1 Introduction
Sharks are divided into eight orders, and the largest and the most important order is the Carcharhiniformes. Carcharhiniform sharks include about 200 species, which is about 55% of all shark species [1]. This order is divided into 8 families: family Sphyrnidae: Hammerhead sharks, family Trikidae: hound sharks, family Leptochariidae: barbelled hound sharks, family Hemigaleidae: weasel sharks, family Scyliorhinidae: cat sharks, family Proscylliidae: finback cat sharks, family Pseudotriakidae: false cat sharks, and family Carcharhinidae: requiem sharks, which constitute the largest family in this order, including about 50 species, distributed into 12 genera. They are Galeocerdo, Glyphis, Isogomphodon, Lamiopsis, Loxodon, Negaprion, Nasolamia, Prionace, Rhizoprionodon, Scoliodon, Triaenodon, and the largest group is the genus Carcharhinus, with about 30 species.
This family includes many different species found in all warm and temperate seas. They are dominant in tropical waters. This is an economically very important family. Most of the species are used for food, oil, leather, fish meal, etc. Just a few of them are dangers to man. Usually, small species are found much closer to the shore than the bigger ones [2,3].
A lot of morphological and non-morphological analyses were carried out to determine the relationship among the genera. For example, the allozyme electrophoretic analyses by Naylor [4] shows that Carcharhinidae and Carcharhinus are paraphyletic, including the blue shark Prionace glauca, while parasite–host data suggest a close relationship between Carcharhinidae and Sphyrnidae by Caira [1,5,6]. Thirty-seven species of carcharhiniform sharks were used in a study about protein variation among these species by Naylor [1]. Evolutionary trees were drawn by using these data together with cladistic character and Wagner distance analysis. In both analyses, Galeocerdo (the tiger shark), Rhizoprionodon, Sphyrna, Loxodon (the slit-eye shark), Negaprion (the lemon shark), and Triaenodon (the reef white-tip shark) were placed outside the genus Carcharhinus. In a Wagner distance tree, Rhizoprionodon and Galeocerdo form together a basal place, with a 95% of the maximum parsimony trees. In both analyses, the four species of sharpnose sharks Rhizoprionodon form a monophyletic cluster branching. The hammerhead sharks also form a monophyletic group. This result was supported by phylogenetic DNA analysis as well [7]. The slit-eye shark, Loxodon macrorhinus, the reef white-tip shark, Triaenodon obsesus, and the lemon shark, Negaprion brevirostris, all branch separately [1].
Positioning of the blue shark Prionace glauca resulted interestingly from both analyses. Because, when the blue shark was not included, the genus Carcharhinus forms a monophyletic group in both analyses. The allozymes data analysis by Naylor [4] determine that the Carcharhinus genus is monophyletic, Prionace being the closest sister taxon to the monophyletic Carcharhinus group, and Negaprion being basal to this group [8]. However, in Wagner distance analysis, it is still unclear whether which one out of Negaprion or Prionace is the closest taxon to the Carcharhinus monophyletic group. When the blue shark was included, the paraphyletic genus Carcharhinus comprises a large clade, including the blue shark, P. glauca. It must be underlined that independent morphological and fossil evidence supports the hypothesis that the blue shark may be a derived species from the genus Carcharhinus. Compagno [9] suggested that there should be a possible link between P. glauca and the obscurus group of sharks at the teeth level. However the first-appearance time of the species based on teeth fossils does not provide a clear answer, because Carcharhinus' first appears during the Middle Eocene, whereas P. glauca does not appear until Pliocene times [1].
Carcharhiniform sharks also have an excellent fossil tooth record. These records show Galeocerdo cuvieri (the tiger shark) first recorded in Lower Eocene times (50–56 Myr). The divergence between Sphyrna, Carcharhinus and Negaprion occurred about 38 Myr ago, in Late Eocene times. The first appearance of Rhizoprionodon occurred in the Lower Eocene, and that of Sphyrna in the Lower Miocene [1,10].
Cytochrome oxidase I (COI) gene sequences were used to find a better answer to the phylogenetic relationship among genera Carcharhinus, Prionace, Negaprion, Triaenodon, Rhizoprionodon and Sphyrna, using Galeocerdo as an outgroup. In this gene analysis, Sphyrna joins the Triaenodon, both join Carcharhinus, Prionace, and Negaprion clades as sister genera [11].
