1 Introduction
Reproduction mechanisms and species evolution are, according to the biological concept of species, intertwined [1]. Though echinoids are mainly broadcasting species, hybridization and introgression are rare phenomena, even in sympatric congeneric species, indicating efficient reproductive isolation [2]. There are two types of reproductive barriers, pre-zygotic and post-zygotic [3–5]. Pre-zygotic reproductive isolation prevents fertilization from occurring by differences in habitat, reproduction dynamics, behavioural or mechanical isolation. In contrast, post-zygotic reproductive barriers correspond to decreased viability or fertility of interspecific hybrids. When post-zygotic barriers exist for sympatric species, pre-zygotic barriers should evolve rapidly as a result of natural selection [6–10].
In echinoderms, both reproductive cycles and reproductive isolation have been extensively studied [2,11], and spawning asynchrony has been suggested as a response to avoid potential hybridization [12–18].
Our study aims to explore reproduction timing and tuning in the light of genetic relationship in different species of the genus Echinocardium (Loveniidae, Spatangoida), a widespread irregular sea urchin genus, with three species observed in the Mediterranean Sea, Echinocardium cordatum (Pennant, 1777), Echinocardium mediterraneum (Forbes, 1844) and Echinocardium capense (Mortensen, 1907).
E. cordatum is one of the most widely studied spatangoids, in biology [19–29], ecology [30–38], development [39–42] and morphometry [43–45] as well as in genetics [46–49]. Yet our understanding of the evolutionary history of this taxon remains partial. The taxonomic validity of several morphologically close species has been a matter of debate for years [45,49] and Echinocardium fenauxi (Péquignat, 1963) in the Mediterranean Sea, Echinocardium australe (Gray, 1855) and Echinocardium zelandicum (Gray, 1855) in the southern Pacific were finally synonymised as morphotypes of E. cordatum (on the basis of morphological [45] as well as molecular [49] data). Inhabiting the Atlantic and the Pacific oceans as well as the Mediterranean Sea, E. cordatum was considered a cosmopolitan species. However, genetic analyses based on both mitochondrial and nuclear DNA revealed that the nominal species E. cordatum (regrouping the former E. cordatum, E. fenauxi, E. austral and E. zelandicum) is truly a complex of separated genetic entities (which do not entirely correspond to the synonymised species) with at least four different cryptic species, presenting statistical differences in morphology, encountered in the Atlantic (A, B1), Pacific (P) and Mediterranean (B1, B2) basins [46,47]. Concerning European seas, species A and B were estimated to have diverged for at least 6.2 myrs and B1 and B2 for at least 2.5 myrs [47]. Nuclear genetic markers (sequence data from an intron of EF1α, and a domain of ribosomal DNA, D8) established that species A and B1 exchange genes in NW Spain (Atlantic) whereas, in the Mediterranean, B1 and B2 do not [46,47].
The reproductive biology of E. cordatum has been investigated since the early 20th century [22]. Gametes are broadcast and fertilization is external, leading to a planktotrophic pluteus larva remaining several weeks in the water column before undergoing metamorphosis. The reproductive cycle has been studied in the Atlantic Ocean (Scottish Sea [22]), the English Channel [26], the Pacific Ocean (Sea of Japan [29] and Seto Inland Sea [29,50]) but only scarce information is available for the Mediterranean Sea [51,52]. Fenaux [51] studied the abundance of the larvae of 13 different species of echinoids in Villefranche-sur-Mer Bay (Ligurian Sea) during the years 1960 to 1964 and reported that larvae of E. cordatum or E. mediterraneum, which were not distinguished (only the first larval stage of E. mediterraneum is described), occurred throughout the year, except in December, with a peak of abundance from February to April. The various studies performed highlighted fine variations in the reproductive cycle dynamics around the geographical range. To disentangle the relative influence of endogenous and exogenous factors, data on E. cordatum's reproductive cycles in places where different cryptic species are found in syntopy seem essential. Cryptic species B1 and B2 live in syntopy in Marseille area (south-eastern French coast of the Mediterranean Sea). Though they are the most recently diverged, and thus the least genetically distant, the absence of hybridization reveals efficient reproductive isolation, possibly linked to discrepancies in reproductive behaviour.
The aim of the present study was to characterize more precisely the reproductive cycle of both nominal species E. mediterraneum and E. cordatum in the Mediterranean Sea, to compare the cycles among the cryptic species of E. cordatum in their different geographical areas and to investigate possible correlations with environmental factors.
