1 Introduction
The babirusa, Babyrousa babyrussa, is a member of the pig family (Suidae). It is endemic to the tropical forests of the Indonesian islands of Sulawesi, Buru and those of the Sula and Togian island groups [1,2]. Anatomically it is an unusual species with a number of characters not shared with its suid relatives [1,3]. In this paper we focus on one of these anatomical anomalies, the stomach.
The stomach of the babirusa differs from those of the other pig species in a number of important aspects [4–7]. These include the large size of the stomach, its large diverticulum ventriculi, and the much larger surface area of mucus-producing cardiac glands. The latter occupy more than 70% of the surface area of stomach mucosa of the adult animal compared to about 30% in the adult domestic pig [4]. An additional feature, which appears to be unique to the babirusa, is the ‘honeycomb’ arrangement of epithelial-like cells that cover this large cardiac gland area [6,7]. However, neither the cellular structure nor the origins of these epithelial-like cells were apparent, and further studies were undertaken to answer these questions.
We obtained stomach tissues from eleven babirusa that were euthanized for veterinary reasons, not related to the gastro-intestinal tract. The quality of fixation permitted transmission electron microscopic investigations. These investigations revealed details of the previously unknown microscopic structure of the cellular sheets arising from the luminal surface of the tunica mucosa of the cardiac gland region of the stomach.
2 Material and methods
2.1 Specimens
The cardiac regions of the stomachs of eleven babirusa from eight zoological collections were used for this study. Details of the animals and the fixation materials employed are contained in Table 1. The animals are identified by their international studbook number [8,9]. All of these individuals were euthanized for veterinary reasons not related to the gastrointestinal system. Samples were collected during autopsies carried out shortly after death. The digesta adhering to the stomach was very gently washed off with water and the samples were then initially fixed either in Bouin's fluid, Klotz fluid, 10% formalin or 3% glutaraldehyde. Those fixed in Bouin's fluid were kept in the fluid for 24 h only, after which they were stored in 70% alcohol.
Studbook and specimen identification, sex, place of death and preliminary tissue fixative solution for each specimen of babirusa stomach collected for scanning and transmission electron microscopy
Studbook ID | Specimen ID | Sex | Place of death | Fixative |
Scanning electron microscopy | ||||
8 | 576 | F | Stuttgart Zoo | 10% Formalin |
12 | 381/578 | M | Frankfurt Zoo | Klotz fluid |
47 | 592 | M | Antwerp Zoo | 10% Formalin |
48 | 388/575 | F | Antwerp Zoo | Bouin's fluid |
49 | 470/577 | M | Antwerp Zoo | 10% Formalin |
80 | 579/633 | M | Rotterdam Zoo | 10% Formalin |
347 | 545 | F | Surabaya Zoo | Glutaraldehyde |
355 | 558 | M | Surabaya Zoo | Glutaraldehyde |
Transmission electron microscopy | ||||
47 | 592 | M | Antwerp Zoo | 10% Formalin |
147 | 686 | F | Copenhagen Zoo | Glutaraldehyde |
183 | 700 | M | Edinburgh Zoo | Glutaraldehyde |
200 | 699 | M | Marwell Zoo | Glutaraldehyde |
347 | 545 | F | Surabaya Zoo | Glutaraldehyde |
355 | 558 | M | Surabaya Zoo | Glutaraldehyde |
For scanning electron microscopy, all specimens were subsequently placed in a solution of 3% glutaraldehyde in 0.1 M sodium cacodylate buffer (pH = 7.3) overnight and then immersed in 2% guanidine hydrochloride and 2% tannic acid for a second night [10]. They were subsequently post fixed in 2% osmium tetroxide in distilled water for 8 hours. Dehydration in graded concentrations of acetone (50%, 70%, 90%, 100%) was followed by critical point drying using carbon dioxide [11]. After they were mounted on aluminium stubs, the specimens were sputter coated with 20 nm gold/palladium [12] and viewed in a Phillips 505 scanning electron microscope.
For transmission electron microscopy, all samples were subsequently fixed in 3% glutaraldehyde in 0.1 M sodium cacodylate buffer, pH 7.3, for 2 hours. Specimens were then post-fixed in 1% osmium tetroxide in 0.1 M sodium cacodylate buffer for 45 minutes and were then washed in three, 10 minute changes of 0.1 M sodium cacodylate. The samples were then dehydrated in graded concentrations of acetone (50%, 70%, 90%, 100%), and embedded in araldite resin. Sections, 1 μm thick were cut on a Reichert OMU4 ultramicrotome, stained with toluidine blue and viewed in a light microscope to select suitable areas for investigation. Ultrathin sections, 60 nm thick, were cut from selected areas, stained in uranyl acetate and lead citrate then viewed in a Philips CM12 transmission electron microscope.
