1 Introduction
Most burrowing petrels and shearwaters (Procellariiformes) are only nocturnally active at breeding sites. This is generally considered an adaptative response to predation risk, limiting encounters with diurnal predators (e.g., [1,2]), or to food availability, since foraging patterns depend on vertical prey migration [3–6]. Even if presence and activity patterns in petrels and shearwaters have been described for several species (e.g., [7–9]), analyses of specific factors affecting these patterns have been much more sporadic, and no detailed study has yet been performed on this topic. Furthermore, environmental and biological factors may influence these patterns. In particular, some authors have indicated that breeding cycle stage, the time of sunset and moonlight intensity were the most influential parameters concerning petrel and shearwater presence at colonies [1,2,10–13]. The influence of these factors seems to be quite different depending on the bird breeding status. Sensitivity to moonlight seems stronger in non-breeders than in breeders, because they do not have as many nest duties as breeders and they are more vulnerable to predation once they are grounded, staying on open ground without burrow protection [13–16]. Presence and activity patterns seem to differ between these two breeding status in breeding cycle stage of presence, arrival time, and behaviour. Pattern differences between males and females have also been shown, with stronger male presence and ground activity associated with the pre-laying period in order to defend a burrow and attract females [11,14–16].
Here, we describe and test the influence of several environmental and biological factors on presence and activity patterns of yelkouan shearwaters Puffinus yelkouan at their colonies. The yelkouan shearwater is endemic to the Mediterranean Basin and is not considered threatened according to the IUCN criteria, although (1) some populations suffer mortality from introduced predators and (2) so far, no study on the population dynamics of this species has been conducted [17]. To understand and/or to better assess the impact of eventual predators on land and the factors of susceptibility to predation, it is necessary to study the behaviour of the yelkouan shearwater at its breeding colonies. Although understanding presence and activity patterns at breeding sites is a prerequisite for studying a species, we found only general and rough descriptions for the yelkouan shearwater [18]. In order to offset this gap, (1) we describe the presence and activity patterns of yelkouan shearwaters, identifying arrival times at colonies and activity levels in relation to breeding cycle stage and the time of night. Breeding site arrival times for burrowing Procellariiformes are generally given in reference to sunset (e.g., [12,19]). Because the deep and winding burrows of these birds prevent accessibility [20], capture studies are restricted to arrival times. A better estimation and understanding of this timing will therefore facilitate yelkouan shearwater banding and monitoring. Furthermore, Zotier and Vidal [21] mentioned that European storm petrels Hydrobates pelagicus were rare or little active at their breeding sites when atmospheric pressure was very low. Strong wind is also likely to affect shearwater activity levels, reducing communication or landing success. Therefore, (2) we not only test the effect of moonlight intensity (time of the start of obscurity, lunar phase and cloud cover), but also that of atmospheric pressure and wind speed on the presence and activity patterns of yelkouan shearwaters. Finally, (3) we also wish to relate colony attendance patterns to bird status (sex and breeding/non-breeding status).
2 Materials and methods
2.1 Study area and species
This study was conducted on the Port-Cros (43°00′N, 6°23′E) and Porquerolles (43°00′N, 6°12′E) islands, in the Hyères archipelago, off the southeastern coast of France. These two proximal Mediterranean islands house 210–270 breeding pairs of yelkouan shearwater. The first returns to breeding sites occur in late October or early November ([18], our own data). Egg-laying takes place from mid-March to early April, hatching in May and fledging in July and early August. Both adults incubate the single egg (shifts of four days on average) and rear the chick [18]. Approximately five days after hatching, the chick is left alone in the burrow during the day. Black rats Rattus rattus and feral cats Felis catus were introduced for several centuries on study islands and proven as predators [22–24]. The yellow-legged gull Larus michahellis has colonised the entire coast of the study islands, but only one potential case of predation on yelkouan shearwaters was observed [18]. Finally, few pairs of the peregrine falcon Falco peregrinus breed on study islands, while this raptor has been shown to prey upon the yelkouan shearwater in Greece [25].