Many species of the genus Carcharhinus are very similar to each other and very easily confused. Many hypotheses were proposed for the interrelationship among the genus Carcharhinus. Firstly, Carcharhinus divided into two forms, smooth-backed forms, and ridge-backed forms by Springer [12,13]. He also divided into ridge-backed forms, then into Eulamia, with first dorsal and pectoral tips, and Pterolamniops, with rounded tips. Garrick [14] suggested that C. obscurus and C. galapagensis, are the centre of the group, called an obscurus group, which includes large ridge-backed sharks with broad triangular teeth, such as C. altimus, C. plumbeus, C. longimanus and C. perezi, in spite of the absence of triangular teeth. With this proposal, C. sorrah, C. falciformis, C. albimarginatus and C. sealei were excluded from Springer's Eulamia group, but, despite C. longimanus has rounded fin morphology, it was included within this group. Compagno [9] agreed with Garrick, who suggested including C. albimarginatus into this group, instead of C. perezi. The allozyme data by Naylor [1] suggested that C. obscurus, C. galapagensis, C. longimanus, C. falciformis, C. plumbeus, C. altimus, and C. perezi all form a monophyletic group, including also P. glauca and all members of the large ridge-backed group [1].
Garrick [14] and Compagno [9] have both proposed some sub-groupings within the Carcharhinus genus, based on morphological similarities. Both of them proposed that C. porosus is a close ally of the C. dussumeri–C. sealei group. This relationship was supported by the Wagner distance tree in Naylor's study [1], but cladistic character analysis does not also support the inclusion of C. macloti and C. sorrah into this group, which was not supported by Naylor either [1].
C. albimarginatus was closely related to C. amblyrhynchos and C. wheeleri based on similar tooth shape and morphological and vertebral character similarities, according to Garrick [14]. However, this relationship was not established in the cladistic analysis by Naylor [1]; in spite of that, the same result was obtained through Wagner distance analysis in the same study. In addition, Naylor [1] cladistic analysis does not support Compagno's suggestion that C. perezi is the sister group of C. amblyrhynchos and C. wheeleri. The close relationships between C. limbatus, C. amblyrhynchoides, and C. brevipinna suggested by Garrick and Compagno was not supported by Naylor's allozyme data analysis [1]. Naylor explained their behaviour similarities, their similar body shape and colour pattern tooth shape by a convergent evolution, instead of a close relation [1].
The recent similar allozyme research was carried out by Lavery [15]. He used 17 species of carcharhinid sharks and four closely related species, collected from Australia. In his study, drawing a most-parsimonious tree with 215 steps, the monophyly of the genus Carcharhinus was not supported. The genus was found paraphyletic, and included two species from other genera (Negaprion acutudiens and Galeocerdo cuvieri), and one species from a different family (Hemipristis elongatus). However, when he used 219 steps, which means an increase of the length, he found a monophyletic genus Carcharhinus. Both results were not repeated. In the allozyme study by Naylor [1], both Wagner distance analysis and character analysis indicate that the family Carcharhinidae is paraphyletic and includes the hammerhead shark (genus Sphyrna) [1].
In another way, a very new molecular phylogenetic study was carried out with mitochondrial aligned sequences and nuclear RAG1 sequences, aiming to clarify the relationships within Carcharhiniformes with a special focus on the two most problematic groups: scyliorhinids and triakids by Iglésias. The strict consensus of 12 MP trees calculated from the mtDNA data set (partial 12S rRNA, complete Valine tRNA and 16S rRNA genes for 45 species) was used for an analysis that indicated that Negaprion is a sister group of Carcharhinus [1], whereas Compagno [9] and Lavery [15] found that Negaprion nested within Carcharhinus [16].
This study was carried out for a better understanding of the phylogenetic relationships among the species of the genus Carcharhinus, via using ribosomal ITS1–2 regions.
Nuclear ribosomal RNA cistron (rDNA) or portions of them were widely used in phylogenetic studies. In the cistron, the rRNA genes repeated tandemly in numerous copies, and rapid concerted evolution occurred in this gene family. Especially, in the sequencing of this cistron, ITS1–2 regions offer a great opportunity for determining the phylogenetic relationship among closely related species [17]. For that reason, ITS1–2 regions were used in many research works, not only in sharks. Sequences of the ITS regions are ideal candidates for molecular evolutionary and systematic studies, and are recently getting more attention. ITS regions evolve fast, and there might be a variation between species within the genus or among the populations. By using the ITS regions, it is possible to determine the nature of the potential variation [18–20].
2 Materials and methods
2.1 DNA sources
A total of seven species of the genus Carcharhinus, one species of the genus Prionace, and one species of the genus Galeocerdo, the ribosomal ITS1 region, were amplified, the last species being also used as an outgroup. For ITS2, a total of 11 species of the genus Carcharhinus, three species of the genus Prionace, one species of the genus Negaprion, one species of the genus Rhizoprionodon, and one species from the genus Galeocerdo, which was used as an outgroup, were amplified (Tables 1 and 6). All the samples were provided by Dr. Mahmood Shivji, Nova Southeastern University, Florida.