3 Results
The cardiac gland region of the stomach is characterised by a ‘honeycomb’ pattern when viewed from the lumen (Fig. 1). No differences in the general or particular aspects of this structure were found between males and females. Patterns of narrow, interconnected ridges formed approximately hexagonal polygons (Figs. 1 and 2). The circumference of each polygon lay between about 250 and 500 μm and the thickness of the ridges appeared to lie between 8 and 20 μm. These ridges represented the openings into the stomach lumen of an array of thin-walled tubes, set in close proximity to one another. Mucus coated the luminal ends of many of these tubes (Figs. 1 and 2A). Granular material filled the tubes, and this was identified as a complex mass of bacterial rods and chains of cocci (Fig. 2B). Where the walls of the tubes could be observed, these too were coated with a complex microflora (Figs. 2C and 3). In addition, these bacteria appeared to be concentrated in circles of about 8–20 μm in diameter, which were slightly raised from the walls of the thin-walled tubes (Figs. 2C and 3B). Closer inspection revealed that these circular aggregations of bacteria were gathered around raised circular disruptions in the membranous structure of the thin-walled tubes.

Scanning electron micrograph of the surface of the cardiac gland region of the babirusa stomach. Note the ‘honeycomb’ appearance of the luminal surface (H) after removal of the layer of mucus (M). Scale bar = 1 mm.
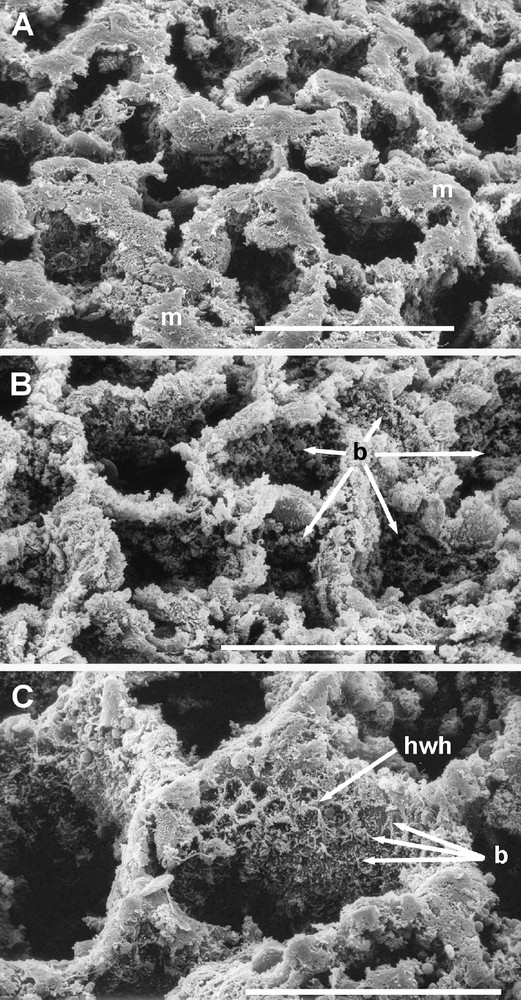
Scanning electron micrographs of the luminal surface of the ‘honeycomb’ over the cardiac gland area of the babirusa stomach. Scale bars = 100 μm. (A) The appearance of the ‘honeycomb’ after removal of some of the mucus (m), the remainder of which lay on the tops of the walls. (B) The lumen of each of the ‘honeycomb’ tubes was filled with the secretions of the glandular epithelium and inhabited by a dense population of bacterial rods and cocci (→). (C) The walls of the ‘honeycomb’ structure close to the luminal opening of the tube had a pattern which suggested a ‘honeycomb within the honeycomb’ appearance (hwh) accentuated by the clustering of bacteria (b).