2.2 Arrival times
In order to identify a parameter accurately predicting the arrival time of yelkouan shearwaters, we considered five standards for describing the onset of dusk: (1) sunset (time at which the trailing edge of the sun sinks below the horizon), (2) civil dusk (time at which the sun is 6 degrees below the horizon in the evening: objects are distinguishable), (3) nautical dusk (time at which the sun is 12 degrees below the horizon in the evening: objects are no longer distinguishable and the horizon is no longer visible to the naked eye), (4) astronomical dusk (time at which the sun is 18 degrees below the horizon in the evening: the sun no longer illuminates the sky) and (5) moon setting (time at which the trailing edge of the moon sinks below the horizon). These parameters were calculated by entering the coordinates of the study islands in the IMCCE calculator (‘Institut de mécanique céleste et de calcul des éphémérides’, http://lychnis.imcce.fr/cgi-bin/levcou.cgi). The time of yelkouan shearwater arrival (i.e., first contact) at three colonies on the Port-Cros and Porquerolles islands was noted for 44 nights during the 2005, 2006, and 2007 breeding seasons. Since light intensity of a waxing half moon is equivalent to a waning half moon and a total lunar cycle is 29.5 days long on average, we defined the lunar phase as the age of the moon from the new moon (lunar phase = 0) to full moon (lunar phase = 14.75). The cloud cover was characterised by nine classes describing the proportion sky with clouds and ranging from cloudless sky (cloud cover = 0) to totally overcast sky (cloud cover = 8). We distinguished three categories of nightfalls according to light intensity: moonless (lunar phase = 0 or set moon), slightly moonlit (moon obscured by cloud cover ⩾ 4 and/or lunar phase ⩽ 4) and moonlit (lunar phase > 4 and cloud cover < 4) nightfalls.
We determined which dusk standards were the best concordant with yelkouan shearwater arrival time by comparing the timing of each parameter with that of bird arrival times (ANOVA). We considered that the best concordant was the one for which the duration between the predictor and shearwater arrivals was the shortest, and the relationship between arrival times and the predictive parameters (linear regressions r) was the strongest. We tested the effect of date (considering 1 November, i.e., the beginning of the pre-laying stage, as day one), nightfall light intensity (moonless = 1, slightly moonlit = 2, moonlit = 3), atmospheric pressure and wind speed on the length of time between the best parameter and bird arrival time using a multiple-regression model. All 15 possible combinations of the four variables were included as competing models. We ranked models by using Akaike's Information Criterion [26,27] for limited sample sizes (AICc) and calculated differences between the model AICc and the lowest AICc (ΔAICc) and AICc weights (wAICc). We selected the best model as that with and 95% confidence intervals (CI) of coefficients not overlapping zero [26]. We tested model significance and goodness-of-fit using log-likelihood ratio (LR) and Pearson tests. The regional weather report, which indicates forecasts from the signal station of Levant Island (43°02′N, 6°29′E, Hyères archipelago), was consulted for atmospheric pressure (in hPa) and wind speed (in m s−1).
2.3 Activity patterns
Bird activity intensity was evaluated by the mean number of contacts (calls, landings, silent flights) with shearwaters per minute of observation, when observation lasted more than 30 min into the first two hours after the start of obscurity (mean observation duration: 70.6 min, during the 2005, 2006, and 2007 breeding seasons). Landings can be detected through the specific sounds made by wing beats when birds slow down and by the impact of birds on the ground or in vegetation. Silent flights over the colony can be detected by silhouettes in the sky. We tested the effect of date, nightfall light intensity, atmospheric pressure and wind speed on bird presence and activity intensity at colonies using generalized linear models (binomial distribution, logit link function and gamma distribution, log link function, respectively). All 15 possible combinations of the four variables were included as competing models. We ranked models and selected the best models by using the same methods as for multiple regression models. To avoid colony size effects, this analysis was carried out on only one colony on Port-Cros Island.
To further detail yelkouan shearwater activity patterns throughout the night, we distinguished five types of contacts: silent flights, calls from the ground, calls in flight, landings and take-offs. They were counted per 30-min intervals without interruption for 11 nights in 2004 and 2006 (three during the pre-laying period and eight during incubation). All counts were made in calm weather and low lunar phase (⩽ 6), from sunset to sunrise. Bird movements were monitored with nocturnal vision (infrared) binoculars.
2.4 Sex ratio variation during the breeding season
Adult yelkouan shearwaters were captured at burrow entrance during the 2004, 2005, 2006, and 2007 breeding seasons at the three studied colonies on Port-Cros and Porquerolles islands. We determined the sex of 88 birds by molecular analysis and of 26 birds by acoustic, morphological and behavioural data [28]. We sampled during the (1) pre-laying (days just before egg-laying were avoided), (2) incubation, (3) early rearing and (4) late rearing breeding cycle stages. Sex ratios were calculated for each stage and compared to 1:1 ratios using binomial tests.