List of the species considered in this study [21]
Species name | Common name | PCR fr. size (ITS1) | (ITS2) |
C. altimus | Bignose | 1.8 kb | 1.4 kb |
C. brevipinna | Spinner | 1.8 kb | 1.4 kb |
C. acronotus | Blacknose 1.8 kb | 1.4 kb | |
C. falciformis | Silky | 1.8 kb | 1.4 kb |
C. isodon | Fine tooth 1.8 kb | 1.4 kb | |
C. leucas | Bull | – | 1.4 kb |
C. limbatus | Black tip | 1.8 kb | 1.4 kb |
C. obscurus | Dusky | – | 1.4 kb |
C. plumbeus | Sandbar | – | 1.4 kb |
C. perezi | Caribbean reef | – | 1.4 kb |
C. porosus | Small tail | 1.8 kb | 1.4 kb |
C. R. terraenovae | Atsh nose | – | 1.4 kb |
C. P. glauca | Blue | 1.8 kb | 1.4 kb |
C. N. brevirostris | Lemon | – | 1.4 kb |
C. G. cuvier | Tiger | 1.8 kb | 1.4 kb |
List of the ridge-backed and smooth-backed species used in this study [29]
Ridge-backed species | Smooth-backed species |
Caribbean reef | bull |
sandbar | blacknose |
big nose | fine tooth |
silky | spinner |
dusky | small tail |
black tip | |
blue | |
lemon | |
Atlantic sharpnose |
Some of the samples arrived as genomic DNA, already extracted. Others were sent as about 1 to 5 g of liver or muscle tissue samples in 70% ethanol. All the samples were kept in a refrigerator. Tissue samples were later submitted to extraction to obtain genomic DNA.
2.2 DNA extraction and PCR amplification
Either, a phenol/water/chloroform extraction method, based on the ABI manual DNA extraction kit, or the QIAamp tissue kit method from QIAGEN Company was used for DNA extraction. All buffers and materials that extraction requires were included in the kit. 0.2 to 0.5 g of tissue, for the first method, and about 25 mg of tissue, for the second one, provided approximately 0.2–1.2 mg of DNA. After extraction, Genomic DNA was stored at 4 °C in a refrigerator.
The ribosomal internal transcribed spacer ITS1–2 was amplified by using the polymerase chain reaction (PCR) with the primers listed in Table 2. For ITS1, 18S3 and 5.8S5 were used in PCR. Internal primers were used in a sequencing reaction for this region.
Primers used in this chapter for PCR amplification and sequencing of ITS1–2 region. L-strand primer forward and H-strand for reverse primer
18-S2 L | TAGAGGAAGTAAAAGTCGTAACAAGGTTTC | ITS1 |
18-S3 L | GTAGGTGAACCTGCGGAAGGATCATT | ITS1 |
5.8-S4 H | TTCATCGATCCACGAGCCGAGTGAT | ITS1 |
5.8-S5 H | GGTGTTCATGTGTCCTGCAGTTCACATT | ITS1 |
ITS2F L | CTACGCCTGTCTGAGTGTC | ITS2 |
ITS2R H | ATATGCTTAAATTCAGCGGG | ITS2 |
For all PCR amplifications, either a PerkinElmer DNA thermal cycler 480 or a PTC-100TM programmable thermal controller (MJ Research, Inc.) was used. The same two primers were used for both PCR amplification and sequence of the ITS2 region. PCR amplification in the ITS1 region was carried out with external primers, 18S3 and 5.8S5. In the sequencing of the ITS1 region, the same primers and internal primer, 5.8S4, were used. After extraction from the tissue sample, the double-stranded genomic DNA was used in 30 cycles of PCR amplification. After the five-minute initial denaturing at 94 °C, the sample was kept at 94 °C for 1 min at 94 °C, then annealed for 1 min at 50–55 °C; this was followed by 2-min extension at 65 °C or 3-min annealing plus extension at 60–65 °C, after 30 cycles of final 10-min extension at 65 °C. The 50-μl reaction medium contains 200 ng μ1−1 of two external primers, 10 mM of each nucleotide (dATP, dCTP, dGTP and dTTP) ultrapure dNTP set from Pharmacia Biotech, 17.5 mM of MgCl2 and 1.75 U of Taq polymerase (Boehringer, Mannheim) or Tag polymerase (ExpandTM High Fidelity PCR system, Boehringer, Mannheim) and at least 300 ng of genomic DNA. After that, this PCR product was run out on a 0.7% agarose gel, stained with an ethidium bromide solution, visualised under low-intensity ultraviolet light and photographed. After determining the approximate size of this fragment, PCR sample was run on a 1% low melt agarose gel. Then the band was visualised under low intensity ultraviolet light, excised and melted in a 65 °C heating block. This excised amplified band amplified again with same PCR technique by using same or internal primers. The final fragments were purified for sequencing by using the highly pure PCR product purification kit (Boehringer, Mannheim), according to the kit's user guide.