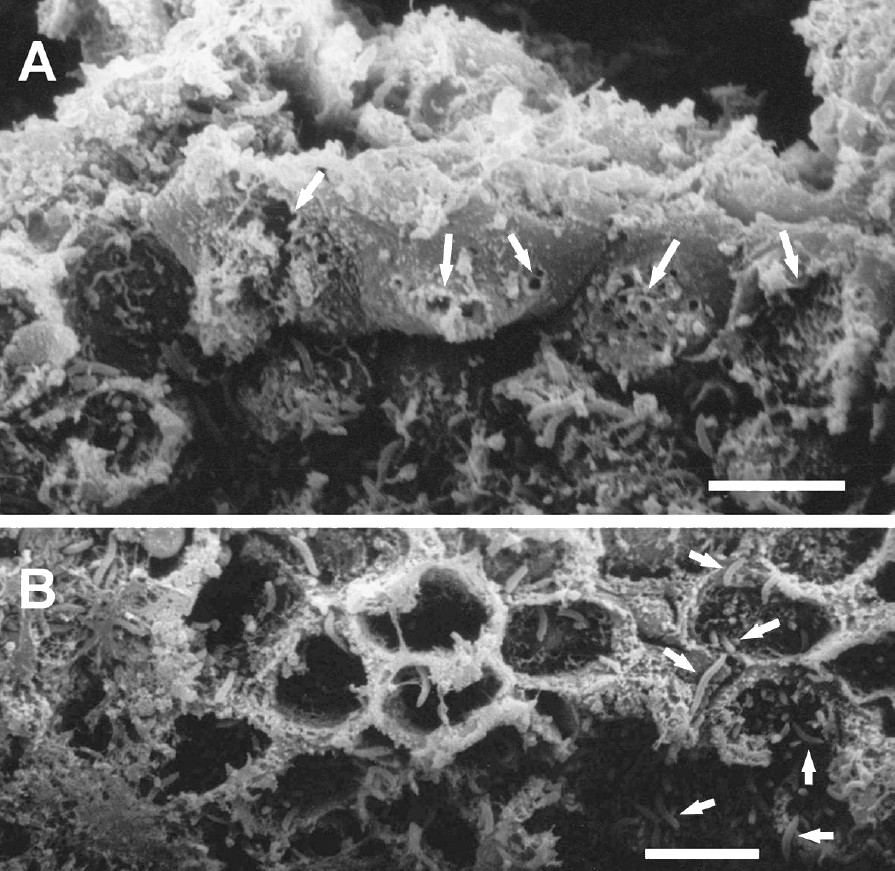
(A) Scanning electron micrograph of the luminal surface of the ‘honeycomb’ layer in the cardiac gland region of the babirusa stomach illustrating epithelial membrane disruption (→) in adjacent cells. Scale bar = 10 μm. (B) Scanning electron micrograph of the luminal wall of one of the ‘honeycomb’ tubes, illustrating the array of short goblet-like structures arising from the tube wall which suggested the ‘honeycomb within the honeycomb’ appearance. Note the presence of bacteria (→). Scale bar = 10 μm.
Longitudinal sections through the ‘honeycomb’ array of thin-walled tubes demonstrated that the tubes were between 200 and 260 μm in length (Figs. 4 and 5). The membrane disruptions mentioned above were largely concentrated in the upper, luminal half of the tubes. Towards the bases of the tubes, goblet-shaped bulbous protrusions extended from the tube walls. Some of these bulbous protrusions appeared to be punctured (Fig. 5). Bacteria were evident throughout the length of the tubes and into the gland pits. The structural arrangement of the ‘honeycomb’ pattern of thin-walled tubes in relation to the pits of the cardiac glands is illustrated in Fig. 6.
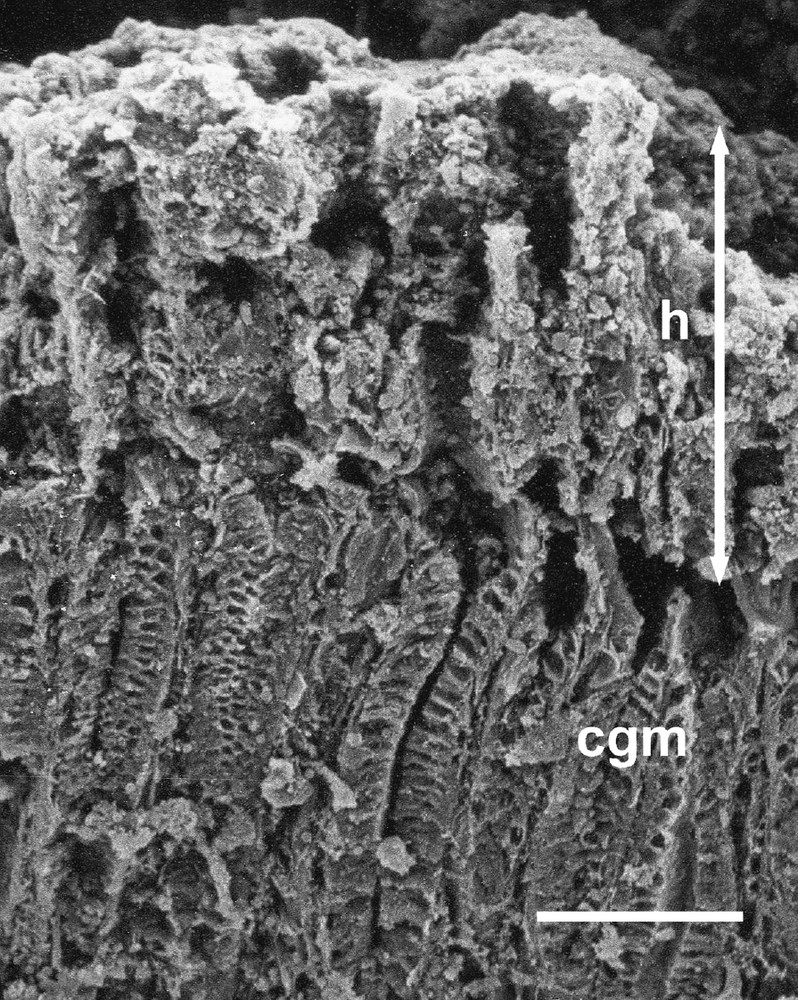
Scanning electron micrograph of a vertical section through the wall of the cardiac gland area of the babirusa stomach. cgm = cardiac gland mucosa; h = ‘honeycomb’ layer; ↕ = the height of the ‘honeycomb’ layer. Scale bar = 100 μm.
