands of mosquito bites are required to deliver the protective dose of irradiated sporozoites, and the latter, once dissected out from mosquito salivary glands, rapidly lose their infectivity and cannot be stored.
In the last twenty years, the nature of the host immune responses initiated by irradiated sporozoites has been under intense scrutiny [3]. Much of what we know on these protective responses comes from studies using the P. berghei and P. yoelii species, which infect rodents. The primary effector cells of protection induced by irradiated sporozoites are thought to be CD8+ T cells [4], which recognize parasite peptides presented on the surface of infected hepatocytes in association with MHC class-I molecules. The mechanisms underlying the CD8+-mediated effects are not completely understood, but IFN-γ, IL-12, and nitric oxide, an effective killer of the Plasmodium liver stage, play central roles. Recently, the demonstration that protection can also be induced by genetically modified sporozoites unable to complete their development in the host liver [5,6] has renewed interest in developing live attenuated vaccines [7,8]. However, if such a live sporozoite vaccine is to be pursued, it is particularly important to know the exact route that sporozoites take from the site of mosquito bite to hepatocytes, as well as the nature of the host–parasite interactions along the way.
A quantitative and real-time view of sporozoite infection in vivo is now possible due to recent advances in imaging techniques and to the generation of transgenic parasites that express fluorescent tags. Using clones of P. berghei that express the green fluorescent protein [9,10], we have investigated by epifluorescence time-lapse microscopy the fate of sporozoites naturally transmitted by Anopheles stephensi mosquitoes [11].
We first imaged sporozoites in the dermis of the host. For this, a single mosquito was allowed to probe the ear of an anesthetized hairless mouse for 1 min, and the probed site was observed by wide-field or confocal microscopy. An average of ∼20 fluorescent sporozoites was detected in the imaged tissue volume. Approximately 75% of them were gliding in the dermis (upon observation intervals of 10 s), and displayed a robust forward locomotion (at speeds of 1–2 μm s−1) that followed a tortuous and apparently random path (Fig. 1). The motility of dermis sporozoites therefore differs from the motility of sporozoites in vitro (on coverslips), where sporozoites glide at similarly high speeds, but in an almost perfectly circular fashion, as well as from the motility of sporozoites in the mosquito salivary ducts, where only 10% (upon observation intervals of 100 s) glide at much slower speed () and in a typically back-and-forth fashion [12]. However, despite their strongly induced motility, most dermis sporozoites remained in the imaged volume over time, due to both their sinuous gliding path and declining average velocity (Fig. 1). This allowed a quantitative assessment of the sporozoite fate in the dermis.

Sporozoite motility in the dermis. The figure shows the dispersion of sporozoites in the dermis, represented by maximum fluorescent intensity projections of parasites moving over 400 s at 5 min (red), 16 min (green) and 48 min (blue) after the mosquito bite. The movement of most sporozoites is localized at the site of bite and decreases over time.
We then addressed how sporozoites were leaving the site of mosquito bite. Blood vessels were revealed by tracking the movement of erythrocytes with transmission light at high image acquisition rates prior to or after imaging fluorescent sporozoites, or the blood flow was tracked by intravenous injection of fluorescent bovine serum albumin (BSA). As already described [13], sporozoites invaded blood vessels. Sporozoites gliding in the dermis were seen interacting with blood vessel walls, frequently decreasing their speed when gliding along them (Fig. 2), before being suddenly carried away at speeds similar to that of erythrocytes in the dermal capillary bed, i.e., .