2.3 Sequencing and phylogenetic analysis
Either a PerkinElmer DNA Thermal Cycler 480 or a PTC-100TM programmable thermal controller (MJ Research, Inc.) was used in a cycle sequencing reaction. The PCR-amplified products were sequenced directly by using the dideoxy chain termination method. 20 μl of dye-termination reaction medium contain 300–500 ng of purified PCR-amplified product, 5 pmol of each primer and 20 mM of MnSO4. The cycle includes first denaturation at 96 °C for 1 min, followed by 25 cycles of 50 s at 96 °C, 4 min at 62 °C, and 20 s at 50 °C. After that, the reactions were loaded onto ABI 373A automated sequencers. Then, 300–400-bp-long good- quality sequencing was obtained by using each primer every time; new internal primers were designed differently for each species to complete the sequencing of all fragments.
The sequences were loaded into the Eyeball sequence editor [22]. The best alignment was constructed and used in a tree construction.
The data were analysed by maximum parsimony (MP), neighbour-joining (NJ) methods within the PHYLIP 3.5c [23] and maximum likelihood (DNAML) with PUZZLE [24] quartet-puzzling approach. Both MP and NJ analyses' reliabilities were later tested by bootstrapping [25] with 1000 replications of the data. The Kimura 2-parameter distance matrix method was used for NJ analysis, with a transition: transversion ratio of 2:0 as in DNAML analysis, by randomising10 times the input order.
3 Results
The analysis of the molecular data obtained from the ribosomal ITS1, ITS2, ITS1–2 regions was carried out. The sequence alignments of 1938 bp, for the ribosomal ITS1 region with 10 species data, 1590 bp for the ITS2 region, including 18 species data, and 3538 bp for ribosomal ITS1–2 (10 species) were used together for tree construction. In ITS1 data, Galeocerdo cuvieri (tiger shark) 300 bp, Negaprion brevirostris (lemon shark) 80 bp, C. acronotus (blacknose shark) 50 bp are missing. In ITS2 data, C. acronotus (blacknose shark) ∼50 bp and C. perezi (Caribbean reef) 100 bp are missing. For each of them, the data had a high GC-content (about 70%, 80%, 75%).
In all data, Galeocerdo cuvieri (tiger shark) was chosen as an outgroup. In ITS1 data, both maximum parsimony and NJ (Kimura) analysis placed Prionace glauca within the genus Carcharhinus. In maximum parsimony analysis, C. bervipinna and C. porosus was placed outside of the other the genus Carcharhinus species. After C. porosus, two main clades are seen, on the one hand P. glauca, C. limbatus, C. isodon and C. acronotus clades, on the other hand C. altimus and C. falciformis clades. P. glauca was classified as a different genus that belongs to the Carcharhinus in the commonly accepted classification. P. glauca position in that clade was supported with 442 and 431 bootstrap values (MP and NJ analysis), but not very strongly.
ITS2 data maximum parsimony and NJ (Kimura) analysis also puts forward Prionace with two more species, collected in California and in Australia, within the genus Carcharhinus. In ITS2 data maximum-parsimony analysis, after the R. terraenovae, the C. porosus–C. isodon clade separates and comes on the outside of all the other species belonging to the genus Carcharhinus; then two main clades is seen. On the one hand, C. falciformis–C. obscurus, C. altimus–C. plumbeus and C. perezi with three species of the P. glauce clade, on the other hand C. limbatus, C. brevipinna, N. brevirostris, and C. leucas–C. acronotus clades are seen. This result indicates also that N. brevirostris must be placed with the genus Carcharhinus, even if it is classified in a different genus.
ITS1–2 combined data maximum-parsimony, NJ and DNAML analysis also placed P. glauca within the genus Carcharhinus. Maximum parsimony analysis indicates that P. glauca joins to the clade including C. altimus and C. falciformis (960), and placed within the clade including C. isodon and C. porosus (620) (Figs. 1–3).