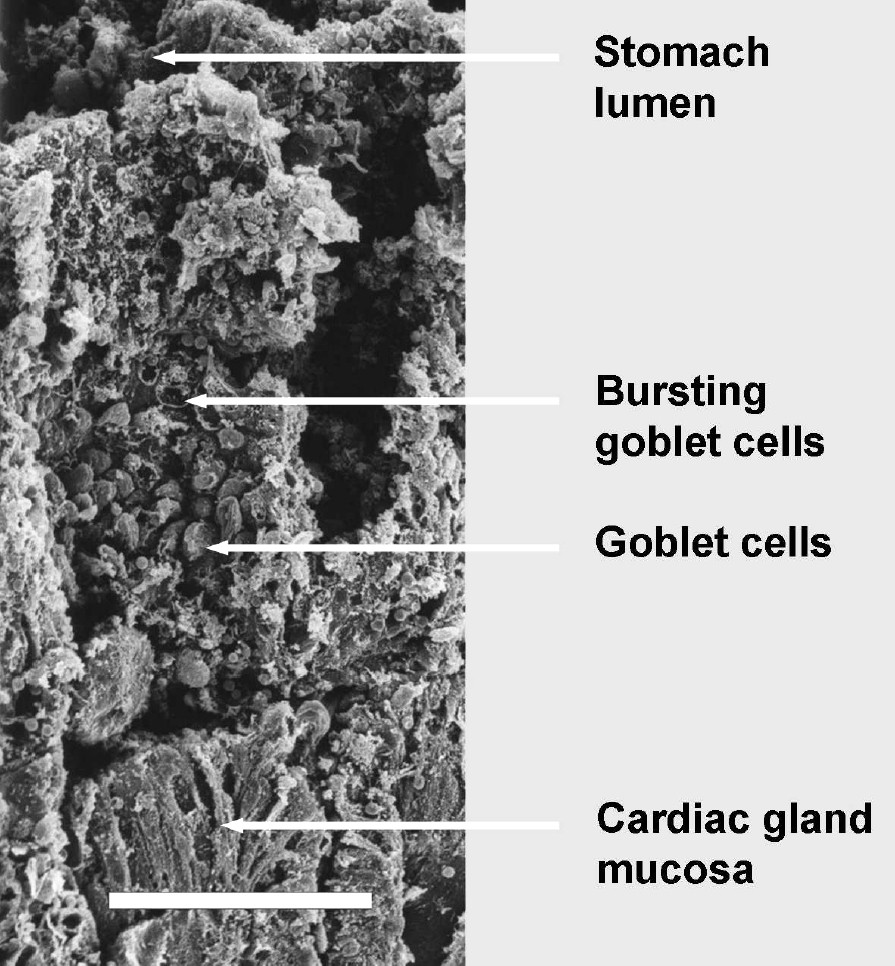
Scanning electron micrograph of a vertical section through the ‘honeycomb’ layer in the cardiac gland region of the babirusa stomach illustrating the pattern of change in the tubular surfaces: the proximal half of the tubes, close to the cardiac gland mucosa, contained protruding goblet cells; the goblet cells half way up the tube appeared to have burst, and the distal cells appeared flatter. Disrupted cell membranes were evident in that part of the thin-walled tube close to the stomach lumen. Scale bar = 100 μm.
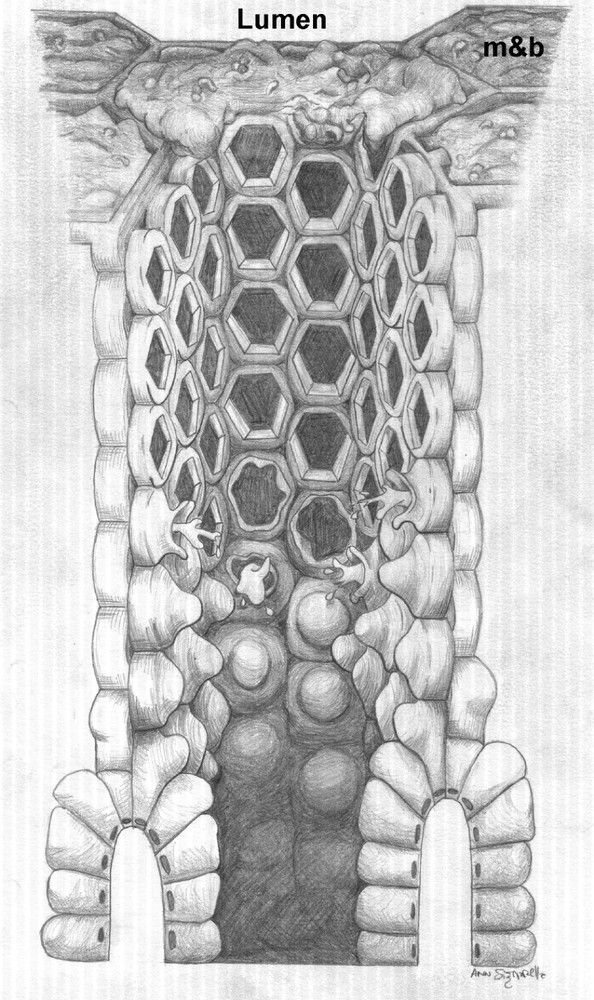
A schematic diagram of the ‘honeycomb’ arrangement of tubes covering the cardiac gland region of the babirusa stomach: mucus and bacteria (m&b) have not been included in the central tube, firstly to illustrate the arrangement of cells comprising the tube wall which give rise to the ‘honeycomb within the honeycomb’ appearance towards the lumen, and secondly to show the manner in which these cells arise from the glandular epithelium. For diagrammatic purposes the height of the tube has been somewhat shortened.