2 Material and methods
2.1 Specimen collection
Adults of both Echinocardium cordatum and Echinocardium mediterraneum (length exceeding 25 mm [41]) were collected by scuba-diving during 17 months between December 2006 and May 2008 at Sormiou beach (43°12′36.17″N, 5°25′26.40″E), a shallow and sheltered bay about 6 km east of the city of Marseille. Sampling site depth is between 6 and 8 m on a patch of clean fine sand, surrounded by rocks and Posidonia oceanica beds. Sampling periodicity varied from 1 to 2 months, with some variations according to weather constraints, leading to a total of 229 individuals from 12 temporal samples.
Identification was first conducted using morphological characters [53]. A molecular characterization using the mitochondrial 16S marker was also performed to confirm the morphological identification and to distinguish between the two cryptic species within E. cordatum.
2.2 Tissue preparation
To avoid stress spawning, specimens were anaesthetized as soon as collected using clove essential oil [54].
For histological observations two gonads were fixed in Bouin's fluid for one to two hours. The gonads were then washed and dehydrated in an ascending concentration series of ethanol (70%, 95%, and 100%). A one hour LMR® (sodipro) bath was performed before embedding in Paraplast® (2 baths of one and two hours). Serial sections, 7 μm-thick, were deparaffinised with LMR® and stained using the Masson-Goldner-trichrome method. The sections were observed under a light microscope.
For each individual, tissue samples (spines and gonads) were fixed in 95% ethanol to preserve DNA.
2.3 Histological analysis
We described the reproductive cycle on the basis of a 6-stage scale of gonad maturity defined as follows: stage 1: spent; stage 2: spent empty; stage 3: recovery; stage 4: growth (beginning of gametogenesis); stage 5: premature; stage 6: fully mature and partly spawned (Fig. 1).

Gonad histology of E. cordatum and E. mediterraneum, maturation stages as defined in the study, for females (a) and males (b). Stage 1: Spent stage. Follows the spawning period, few relict gametes still present in the lumen. Presence of nutritive phagocytes. Stage 2: Empty stage. The gonads are empty of all mature gametes, only vacuolated nutritive phagocytes and undeveloped germinal cells are seen. Stage 3: Recovery stage. First appearance of spermatogonia and oogonia. Many nutritive phagocytes are present. Thin layer of differentiating cells on the gonad wall. Stage 4: Growing stage. Beginning of spermatogenesis and vitellogenesis. The layer of maturating germinal cells progresses to the center of the gonad. Stage 5: Premature stage. First appearance of mature gametes (spermatozoids and oocytes) in the lumen of the gonad. Nutritive phagocytes still present. Thick columns of differentiating gametes near the gonad wall. Stage 6: Fully mature and partly spawned. Gonad wall very thin, no nutritive phagocytes present. Lumen more or less packed with mature gametes.
The Maturity Index (MI) determines the average sexual maturity of a population, at a given date and is calculated as follows:
The MI is a reliable source of information on the gonadal activity, the spawning period, the rate of gametogenesis [55] and possible differences in reproductive event timing [26]. After checking the absence of variation between the different gonads of the same individual, the sex-ratio and the MI variation over the sampling period were studied for both E. cordatum (and distinguished cryptic species B1 and B2) and E. mediterraneum.
2.4 Molecular analysis
DNA was extracted on dehydrated spines using the Chelex® 100 chelating resin (Sigma) [56]. The 16S ribosomal gene of the mitochondrial genome was amplified as in Chenuil and Féral [47], slightly modified according to Promega recommendations for the Go Taq® DNA polymerase.
The sequence of this gene is typical of each species of the Echinocardium genus and of the different cryptic species in E. cordatum [47]. Restriction Fragment Length Polymorphism (RFLP) analysis using Mse I enzyme and when necessary HpyCH4 III allowed distinction of the three different species, E. mediterraneum, E. cordatum B1 and E. cordatum B2. Digestion of the 16S mt DNA fragment by Mse I restriction enzyme of all E. mediterraneum haplotypes known to date generated a single discernable 180 bp band profile. For E. cordatum, two different profiles can be obtained, one specific to some B2 haplotypes composed of three bands of 270, 160 and 130 bp; and the other, corresponding to all B1 haplotypes and a few B2, composed of 270, 160 and 50 bp bands. The HpyCH4 III digestion was performed on the individuals with the latter profile, generating two different profiles segregating haplotypes B1 (one strong fragment of 200 bp) and B2 (one strong fragment of 300 bp).