2.5 Breeder and non-breeder behaviour
The ground behaviour of birds outside burrows was monitored using nocturnal vision binoculars. By combining data from other studies on shearwaters and petrels, we described behaviour differences between breeders and non-breeders (Table 1). These differences and our knowledge of colonies (burrow location and occupancy monitored during three breeding seasons) permitted us to distinguish two categories of birds and to time how long each category stayed on open ground before entering or not burrows (comparison using a Mann–Whitney test). Observations were performed during the eight nights of activity pattern monitoring, during the incubation period.
Shearwater and petrel breeder and non-breeder behavioural differences
Breeders | Non-breeders | References |
Call from within burrow | Call from the air | [10,15,29,30] |
Coo and call simultaneously with their mate | Isolated individuals call outside burrows, on the ground | [14,16,29,31] |
Enter one burrow and stay within | Enter and leave several burrows | [15,30,32] |
Leave the burrow and come back in rapidly | Wander outside burrows | [30–33] |
Land directly close to the burrow entrance | Land and fly away rapidly, far from burrows | [30–32] |
Statistical analyses were performed using Statistica 6.0. All tests are two-tailed and means ±95% CI are given.
3 Results
3.1 Yelkouan shearwater arrival times at colonies
For moonless and slightly moonlit nightfalls, the shortest time intervals between the considered dusk standards and shearwater arrivals occurred for nautical dusk ( and , respectively, Fig. 1). In these cases, yelkouan shearwaters arrived significantly later at colonies when nautical dusk was also later (, , and , , , respectively). We considered that nautical dusk was the best concordant with yelkouan shearwater arrivals at colonies for these nightfall light intensities. Nautical-dusk–shearwater-arrival intervals were longer for slightly moonlit versus moonless nightfalls (, , ). For moonlit nightfalls, the shortest time interval between the considered dusk standards and shearwater arrivals occurred for moonset (), and yelkouan shearwaters arrived significantly later at colonies when the moon also set later (, , ). We considered that moonset was the best concordant with yelkouan shearwater arrivals for moonlit nightfalls. Among the 15 competitive models used to predict the duration between the nautical dusk/moonset and shearwater arrivals (Table 2), only four exhibited a and only model LT2 had the 95% CI of coefficients not overlapping zero. This selected model included only the date as variable and showed a significant positive relationship between the date and the duration between the nautical dusk/moonset and shearwater arrivals (, , 95% , Wald , ). This model fitted well with data (scaled Pearson , , ).
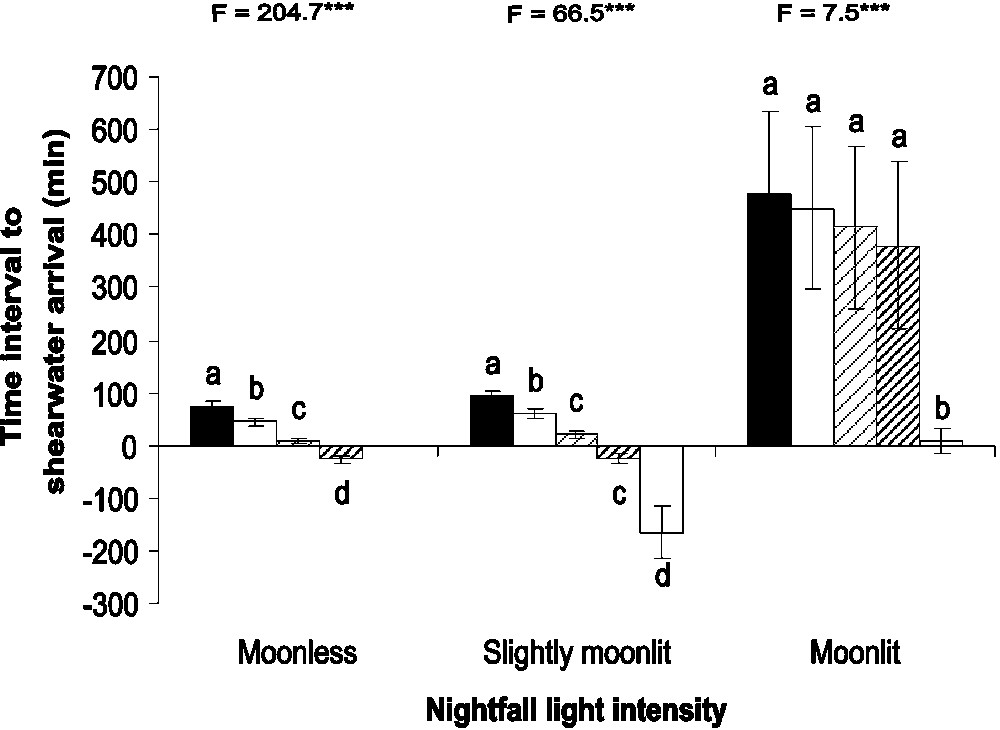
Time intervals between yelkouan shearwater arrival and five dusk standards: sunset (black), civil dusk (white), nautical dusk (thin striping), astronomical dusk (bold striping) and moonset (grey) for moonless, slightly moonlit and moonlit nightfalls (mean±95 % CI). For each nightfall light intensity, different letters indicate a significant difference in time intervals.