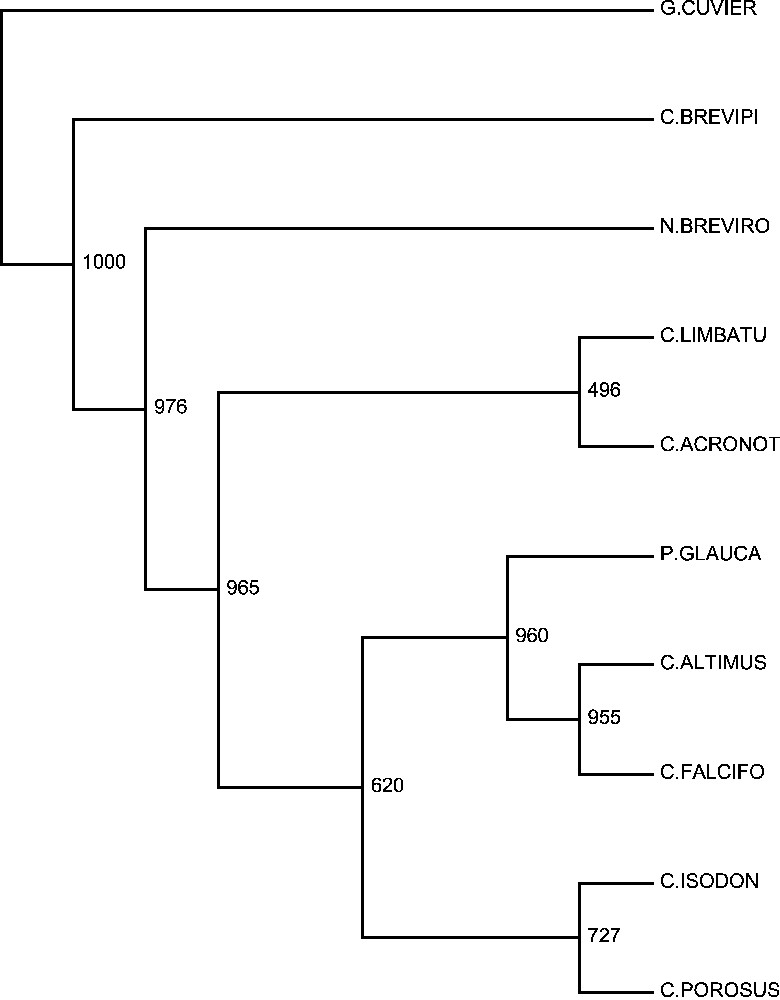
Majority consensus bootstrap tree of the maximum parsimony analysis for the ribosomal ITS1–2 combined data, sequenced for representing the species of the genus Carcharhinus (10 species).
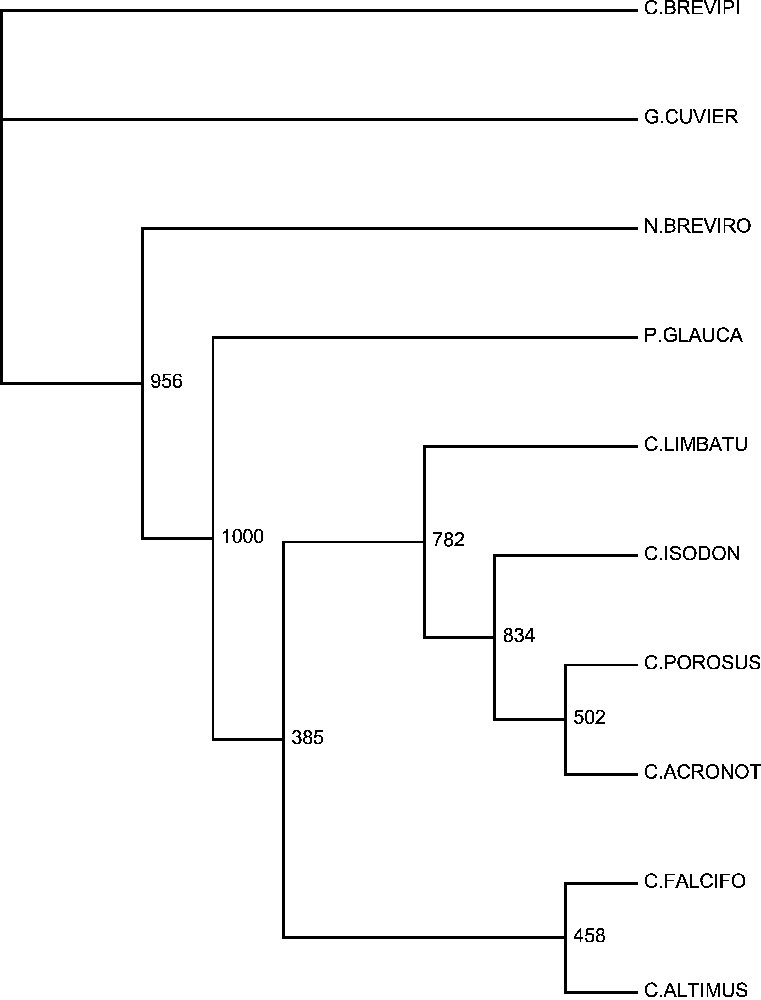
Majority consensus bootstrap tree of the NJ (Kimura) analysis for the Ribosomal ITS1–2 combined data, sequenced for representing the species of the genus Carcharhinus (10 species).