Digestions were performed in a 10 μL reaction mix containing 2 μL of 16S PCR product, one unit of restriction enzyme, 1 μL of 10X corresponding buffer, and when needed 0.1 μL of 100X BSA. The mix was incubated at 37 °C for 6 h and then warmed to 80 °C for 20 minutes. The digestion products were run on an electrophoresis 3% agarose gel for one hour.
2.5 Environmental data
Exogenous factors, particularly photoperiod, lunar cycle, food supply and temperature as well as intra- or interspecific chemical signalling (aggregation, asynchrony between sexes, etc.) are known to influence reproductive cycles and spawning process in echinoderms [11,57–59]. Daily average seawater temperature based upon 24-per-day measurements by Stowaway Tidbits autonomous sensors (precision 0.2 C, resolution 0.15 C) [60] were provided by the MEDCHANGE project for the station “Archipel de Riou – Marseille (France)”, 43°10′22.08″N, 05°23′18.96″E, 5 m depth.
Food supply was assessed via chlorophyll a concentration (in μg.L−1) in the water column. Data were provided by the SOMLIT (Service d’observation en milieu littoral, INSU-CNRS, Frioul, [43°14′30″N, 05°17′30″E]).
2.6 Statistical analysis
The Maturity Index was computed and compared for different situations, between nominal species (E. cordatum vs. E. mediterraneum), between sex for each nominal species, between cryptic species B1 and B2, between sex within each cryptic species, and finally, in males, between B1 and B2 and in females between the same species.
In each case, trend similarity of the two respective MI time series were analysed by means of cross-correlation. This provides a statistical comparison of two series as a function of the time lag between them [61]. In particular, cross-correlation measures how closely the trends of two-time series resemble each other, when one is shifted forward or backward by a specified interval. The cross-correlation coefficient lies between −1 and +1, with zero indicating no correlation between the two series, i.e. no trend similarity. Cross-correlations were performed using the R software (version 2.9.0 [62]).
In addition, exact tests (more accurate than the chi-square test χ2) were performed using the application “Struc” in the Genepop software (100,000 MCMC iterations) [63], on the contingency table of the number of individuals at each maturation stage (Table 1) (comparison of 2 species at 6 stages), for each date considered separately, to compare E. cordatum and E. mediterraneum, as well as (not shown) cryptic species B1 and B2. The very conservative sequential Bonferroni correction of significance levels [64] was performed to correct for multiple testing on the annual dataset.
Number of individuals for each maturation stage per date, per species and per sex.
Date | Clade | Sex | Stage | Total | |||||
1 | 2 | 3 | 4 | 5 | 6 | ||||
21/12/2006 | B2 | ♀ | 3 | 3 | |||||
♂ | 2 | 1 | 3 | ||||||
E. med. | ♀ | 2 | 2 | ||||||
31/01/2007 | B1 | ♂ | 1 | 1 | |||||
B2 | ♀ | 2 | 6 | 8 | |||||
♂ | 1 | 12 | 13 | ||||||
E. med. | ♀ | 3 | 1 | 4 | |||||
15/03/2007 | B1 | ♀ | 2 | 2 | |||||
♂ | 1 | 1 | 2 | ||||||