Statistics of the 15 candidate models used to predict the length of time between nautical dusk/moonset and yelkouan shearwater arrivals at colonies
Models | df | AICc | ΔAICc | wAICc | LR | P | |
LT1 | D + WS | 2 | 247.6 | 0.0 | 0.179 | 5.95 | 0.052 |
LT2 | D | 1 | 247.6 | 0.0 | 0.178 | 3.64 | 0.056 |
LT3 | D + AP | 2 | 248.1 | 0.5 | 0.142 | 5.49 | 0.064 |
LT4 | D + AP + WS | 3 | 248.1 | 0.5 | 0.142 | 7.96 | * |
LT5 | NLI + D | 2 | 249.9 | 2.3 | 0.058 | 3.70 | 0.158 |
LT6 | NLI + D + WS | 3 | 250.0 | 2.4 | 0.054 | 6.01 | 0.111 |
LT7 | NLI + D + AP | 3 | 250.2 | 2.6 | 0.050 | 5.87 | 0.118 |
LT8 | WS | 1 | 250.3 | 2.7 | 0.047 | 0.98 | 0.321 |
LT9 | NLI + D + AP + WS | 4 | 250.3 | 2.7 | 0.046 | 8.40 | 0.078 |
LT10 | AP | 1 | 251.2 | 3.6 | 0.030 | 0.04 | 0.841 |
LT11 | NLI | 1 | 251.2 | 3.6 | 0.029 | 0.03 | 0.861 |
LT12 | NLI + WS | 2 | 252.6 | 4.9 | 0.015 | 1.02 | 0.601 |
LT13 | AP + WS | 2 | 252.6 | 5.0 | 0.015 | 1.00 | 0.608 |
LT14 | NLI + AP | 2 | 253.5 | 5.9 | 0.010 | 0.09 | 0.956 |
LT15 | NLI + AP + WS | 3 | 255.0 | 7.4 | 0.004 | 1.04 | 0.792 |
3.2 Yelkouan shearwater activity patterns
Among the 15 competitive models used to predict shearwater presence at colonies (Table 3), only three exhibited a and only model P1 had the 95% CI of coefficients not overlapping zero. This selected model included date and wind speed as variables and showed a significant positive effect of date (, , 95% , Wald , ), and a significant negative effect of wind speed (, 95% to −0.092, Wald , ). Wind speed ranged between 2 and 37 m s−1 during the 44 nights monitored and with wind data available (). Pearson test did not find evidence of model inadequacy (scaled Pearson , , ). We did not observe shearwaters at colonies during the four moonlit nights monitored.
Statistics of the 15 candidate models used to predict yelkouan shearwater presence at colonies
Models | df | AICc | ΔAICc | wAICc | LR | P | |
P1 | D + WS | 2 | 20.5 | 0.0 | 0.393 | 24.08 | *** |
P2 | D + NLI + WS | 3 | 21.2 | 0.7 | 0.282 | 25.90 | *** |
P3 | D + AP + WS | 3 | 22.1 | 1.6 | 0.173 | 24.92 | *** |
P4 | D + NLI + AP + WS | 4 | 23.1 | 2.6 | 0.106 | 26.62 | *** |
P5 | NLI + WS | 2 | 26.7 | 6.2 | 0.018 | 17.91 | *** |
P6 | WS | 1 | 27.5 | 7.0 | 0.012 | 14.78 | *** |
P7 | NLI + AP + WS | 3 | 28.0 | 7.5 | 0.009 | 19.05 | *** |
P8 | AP + WS | 2 | 29.1 | 8.6 | 0.005 | 15.50 | *** |
P9 | D + AP | 2 | 35.3 | 14.8 | 0.000 | 9.33 | ** |
P10 | D + NLI + AP | 3 | 36.4 | 15.9 | 0.000 | 10.67 | * |
P11 | NLI | 1 | 36.9 | 16.4 | 0.000 | 5.37 | * |
P12 | NLI + AP | 2 | 37.4 | 16.9 | 0.000 | 7.15 | * |
P13 | D + NLI | 2 | 37.7 | 17.2 | 0.000 | 6.84 | * |
P14 | AP | 1 | 38.5 | 18.0 | 0.000 | 3.79 | 0.052 |
P15 | D | 1 | 39.8 | 19.3 | 0.000 | 2.44 | 0.118 |
Activity intensity varied between 0.01 and 6.27 (, contacts min−1). We observed three peaks of activity: in December (pre-laying), February (mating) and late April–early May (hatching). Among the 15 competitive models used to predict shearwater activity intensity at colonies (Table 4), only four exhibited a and only model AI1 had the 95% CI of coefficients not overlapping zero. This selected model included only nightfall light intensity as variable and showed a significant positive effect of slightly moonlit nightfall on shearwater activity intensity (, , 95% , Wald , ). This model fitted well with data (scaled Pearson , , ).