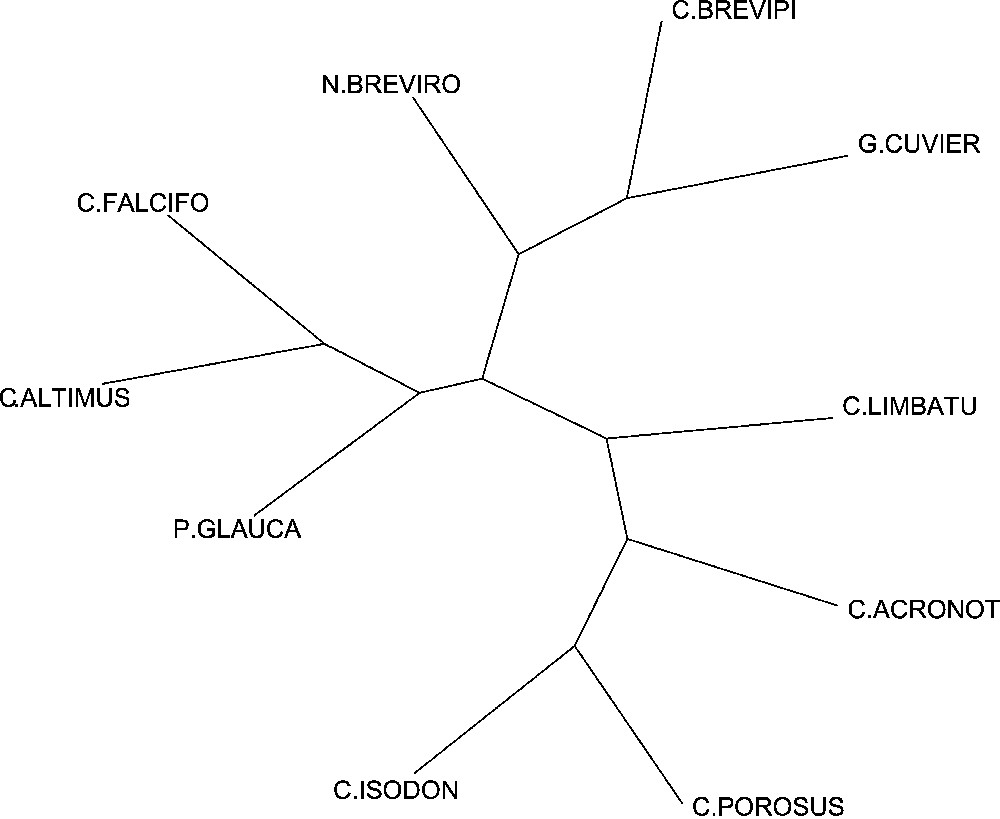
Maximum-likelihood tree (DNAML) with PUZZLE for the ribosomal ITS1–2 combined data, sequenced for representing the species of the genus Carcharhinus (10 species).
The table of the sequence divergence of the ITS2 region indicates that the sequence divergence between blue sharks from Australia, California, and New York is about 1.5–2%, that between the blue sharks, genus Prionace, and the genus Carcharhinus range being about 4–7.5%, which is about the same sequence differences as within the genus Carcharhinus (Table 5). Only exception, very high sequence divergence values were astonishingly obtained between P. glauca species and C. isodon (13–14%), and between C. porosus (26–27%). The sequence divergences are between the species of the genus Carcharhinus, including three species of P. glauca (Blue shark) and S. mokarran 18–30%, N. brevirostris 8–25%, R. terreenovae 21–42%, and G. cuvieri 20–39%. This indicates that the most divergent member of these data is R. terreenovae.