The glandular mucosa of the extensive cardiac region largely comprised columnar epithelium. This columnar epithelium was continuous from one glandular pit or fovea to the adjacent pit (Figs. 4–6). Many of the cells were mucus-secreting goblet cells (Fig. 7). Microvilli appeared to cover the apical surfaces of many of these cells. However, those cells with either distended apical surfaces, or which appeared to be discharging their mucus granules, had many fewer microvilli. Mucus secretory granules were generally round in shape and varied both in size and in electron density. Those cells with distended or open apical surfaces tended to have mucous granules of lower electron density in the region of the apical surface (Fig. 7). Bacteria were evident around and within the mucus secretion in the lumen of the glandular pit (Fig. 7).

Transmission electron micrograph through the glandular mucosa in the pit region of the cardiac gland area of the babirusa stomach. b = bacteria; g = goblet cell mucus; ▸ = junctional complex; mv = microvilli; Scale bar = 5 μm.
Transmission electron micrographs of longitudinal sections through the thin-walled tube and the ridges between the underlying glandular pits showed the relationship between these structures. Approximately midway between adjacent glandular pits, pairs of cells arose from the columnar mucosal layer to form thin, connected sheets of tissue which projected towards the lumen of the stomach (Figs. 6 and 8). The stratified cells comprising the thin-walled tube were attached to one another by means of desmosomes. Where they arose from the glandular mucosa they were attached by means of junctional complexes and desmosomes to the columnar goblet cells on the ridges between adjacent glandular pits (Fig. 8A and B). The nuclei of some of these cells of attachment appeared to be enlarged whereas others seemed relatively small and electron dense. The cytoplasm was sometimes vacuolated and the cytoplasmic granules were less electron dense than those of neighbouring mucus-filled goblet cells (Fig. 8A). Several of the cells of the tubes showed aggregations of mucus granules in their cytoplasm (Fig. 8A), and microvilli tended to be absent from the membranes of these cells. Bacteria of various sizes were present in the lumen of each thin-walled tube (Fig. 8B).

Transmission electron micrographs of sections through the junction between the cardiac gland tissue and the ‘honeycomb’ arising from it in the babirusa stomach. (A) Oblique section showing the dark-stained mucus in the goblet cells of the cardiac glandular mucosa (lower left) contrasted with the paler stained mucus present in the cells of the ‘honeycomb’ tube (upper right). (B) Longitudinal section illustrating the transition from the ridge of the cardiac glandular mucosa (lower half) to the cells of the ‘honeycomb’ tube, and the varied and dense population of bacteria around and within the secreted mucus. b = bacteria; g = Mucus aggregations in the goblet cells; L = lumen of the ‘honeycomb’ tube; m = mitochondria; mv = microvilli; n = nucleus; P = glandular pit; s = glandular secretion; ▸ = tight junctions; scale bar = 5 μm.
The thin-walled tubes were between twelve and sixteen cells in height, and were usually two layers of cells (8–20 μm) in thickness (Figs. 6 and 8). Some longitudinal sections through the length of the tube demonstrated the arrangement of cells in the plane of one of the walls of the ‘honeycomb’ tube (Fig. 9). These cells had a somewhat flattened polygonal appearance and adhered to one another by means of desmosomes and junctional complexes. Many of the cells demonstrated vacuolated cytoplasm. Others contained mucus granules. Some of the cells lining the lumen of the tube showed open goblet-like structures with bacteria coating the cell membranes and populating the interior of the open goblet-like structure (Fig. 9). These open goblet-like structures were also seen in cross sections of the ‘honeycomb’ of thin-walled tubes (Fig. 10). The largely two-cell structure of the tube walls was evident, although at the corner junctions between adjacent tubes the walls appeared to be three or more cells thick. Bacteria were evident within the ‘honeycomb’ tubes but were not seen inside the cells themselves except at the boundary of the ‘honeycomb’ and the stomach lumen. Here cell membranes were disrupted (Figs. 3a and 11) and dense populations of bacteria were present around and within the cell cytoplasm (Fig. 11).