Statistics of the 15 candidate models used to predict yelkouan shearwater activity intensity at colonies
Models | df | AICc | ΔAICc | wAICc | LR | P | |
AI1 | NLI | 1 | 12.1 | 0.0 | 0.255 | 5.38 | * |
AI2 | D + NLI | 2 | 13.1 | 1.0 | 0.153 | 6.67 | * |
AI3 | NLI + WS | 2 | 13.3 | 1.2 | 0.139 | 6.47 | * |
AI4 | NLI + AP | 2 | 14.1 | 2.0 | 0.094 | 5.70 | 0.058 |
AI5 | D + NLI + WS | 3 | 14.9 | 2.7 | 0.065 | 7.43 | 0.059 |
AI6 | WS | 1 | 15.5 | 3.4 | 0.047 | 2.01 | 0.156 |
AI7 | D | 1 | 15.6 | 3.5 | 0.045 | 1.92 | 0.166 |
AI8 | D + NLI + AP | 3 | 15.6 | 3.5 | 0.044 | 6.67 | 0.083 |
AI9 | NLI + AP + WS | 3 | 15.8 | 3.6 | 0.041 | 6.53 | 0.089 |
AI10 | AP | 1 | 16.4 | 4.3 | 0.030 | 1.10 | 0.295 |
AI11 | D + WS | 2 | 16.6 | 4.5 | 0.027 | 3.21 | 0.201 |
AI12 | AP + WS | 2 | 17.3 | 5.2 | 0.019 | 2.49 | 0.287 |
AI13 | D + NLI + AP + WS | 4 | 17.5 | 5.4 | 0.018 | 7.49 | 0.112 |
AI14 | D + AP | 2 | 17.8 | 5.6 | 0.015 | 2.04 | 0.361 |
AI15 | D + AP + WS | 3 | 19.1 | 6.9 | 0.008 | 3.24 | 0.356 |
Overall activity patterns throughout the night showed two peaks of intensity, which occurred during the three hours following nautical dusk and during the two hours preceding nautical dawn (Fig. 2). The first peak was less intense than the second one ( contacts/30 min vs. contacts/30 min, , , ). The two peaks were more distinct during the pre-laying period than during incubation. During the pre-laying period, the peaks were separated by 5.5 h of low overall activity ( contacts/30 min), while they were separated by 4.5 h of medium-intense overall activity ( contacts/30 min) during incubation (, , ). The mean intensity of overall activity was the same during pre-laying ( contacts/30 min), and incubation ( contacts/30 min, , , ), but the rate of calls from the ground was significantly higher during incubation ( calls/30 min vs. calls/30 min, , , ). Overall activity lasted 10.5 h during the pre-laying and 8.5 h during incubation.