B2 | ♀ | 10 | 5 | 15 | |||||
♂ | 7 | 4 | 11 | ||||||
E. med. | ♀ | 8 | 3 | 11 | |||||
♂ | 3 | 1 | 4 | ||||||
16/04/2007 | B1 | ♀ | 3 | 3 | 6 | ||||
♂ | 1 | 1 | 2 | ||||||
B2 | ♀ | 2 | 6 | 8 | |||||
♂ | 3 | 1 | 4 | ||||||
E. med. | ♀ | 2 | 3 | 5 | |||||
♂ | 2 | 4 | 6 | ||||||
31/05/2007 | B1 | ♀ | 2 | 1 | 3 | ||||
♂ | 1 | 1 | 2 | ||||||
B2 | ♀ | 1 | 3 | 4 | |||||
♂ | 2 | 3 | 5 | ||||||
E. med. | ♀ | 3 | 3 | ||||||
♂ | 1 | 1 | 3 | 5 | |||||
16/07/2007 | B1 | ♀ | 1 | 1 | |||||
♂ | 1 | 1 | |||||||
B2 | ♀ | 2 | 2 | 1 | 5 | ||||
♂ | 2 | 1 | 3 | ||||||
E. med. | ♀ | 1 | 1 | 2 | |||||
♂ | 1 | 2 | 3 | ||||||
09/10/2007 | B1 | ♂ | 1 | 1 | |||||
B2 | ♀ | 1 | 1 | 1 | 3 | ||||
♂ | 1 | 1 | |||||||
E. med. | ♀ | 1 | 5 | 6 | |||||
♂ | 2 | 2 | |||||||
06/12/2007 | B1 | ♀ | 2 | 2 | |||||
♂ | 2 | 1 | 3 | ||||||
B2 | ♀ | 7 | 7 | ||||||
♂ | 1 | 2 | 3 | ||||||
10/01/2008 | B1 | ♀ | 1 | 1 | |||||
♂ | 1 | 1 | |||||||
B2 | ♀ | 1 | 1 | ||||||
E. med. | ♀ | 4 | 4 | ||||||
♂ | 2 | 1 | 3 | ||||||
13/02/2008 | B1 | ♀ | 1 | 1 | |||||
♂ | 1 | 1 | |||||||
B2 | ♀ | 4 | 4 | ||||||
♂ | 1 | 1 | 1 | 3 | |||||
E. med. | ♀ | 4 | 4 | ||||||
♂ | 4 | 4 | |||||||
02/04/2008 | B1 | ♂ | 2 | 2 | |||||
B2 | ♀ | 5 | 5 | ||||||
♂ | 4 | 2 | 6 | ||||||
E. med. | ♂ | 1 | 1 | 1 | 3 | ||||
06/05/2008 | B2 | ♀ | 1 | 1 | 2 | ||||
♂ | 1 | 2 | 3 | ||||||
E. med. | ♀ | 2 | 2 | 4 | |||||
♂ | 1 | 1 | 2 | ||||||
Total | 11 | 16 | 12 | 55 | 79 | 56 | 229 |
3 Results
3.1 Species and clade composition
The RFLP analysis generates specific patterns of digestion according to the species. For each sample, the species (nominal and cryptic) and sex were identified (Table 1). Of the 229 specimens caught, 152 were identified as E. cordatum (ca two-thirds) and 77 as E. mediterraneum (ca one-third). Within E. cordatum, 21% of the individuals belonged to clade B1 (32 individuals) and 79% to clade B2 (120 individuals). None of the sex-ratios calculated were significantly different from 1 at the 5% level (χ2 test).
Echinocardium populations being scarce around Marseille, it was impossible to increase the number of specimens without threatening the sampled demes.
3.2 Gonadic stage analyses
The relative frequencies of each maturity stage (Fig. 2, Table 1) revealed inter-individual variability of the maturation process in both E. mediterraneum and E. cordatum. Despite our limited sample size, for most of the year 2 to 3 consecutive stages were observed at a given date, and in July 2007 five and four different maturation stages were encountered for E. cordatum and E. mediterraneum respectively. This pattern resulted from the overlap of the end of the first reproductive cycle (2007) and the beginning of the second one (2008) from May to October for E. cordatum and from May to July for E. mediterraneum (Fig. 2).
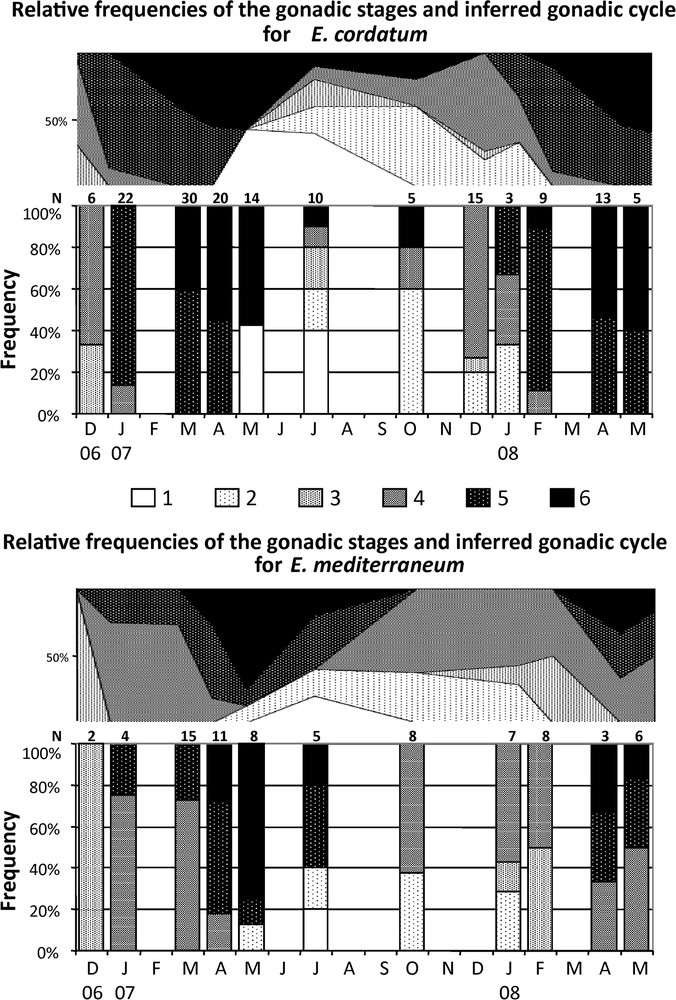
Relative frequencies histograms (stacked graph) and gonadic maturation cycle inferred from these data (area graph), of the 6 gonad stages for E. cordatum (top) and E. mediterraneum (bottom) in Sormiou between December 2006 and May 2008. N: number of individuals sampled at each date.