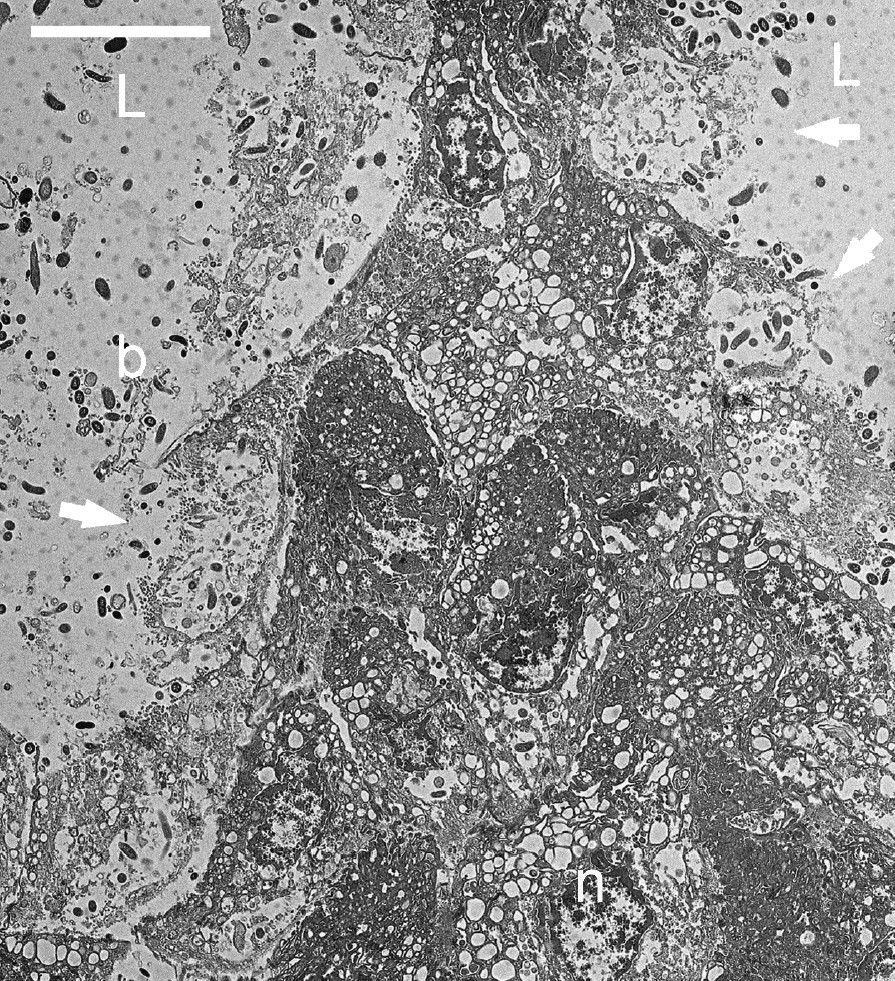
Transmission electron micrograph of an oblique longitudinal section through the upper part of the sheet of vacuolated cells forming a wall of one of the ‘honeycomb’ tubes in the babirusa stomach. The cells were attached to one another by means of desmosomes. Note the goblet-shape of the disrupted cellular membrane and the bacteria present within the cavity (→). b = bacteria; L = lumen of the ‘honeycomb’ tube; n = nucleus. Scale bar = 10 μm.
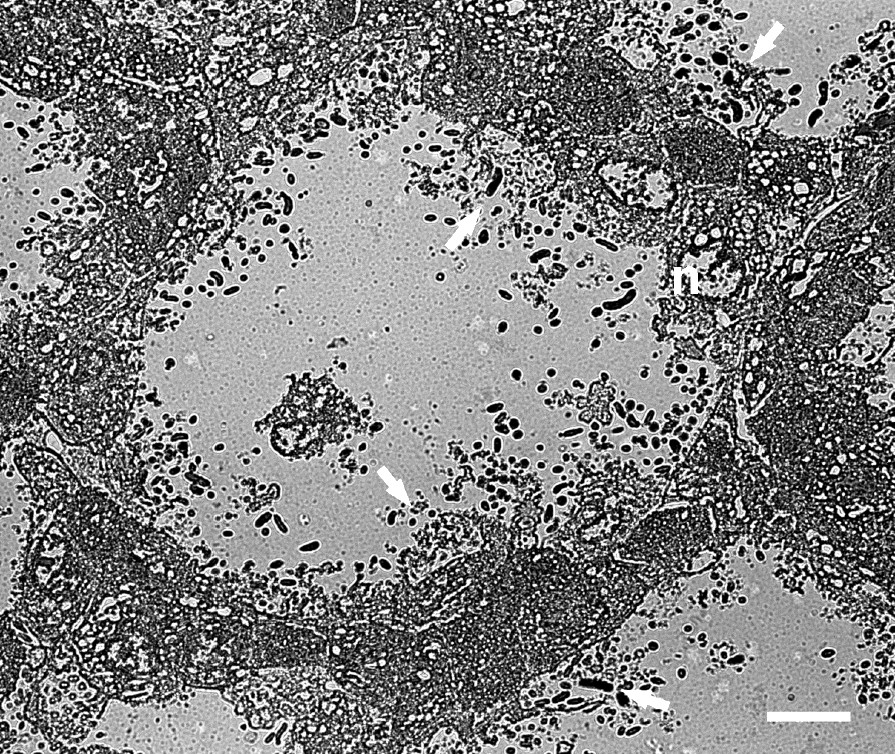
Transmission electron micrographs of a cross-section through the ‘honeycomb’ arrangement of tubes covering the cardiac gland region of the babirusa stomach illustrating the two- to three-cell thickness of the walls. → = the goblet-like shape of the disrupted cellular membranes and the bacteria present within the cavity; n = nucleus. Scale bar = 5 μm.