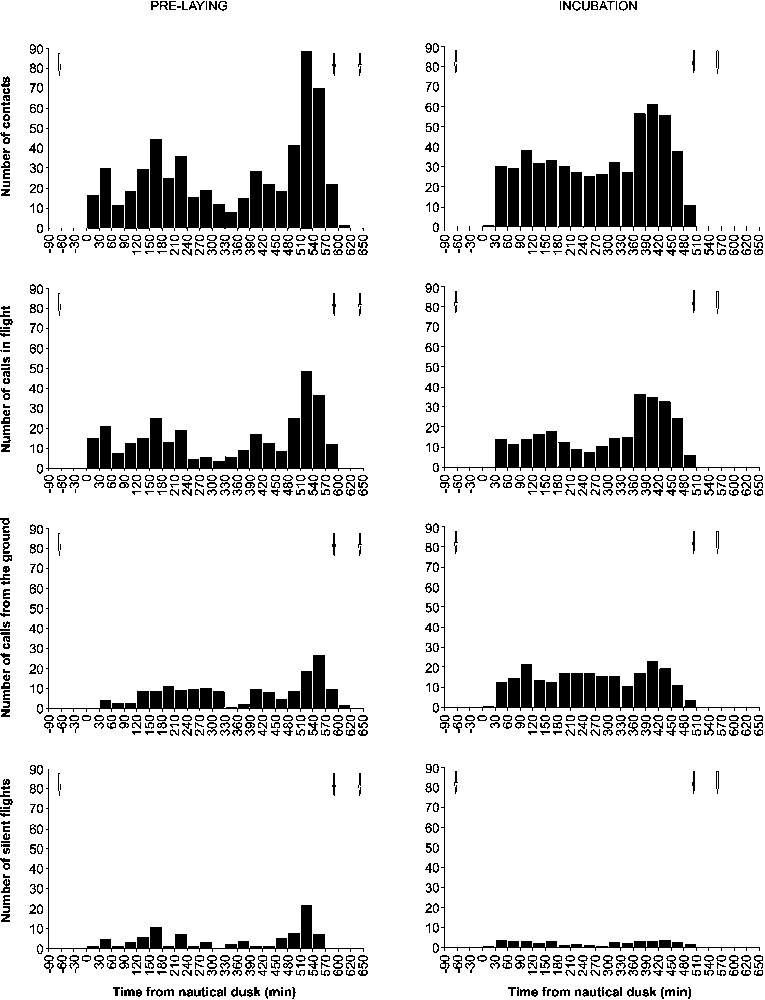
Yelkouan shearwater nocturnal activity patterns during the pre-laying period (n=3 nights) and incubation (n=8 nights). Mean numbers of all contacts, calls in flight, calls from the ground and silent flights per 30 min are indicated. White arrows indicate sunset and sunrise. Black arrow indicates nautical dawn.
Bird landing and take-off patterns throughout complete nights were different between the pre-laying and incubation periods (Fig. 3). During the pre-laying period, a peak in landings occurred into the three hours following nautical dusk (Fig. 3a). Following this period, landings and take-offs alternated and were less numerous. During incubation, one activity peak with 2.3 times as many landings as take-offs occurred during the three hours following nautical dusk ( landings/30 min vs. take-offs/30 min, , , Kruskal–Wallis , ), while a second activity peak with 3.3 times as many take-offs as landings occurred during the two hours preceding nautical dawn ( landings/30 min vs. take-offs/30 min, , , , ; Fig. 3b). Between the two peaks, almost no movement occurred. Mean movement rates were not significantly different between the two stages ( movements/30 min vs. movements/30 min, , , , ).
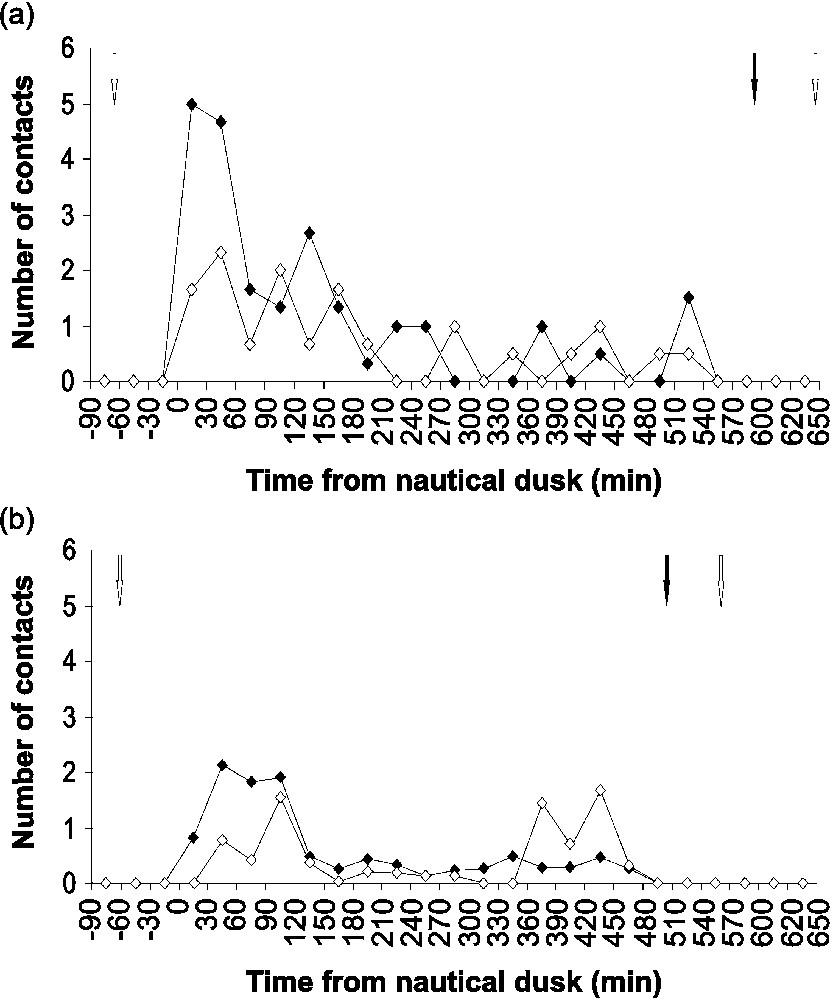
Yelkouan shearwater nocturnal movement (landings in black and take-offs in white) patterns. (a) Pre-laying period, n=3 nights. (b) Incubation, n=8 nights. White arrows indicate sunset and sunrise. Black arrow indicates nautical dawn.