Sequence divergence values for the ribosomal ITS2 region of some Carcharhiniformes species
G.CUVIER | 0.196 | 0.397 | 0.217 | 0.229 | 0.218 | 0.212 | 0.267 | 0.228 | 0.312 | 0.217 | 0.233 | 0.235 | 0.223 | 0.235 | 0.203 | 0.309 | 0.209 |
C.ALTIMUS | 0.243 | 0.035 | 0.038 | 0.034 | 0.014 | 0.12 | 0.045 | 0.213 | 0.047 | 0.030 | 0.040 | 0.076 | 0.052 | 0.030 | 0.186 | 0.041 | |
C.POROSUS | 0.259 | 0.269 | 0.273 | 0.249 | 0.311 | 0.247 | 0.424 | 0.219 | 0.253 | 0.248 | 0.241 | 0.259 | 0.231 | 0.395 | 0.256 | ||
P.GLAUCAJ3 | 0.014 | 0.015 | 0.045 | 0.130 | 0.074 | 0.233 | 0.074 | 0.057 | 0.067 | 0.095 | 0.076 | 0.043 | 0.198 | 0.065 | |||
P.GLAUCAS2 | 0.018 | 0.048 | 0.140 | 0.075 | 0.236 | 0.075 | 0.059 | 0.069 | 0.102 | 0.080 | 0.046 | 0.204 | 0.065 | ||||
P.GLAUCA | 0.036 | 0.135 | 0.065 | 0.237 | 0.066 | 0.050 | 0.060 | 0.100 | 0.072 | 0.037 | 0.194 | 0.059 | |||||
C.PLUMBEU | 0.127 | 0.045 | 0.212 | 0.056 | 0.032 | 0.042 | 0.085 | 0.056 | 0.035 | 0.189 | 0.047 | ||||||
C.ISODON | 0.152 | 0.312 | 0.146 | 0.137 | 0.138 | 0.154 | 0.149 | 0.123 | 0.272 | 0.135 | |||||||
C.LEUCAS | 0.238 | 0.053 | 0.054 | 0.069 | 0.077 | 0.048 | 0.055 | 0.205 | 0.056 | ||||||||
R.TERRAEN | 0.224 | 0.224 | 0.239 | 0.257 | 0.239 | 0.233 | 0.287 | 0.234 | |||||||||
C.ACRONOT | 0.063 | 0.064 | 0.084 | 0.062 | 0.067 | 0.216 | 0.064 | ||||||||||
C.OBSCURUS | 0.043 | 0.090 | 0.060 | 0.041 | 0.193 | 0.048 | |||||||||||
C.FALCIFOR | 0.098 | 0.069 | 0.053 | 0.208 | 0.050 | ||||||||||||
N.BREVIROS | 0.088 | 0.071 | 0.272 | 0.091 | |||||||||||||
C.BREVIPIN | 0.062 | 0.218 | 0.062 | ||||||||||||||
C.PEREZI | 0.207 | 0.049 | |||||||||||||||
S.MOKARRAN | 0.197 | ||||||||||||||||
C.LIMBATUS |
The table of the sequence divergence of the ITS1 region indicates that the sequence divergence between the blue shark, the genus Prionace, and the genus Carcharhinus is about 4–9%, which is a difference similar enough to that within the genus Carcharhinus (Table 4). Again, the obtained sequence divergence values were very high, all other species of the genus Carcharhinus including P. glauca to C. brevipinna and C. porosus (15–17%). C. brevipinna was placed on the outside of all the other species as a most divergent member. The table of the sequence divergence of the ITS1–2 regions combined data indicates almost the same result with ITS1 region. The sequence divergence of all other species showed that C. brevipinna (56–82%) is the most divergent member, then C. porosus (20–23%), followed by N. brevirostris (14–30%). The sequence divergence between P. glauca and other species of the genus Carcharhinus, rather than between most different ones, is about 5–9%, whereas it is 5–10% within the genus Carcharhinus (Table 3).
Sequence divergence values for the ribosomal ITS1 regions of some Carcharhiniformes species
C.BREVIPI | 2.37 | 2.29 | 2.36 | 2.08 | 2.67 | 2.11 | 2.03 | 2.29 | 2.04 |
C.LIMBATU | 0.05 | 0.06 | 0.07 | 0.16 | 0.07 | 0.19 | 0.04 | 0.37 | |
C.ALTIMUS | 0.05 | 0.07 | 0.16 | 0.09 | 0.19 | 0.05 | 0.39 | ||
C.FALCIFO | 0.07 | 0.15 | 0.08 | 0.20 | 0.06 | 0.38 | |||
P.GLAUCA | 0.17 | 0.08 | 0.21 | 0.05 | 0.36 | ||||
C.POROSUS | 0.18 | 0.31 | 0.15 | 0.56 | |||||
C.ACRONOT | 0.21 | 0.05 | 0.41 | ||||||
N.BREVIRO | 0.18 | 0.46 | |||||||
C.ISODON | 0.37 | ||||||||
G. CUVIER |
Sequence divergence values for the ribosomal ITS1–2 regions together with those of some Carcharhiniformes species
C.BREVIPI | 0.58 | 0.57 | 0.59 | 0.56 | 0.82 | 0.57 | 0.61 | 0.69 | 0.71 |
C.LIMBATU | 0.05 | 0.06 | 0.07 | 0.20 | 0.06 | 0.16 | 0.08 | 0.29 | |
C.ALTIMUS | 0.05 | 0.05 | 0.20 | 0.07 | 0.14 | 0.09 | 0.28 | ||
C.FALCIFO | 0.07 | 0.20 | 0.08 | 0.15 | 0.10 | 0.31 | |||
P.GLAUCA | 0.23 | 0.08 | 0.16 | 0.09 | 0.29 | ||||
C.POROSUS | 0.20 | 0.30 | 0.22 | 0.47 | |||||
C.ACRONOT | 0.16 | 0.09 | 0.33 | ||||||
N.BREVIRO | 0.17 | 0.35 | |||||||
C.ISODON | 0.32 | ||||||||
G.CUVIER |
4 Discussion