Transmission electron micrograph of a slightly oblique longitudinal section through the upper part of the sheet of cells forming the top part of the wall of one of the ‘honeycomb’ tubes in the babirusa stomach. b = bacteria; L = lumen of the stomach; n = nucleus. Scale bar = 10 μm.
4 Discussion
This study has enabled the first detailed description to be given of the ultrastructure of the unique tissue overlying the cardiac glands of the babirusa stomach. It has revealed that the thin, epithelial-like cells which comprised the ‘honeycomb’ overlying the cardiac glands were derived from the columnar epithelial mucosa of the cardiac glands (Fig. 6). Moreover, these relatively thin cells demonstrated an ability to retain elements of their former mucus-releasing, goblet-cell function for some distance beyond the histological structures normally recognised as representative of the cardiac gland mucosa of the stomach.
No comparable evidence of this type of ‘honeycomb’ arrangement of thin-walled tubes has previously been reported from studies of the histology and ultrastructure of the cardiac gland area in the stomach of the domestic pig [7,13–15]. Indeed, literature studies failed to find a single reference to comparable ‘honeycomb’ structures on the surface of the cardiac gland area of either wild pigs or any non-ruminant forestomach fermenters [7]. Experience has shown that this may be due to the need to collect and conserve tissue samples from the stomach very shortly after the death of the animal; autolysis of stomach tissues takes place very rapidly [16]. Within our collection of samples, cellular disruption and destruction of the ‘honeycomb’ area was obvious if the samples had not been collected and placed in the appropriate fixative within 15 minutes of the death of the animal [6], [Macdonald and Leus, unpublished]. Two particular groups of animals, the colobine monkeys and the macropod marsupials, share with the babirusa the possession of an enlarged region of the stomach lined almost exclusively with cardiac glands. Although no ‘honeycomb’ layer has been reported for these animals by others, and was not found over the cardiac gland region in the stomach of the Bennet's wallaby (Macropus rufrogriseus) by Leus et al. [7], further research is needed on the stomachs of other examples of the macropod marsupials and the colobine monkeys to test the uniqueness of the structure in the babirusa stomach.
It has long been recognised, as a result of work carried out on laboratory species that the columnar cells of the cardiac gland migrate from the pit wall to the luminal surface of the stomach [17–19]. Their fate after reaching the surface, was either to degenerate or to be extruded into the lumen of the stomach [18,20,21]. Two forms of cellular degeneration were noted on the luminal surface of the cardiac glands by Karam and Leblond [22]. In the first, the cytoplasm became pale and vacuolated and the nuclear matrix tended to disintegrate. Alternatively there was a tendency for the nuclear chromatin to condense and the cytoplasmic components to aggregate. In the babirusa, those columnar cells immediately under the ‘honeycomb’ showed some of these signs of degeneration (Figs. 8–10). However, instead of subsequently either being lost into the lumen of the stomach or being phagocytosed by neighbouring cells [22], the babirusa cardiac gland cells retained their attachment to their predecessors and neighbours and became stratified. Unlike the dense, multi-layered stacking of stratified squamous epithelium, these cells appeared to layer like plates stacked on edge, and in this configuration contributed to the formation of the cellular tubes.
The structural properties of naturally occurring hexagonal tubular arrays have been discussed in some detail by Gibson [23], and with specific respect to the exoskeletons of the Blue crab (Callinectes sapidus) [24] and the American lobster (Homarus americanus) [25], the lungs of birds [26], and the sperm-storage region in the seminal vesicles of the auchenipterid catfish (Trachelyopterus lucenai and T. galeatus) [27]. With respect to the babirusa stomach, the cardiac gland ‘honeycomb’ can be regarded as sited between two relatively stiff structures; that comprising the mass of largely vegetable ingesta within the stomach lumen, and that representing the mucosal layer of the cardiac glands. The approximately hexagonal, tubular structure of the ‘honeycomb’ array in the babirusa's stomach may have the dual effect of increasing the tissue's moment of inertia as well as providing efficiency in resisting bending and buckling loads resulting from the contraction of gastric smooth muscle [23]. If demonstrated to be so by experimental investigation, it would help explain the functional integrity of this self-renewing ‘honeycomb’ layer.