3.3 Status of yelkouan shearwaters present at colonies
Sex ratios at colonies were significantly different from the 1:1 value only during the pre-laying stage, with two males for one female (Fig. 4). Observed differences in bird behaviour on the ground, combined with our precise knowledge of burrow occupancy at the studied colonies allowed us to securely distinguish 39 breeders and 49 non-breeders among birds whose activity times were recorded. Non-breeders stayed on the ground outside burrow for significantly longer times than breeders ( min vs. , , ). The longest time intervals spent on the ground outside burrow were 22 min for breeders and 90 min for non-breeders.
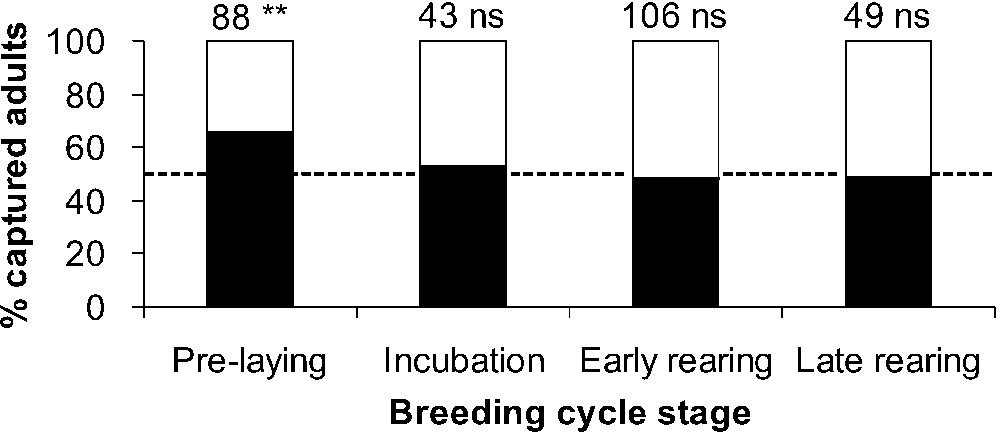
Sex ratios of yelkouan shearwater adults captured throughout the breeding season (males in black and females in white). The dotted line represents the 1:1 value. Sample size and significance of binomial tests are given for each stage.
4 Discussion
4.1 Factors affecting arrival times
We found that yelkouan shearwater arrivals were later for slightly moonlit than for moonless nightfalls and were delayed until moonset for moonlit nightfalls. This indicates that yelkouan shearwaters wait for a low light level before returning to colonies. The hypothesis that shearwaters and petrels move from the sea to colonies at a given threshold light level has also been reported by other studies [2,9,12,13], conforming with both predator avoidance and food availability theories for moonlight avoidance. Intervals between nautical dusk/moonset and shearwater arrival increased throughout the breeding period. This may be linked to variation in duties during the breeding cycle. At the beginning of the cycle, birds return to colonies to prepare and defend their burrows and re-establish pair bonds with the previous year's mate or seek a new mate [32,34]. The earlier they arrive in their burrow during the night, the lower the risks of burrow eviction and of losing their mate. Later in the cycle, both mates alternate incubation and chick-rearing duties, with the burrow always occupied by at least one mate or the chick. Early return is therefore less important. Moreover, later arrival during chick-rearing may be linked to a longer time spent in foraging in order to provide the chick with a sufficient meal size [5].