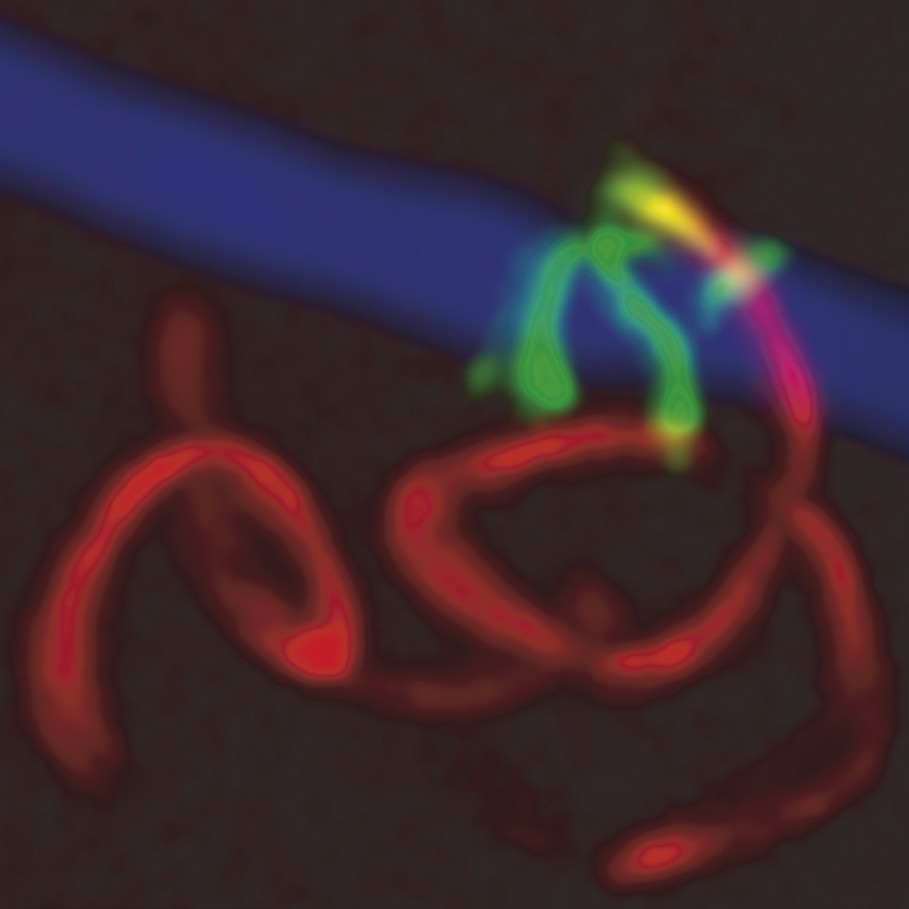
Sporozoite invasion of a blood vessel in the dermis. The maximum intensity projection shows a sporozoite gliding in the dermis (red; 20 confocal images over 120 s) and gliding along the blood vessel wall (green; 25 confocal images over 150 s) before invasion. The blood vessel, tracked by fluorescent BSA, is depicted in blue.
We also observed, for the first time, sporozoites invading lymphatic vessels. Sporozoites gliding in the dermis anterior pole first were subsequently seen drifting in a sideward and low-velocity movement along a path that did not coincide with the trajectory of a blood vessel. Confocal microscopy after intra-dermal injection of sporozoites with red fluorescent dextran confirmed the presence of sporozoites inside lymphatics. Based on data collected from 1-h-time-lapse analysis of dermis sporozoites delivered during 12 independent mosquito bite experiments, we estimate that in 1 h of the inoculated sporozoites leave the site of bite by invading either blood () or lymphatic () vessels. Unexpectedly, an important proportion of the sporozoites remained in the dermis, where they were detected up to 7 h post-injection.
The traditional assumption is that sporozoites potentially drained by lymphatic vessels would eventually reach the blood and the liver [14,15], where they can invade the only cells in which they are known to develop in vivo, i.e., hepatocytes. To analyze sporozoites in the lymphatic system, 10 infected mosquitoes were allowed to bite for 1 min the footpad of anesthetized hairless mice, and at various times post-bite the proximal (popliteal) and distal (iliac) draining lymph nodes were dissected to determine the number of fluorescent sporozoites. An average of ∼50 parasites was found in the popliteal node at 1 h, 2 h, and 4 h post-bite, representing of the inoculated sporozoites. In the iliac node, however, sporozoites were not encountered up to 4 h post-bite. Similar results, i.e., a very small proportion of inoculated sporozoites found beyond the popliteal lymph node, were also obtained using C57/Bl6 mice and in Brown–Norway rats, which are both highly permissive to P. berghei. We conclude that most of the sporozoites drained by lymphatics stop their journey in the proximal lymph node. Importantly in the context of a potential live sporozoite vaccine, we also found that a ∼20-fold higher proportion of sporozoites reached the popliteal node when sporozoites are injected by mosquitoes than intra-dermally with a syringe.
We next investigated the fate of sporozoites in the popliteal lymph nodes. Early after injection (1–2 h), sporozoites were found mainly in the sub-capsular zone of the lymph nodes close to the afferent lymph vessel and sub-capsular sinuses, but re-localized towards the efferent vessel over time. In the process, the proportion of parasites displaying a normal shape sharply declined. While at 1 h most parasites displayed the characteristic sporozoite shape, at 4 h most parasites appeared damaged, and at 8 h most fluorescence had disappeared. When extracted lymph nodes were stained with antibodies to CD11c, a marker specific of dendritic cells (DC), and of the lymph node parasites were closely associated with DC at 2 h and 4 h post-bite, respectively. Sporozoites were either extracellular, with the surface areas of the two cells apposed, or were detected inside DC as normally shaped or partially degraded parasites.