We estimated that about 20–30 cells comprise the circumference of each thin-walled hexagonal tube, and that the height of the tube was made up of about fourteen cells. Thus each tube was constructed from approximately 280–420 cells and was closely attached by means of desmosomes and junctional complexes to adjacent tubes. Experiments using rat (Rattus norvegicus) and mouse (Mus musculus) have shown that the average amount of time that a columnar cell remained at the top of the cardiac pit was about 12 hours [22,28]. If similar cellular turnover rates are applicable in the stomach of the babirusa then the cellular structure of the ‘honeycomb’ can be estimated to be replaced progressively over a six to eight day period.
The presence of cytoplasmic granules resembling mucus in the cells of the thin-walled tube as far as about half way up the ‘honeycomb’ suggested that these cells had an active secretory role to play despite being some histological distance away from the arterial blood supply (Fig. 8A). The absence of intact cellular membranes bulging into the lumen of the thin-walled tube towards the lumen of the stomach, and their apparent replacement with goblet-like raised rings of membrane around which populations of bacteria had aggregated suggested that this aspect of the secretory function of the cells may not extend to the lumen of the stomach (Figs. 9 and 10) In its stead, it would appear that the thin-walled ‘honeycomb’ tubes thereby obtained an internal, surface array of low tubes (Figs. 2C and 3B). These low tubes with a diameter of about 10 μm, the ‘honeycomb within the honeycomb’ shown schematically in Fig. 6, may function by contributing further to the structural stability of the ‘honeycomb’ by reinforcing its ad-luminal edge against the abrasion of ingesta [23]. Additional, micro-mechanical, studies are required to test this hypothesis.
Large numbers of bacteria inhabited the stream of mucus passing from the cardiac glands up into the lumen of the stomach. They were seen associated with the mucus in the cardiac pits (Fig. 7), throughout the closely interconnected array of cellular tubes that comprised the ‘honeycomb’ [6,7 and present study] and were present in the mucus covering the luminal ridges of the ‘honeycomb’ (Figs. 3 and 11). The identities of most of these micro-organisms remain unknown [6]. However, the close association they exhibited with the mucus secreted by the cardiac glands (Fig. 7), and with the cells and the cellular membranes of the honeycomb (Figs. 2, 3, and 8–10) indicated that this large area of the babirusa stomach may have been structured to form a stable and yet self-refreshing environment for the housing and multiplication of what appear to be autochthonous commensal bacteria. The role played by each species of them also remains to be studied. The micro-environment of the cardiac gland area may be consistent with specialised microbial fermentation of ingesta or may support microbial modification of glandular secretions [29,30].
The manner in which, and the extent to which the secretions from the cardiac glands of the babirusa contribute to microbial fermentation have not yet been studied. Histochemical studies indicate that neutral glycoproteins are produced deep in the babirusa's cardiac glands and that neutral and both sulphated and non-sulphated acid glycoproteins are present in the cells of the gastric pits and at the surface of the stomach [4]. Mucin-type glycoproteins are thought to be the major structural proteins in mucus. In rodents and humans, immunoreactive class-III mucins are found in the mucous cells of cardiac glands [31,32], thereby confirming biochemical analyses which had earlier suggested that the sugar residues responsible for class-III paradoxical concanavalin A histochemical reactivity were N-acetylglucosamine (GlcNAc) residues [33]. Class-III mucin is an essential component of the human gastric surface mucous gel layer and plays an important part in protecting the gastric mucosa [34]. The production of mucus is stimulated by high fibre diets in rats [35].
In addition to the mucus-secreting cells, several different types of endocrine cells have also been found in the cardiac gland region of the babirusa stomach [5]. Cells immunoreactive for chromogranin, serotonin, somatostatin, BPP, glucagon and MENK8 have been detected, with the numbers of BPP and MENK8-immunoreactive cells being greater than found in the equivalent region of the domestic pig stomach. Thus, when taken together the available observations indicate that there remains a complex of unresolved questions waiting to be investigated in the cardiac region of the babirusa stomach. Factors such as pH, bacterial adhesion, bacterial cooperation, diet composition, nutrient availability, redox potential, smooth muscle contraction, bacterial antagonism and mucin composition can be anticipated to play important roles in the functioning of the ‘honeycomb’.
Acknowledgements
The authors gratefully acknowledge the assistance of the veterinarians, veterinary staff, curators and animal keepers of the zoos which housed the babirusa used in this study. The positively critical and helpful comments made by one reviewer were much appreciated. The work was financially supported by the Development Trust of the University of Edinburgh (to AS) and the Balloch Trust. The Centre for Research and Conservation of the Royal Zoological Society of Antwerp gratefully acknowledges the structural support of the Flemish Government.