4.2 Factors affecting activity
We demonstrated a positive effect of the date in the breeding cycle and a negative effect of the wind speed on the yelkouan shearwater presence at colonies. The increase in presence frequency throughout the breeding cycle may be due to the more regular burrow attendance of both mates who alternate in egg incubation and chick provisioning. On the other hand, shearwater presence at colonies during the pre-laying period may be more sporadic and related to environmental factors because of the absence of strict breeding duties. Strong wind is likely to increase the difficulty of landing and to limit the presence at colonies because the foraging becomes more energetically interesting than the travel to colonies (reduced flight cost, [35–37]). However, wind is expected to be a favourable element for petrels and shearwaters at colonies, reducing take-off and landing costs [38]. Thus, we can expect a complex effect of wind speed on shearwater activity with a first wind speed threshold needed for take-offs and landings and enhancing presence at colonies, and a second threshold enhancing flight activity and presence at sea. It is notable that no shearwater presence was observed during moonlit nights, conforming with moonlight avoidance behaviour generally observed in petrels and shearwaters [1,2,10,13]. Activity intensity was determined only during moonless night intervals. Thus, the positive relationship between activity intensity and nightfall light intensity was not contradictory with moonlight avoidance behaviour, or with our results concerning yelkouan shearwater arrival times. This may illustrate a concentrated activity during shortened moonless periods.
4.3 Nocturnal activity variation
Yelkouan shearwaters were more active at the beginning of the night, shortly after arrival, and at the end of the night, shortly before departure to sea. Overall activity decreased markedly between these two peaks. This pattern was mainly linked to flying activity. During incubation, the decrease in overall activity was less marked due to calls from the ground emitted by mates in burrows and due to the shorter duration of the night. The two-peaked pattern of activity has been observed for other petrels and shearwaters [11,19]. While movement patterns were more or less homogenously distributed throughout the night during the pre-laying period, two peaks were marked during incubation, illustrating the change over in incubation duties between mates. In the yelkouan shearwater, this change off can be carried out quickly at the beginning of the night as soon as a mate arrives, or can last almost all night long with the departing mate staying several hours in the burrow with the arriving mate before leaving ([8], our own data).
4.4 Status of yelkouan shearwaters present on colonies
We captured more males than females during the pre-laying period, but the proportions of males and females captured during incubation and chick-rearing were similar. In most petrels and shearwaters, males arrive first at breeding sites after the interbreeding period and, during the pre-laying period, attend the nest more frequently than females who undertake a pre-laying exodus [11,30,32,34]. After egg-laying period, both males and females participate in egg and chick care. Our results suggest that the same patterns occur in the yelkouan shearwater. Yelkouan shearwater non-breeders spent four times as long time on the ground outside burrows as breeders, where they can stay for up to 90 min. James [15] observed that Manx shearwater non-breeders spent 59.3–64.3% of their time on the ground, 4.9–11.8% flying and 28.9–30.8% in burrows, confirming the long time spent by non-breeder shearwaters on the ground, without protection.
5 Conclusion
Yelkouan shearwater activity patterns at breeding sites are described for the first time in this study. While the expected influence of moonlight has been shown, we highlight that other factors are also important in these patterns, particularly breeding cycle stage and wind speed. We demonstrated that bird behaviour and presence at colonies differed between breeders and non-breeders, and between sexes, with non-breeders spending more time on the ground outside burrow and males being more present at colonies during the pre-laying period. The yelkouan shearwater is the main avian prey of feral cats on the studied islands and the peak of predation occurs from October to March [23,24]. Thus, yelkouan shearwater non-breeders and males appear to be more susceptible to predation, because they spend more time on the ground without protection and they are more present during the peak of predation by feral cats, respectively. The relation between predation risk and bird behaviour would be particularly interesting to analyse in order to better understand and evaluate the impact of predation on yelkouan shearwater population dynamics and survival.
Acknowledgements
Funds and support were provided by the EU and the DIREN PACA through the granting of a Life Nature project (ref. LIFE03NAT/F000105), the ‘Conseil régional PACA’ (contracts Nos. 2002-01625 and 2003-15028) and the Port-Cros National Park (contract No. 03 011 83400PC). We are very grateful to the director and managers of the Port-Cros National Park for granting permission and providing support to conduct this research, and to all those who helped us during field work, and especially M. Bourgeois, C. Curé, E. Guillem, C. Heurtebise, A. Vaniscotte, and S. Voiriot. We thank C.M. Suehs for improving the English. Licences and permissions for capturing and handling were given by the prefecture of the Var (authorisation No. 7/2004) and CRBPO (National Museum of Natural History, Paris).