Most surprisingly, examination of lymph nodes over time revealed that lymph sporozoites could mature into exo-erythrocytic forms (EEF). Normal development of P. berghei inside rodent hepatocytes takes , and consists in a remodelling process from the elongated sporozoite to an enlarged and spherical EEF, in which nuclei divide and from which daughter cells eventually bud off. To investigate parasite development in the lymph nodes, the footpads of C57/Bl6 mice were subjected to 15 mosquito bites or to intra-dermal injection of 105 salivary gland sporozoites. Following delivery by mosquito or syringe, a small proportion of fluorescent parasites was detected in the lymph node at 15 h and 24 h. The location of these parasites inside lymph nodes was investigated by staining with antibodies to CD11c; CD11b, a macrophage/monocyte marker; CD45, a generic leukocyte antigen; and podoplanin, which is specifically expressed by lymphatic but not blood vascular endothelial cells. Parasites co-localized only with podoplanin-positive cells (Fig. 3). However, parasite development was not complete in lymph nodes. Indeed, although at 15 h lymph node parasites had a size, shape and fluorescence intensity that were similar to those of EEF developing inside hepatocytes of the same animals, at 24 h the lymph node parasites had a smaller size than that of concomitant liver EEF, and at 52 h post-injection, fluorescence was not detected in popliteal nodes, while large fluorescent EEF were present in the liver. Finally, RT-PCR experiments were performed to confirm that parasites were partially developing inside lymph nodes. Expression of the TRAP gene, which normally peaks in sporozoites and decreases in liver EEF, was not detected at both 15 h and 24 h, while expression of the HEP17 gene, which is weak in sporozoites and sharply increases in liver EEF, was detected in lymph node EEF at both 15 and 24 h. This demonstrates the presence of developing parasites inside lymph nodes.
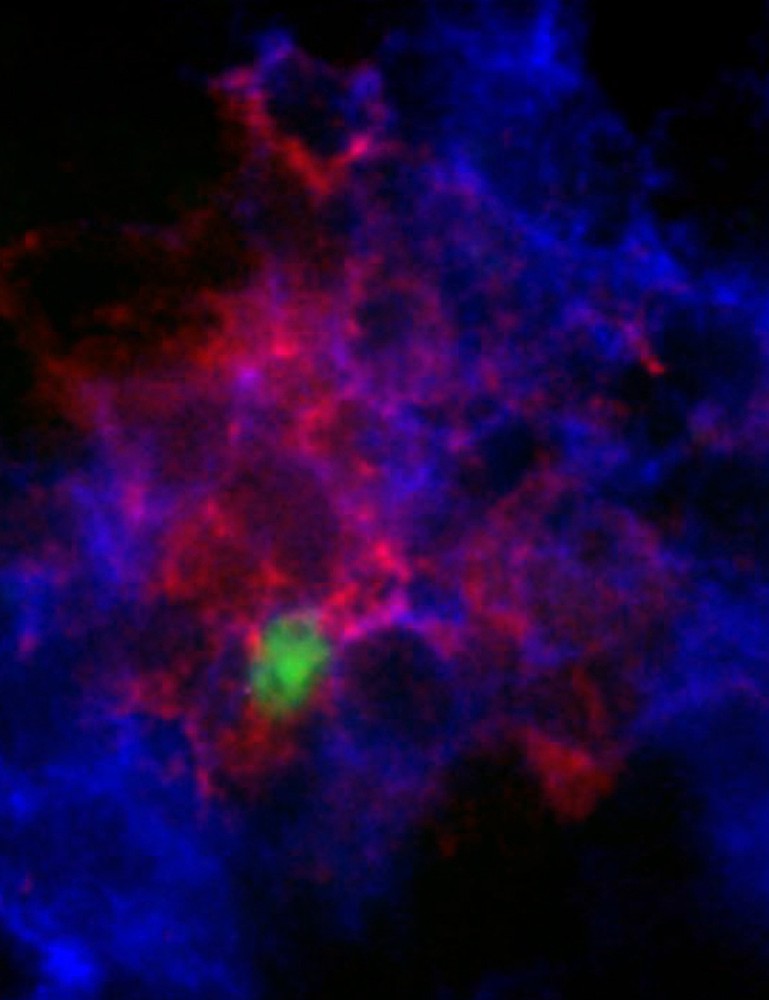
Lymph node EEF co-localize with endothelial cells. Twenty-four hours after parasite injection into the footpad of mice, popliteal lymph nodes were removed and stained with antibodies to podoplanin (red). Lymph node exo-erythrocytic forms (EEF) of the parasite (green) co-localize with labeled endothelial cells.
These observations indicate that Plasmodium sporozoites inoculated by Anopheles mosquitoes have various fates in the mammalian host. Sporozoites can either remain in the dermis, or invade blood or lymphatic vessels. Sporozoites drained by lymphatics are trapped in the proximal lymph node, where most are degraded but some can partially develop and deliver early EEF antigens. Many questions remain about the pre-erythrocytic phase of the parasite life cycle. What is the fate of the sporozoites left in the dermis? How do sporozoites leave the liver sinusoids and reach the parenchyma? How do sporozoites resist phagocytosis by macrophages, both in the dermis and in the liver sinusoids? It will also be important, in view of the renewed interest in live attenuated sporozoite vaccines, to investigate the contribution of dermal as well as lymph node parasites in mounting a protective immune response, or instead in inducing tolerance to parasite antigens. The increasingly powerful imaging tools should help in dissecting these new host–parasite interactions and the host responses they generate in a physiological context.