Prionace usually separate from Carcharhinus by their first dorsal base, much closer to the pelvic bases than the pectorals. Some species from the genus Carcharhinus, such as C. falciformis and C. obscurus, have a closer first dorsal base, but still more anterior than in Prionace. Also, blue sharks have some different morphologic characters from those of Carcharhinus, like having a big upper tooth, very long pectoral fins, and a first dorsal fin origin well behind the rear angle of the pectoral fin, and dermal gill rakers [9]. Exceptionally, papillose gill rakers are present on gill archers. The weak lateral keels present on caudal peduncle and spiracles are absent in Prionace. The eyes are large, without posterior notches. Unlike other carcharhinids, clasper growth in males is apparently a prolonged and gradual process that may take at least a year, making the condition of claspers rather difficult to be used for determining the maturation of males in Prionace [21]. Also, the reproduction modes are slightly different between Prionace and the genus Carharhinus (including Negaprion), as being live-bearing, matrotropic and plecanta in genus Carharhinus, while live-bearing matrotropic and plecanta & trophonemata in Prionace are seen [26]. The colour is different compared to that of other Carcharhinus, being dark indigo blue at the top, shading to bright blue on the sides; undersides are white, while in the genus Carcharhinus the colour is generally brown, blue, bronze or olive [21].
However, in contrast to these morphological differences, our molecular results placed the blue shark (Prionace glauca) within the genus Carcharhinus, instead of making it a separate genus. According to Jordan and Evermann [27], the blue shark (P. glauca) and Carcharhinus were not separated from each other. Cantor [28] used Prionace to replace the preoccupied subgenus Prionodon, which was another type of Prionace, and this led to find out similarities between Prionace and Carcharhinus [9]. The recent studies about the connection between P. glauca and the genus Carcharhinus gives different opinions. For example, first-appearance time based on teeth fossil shows that there is not enough evidence to ascertain that P. glauca does not separate from the genus Carcharhinus, which was first seen in the Middle Eocene, while P. glauca does not appear until Pliocene times. However, at the opposite of this, the idea that the blue shark might be a derived species from the genus Carcharhinus was supported by some independent morphological and other fossil evidence. In addition, a possible teeth link between P. glauca and obscurus groups of sharks was put forward by Compagno [1,9].
By using the molecular data, ITS2 data analysis indicates that all three species of P. glauca form a monophyletic group, placed within the genus Carcharhinus group. Also both NJ and MP analyses indicate that C. perezi joins to the P. glauca clade and form a monophyly with 548 and 811 bootstrap values. For ITS1 only and ITS1–2 combined data, 10 species were used and MP analysis placed P. glauca within the genus Carcharhinus, supported by 529 and 620 bootstrap values. Species C. perezi was not used in this analysis.
All molecular data from MP and DNAML analysis, and some NJ analysis agreed that P. glauca has a place within the genus Carcharhinus. Also, sequence divergence values, between and within P. galuca and the genus Carcharhinus, go in this direction.
The allozyme data of Naylor [1] indicates that C. obscurus, C. galapagensis, C. longimanus, C. falciformis, C. plumbeus, C. altimus, and C. perezi all form a monophyletic group, including also P. glauca and all the members of the large ridge-backed group.
According to Martin's analysis of the mitochondrial cytochrome b genes PAUP and NJ [11], Prionace are positioned in a large group, including also most of the species of the genus Carcharhinus, while in cytochrome oxidase I (COI) gene analysis, Sphyrna joins to Triaenodon, and both join Carcharhinus, Prionace, and Negaprion clades as sister genera.
Our results and those of other researchers indicate that there is high probability that P. glauca is not a different genus, as in the accepted classification. P. glauca's position within the genus Carcharhinus is supported by most of our analysis. But still, some diverging results as well as the first-appearance time based on teeth fossil makes us suspicious about the actual place of P. glauca.
These morphological and molecular different outcomes about the position of the Prionace pave the way to a future discussion of a more detailed evaluation.