In E. cordatum individuals initiating their gametogenesis (stage 4) were encountered in December 2006 and January 2007 and from July 2007 to February 2008. Ripe and partly spawned individuals (stage 6) were found from March to October 2007, and from February 2008 to the end of the survey (May 2008). For each date when B2 stage 6 individuals were found, stage 6 B1 individuals were also present whenever the B1 sample exceeded two individuals. For E. mediterraneum, stage 4 individuals were recorded between January and April 2007 and, for the 2008 breeding season, appeared between July 16th and October 09th 2007 and were encountered until the end of the study. Stage 6 individuals were found in 2007 between April and July (Fig. 2), suggesting a shorter spawning period (4 to 6 months depending on sample gaps) for this species compared to E. cordatum, at least for the considered cycle (2007).
Despite the limited sample sizes, the distribution of the individuals in the six maturity stages were significantly different at four dates, between E. cordatum and E. mediterraneum (P < 0.05, exact tests on 2 species × 6 stages contingency tables) (Table 2). Applying the Bonferroni correction for multiple testing, a significant difference between the two species was still revealed for March 2007 and February 2008 (Table 2) meaning that the difference was also supported globally. The same tests revealed no significant difference between cryptic species B1 and B2 (P > 0.05) though this maybe due to the small sample size of B1.
Exact tests of the null hypothesis that E. cordatum and E. mediterraneum samples have similar maturation stage composition (Table 1 [P-value and Standard Error]).
Date | 21/12 | 31/01 | 15/03 | 16/04 | 31/05 | 16/07 | 09/10 | 10/01 | 13/02 | 02/04 | 06/05 |
2006 | 2007 | 2007 | 2007 | 2007 | 2007 | 2007 | 2008 | 2008 | 2008 | 2008 | |
P-value | 0.427 | 0.028 | 0.000* | 0.096 | 0.033 | 0.419 | 0.359 | 0.749 | 0.001* | 0.349 | 0.246 |
S.E. | 0.0019 | 0.0010 | 0.0000 | 0.0025 | 0.0017 | 0.0054 | 0.0035 | 0.0035 | 0.0002 | 0.0039 | 0.0031 |
3.3 Maturity Index analyses
The study of patterns of change of MI throughout the year revealed similar general trends for every studied case (Figs. 3 and 4). MI increased throughout the year, except in spring, when there was a sudden drop in the index. According to literature, an abrupt MI drop corresponds to the beginning of the population spawning season, here, in mid-April 2007 for E. cordatum, and in late May 2007, and April 2008 for E. mediterraneum (Fig. 3). Time series cross-correlation analyses (Table 3) revealed high and significant correlations for all pairs of MI trends considered except between sexes in E. mediterraneum (Fig. 4c) for which the correlation was lower (r = 0.68, Table 3) and not significant. For all situations considered, cross-correlation analyses highlighted maximum correlation values for the zero time lag (Table 3, Fig. 4) except for the comparison between E. cordatum and E. mediterraneum for which cross-correlation peaked at lag one (r = 0.75, P < 0.05, Table 3, Fig. 3). In other words, the MI trends of the two species match when E. mediterraneum MI is lagged one time backward from that of E. cordatum. This corresponds to an observed delay of one and a half months in the MI drop of E. mediterraneum compared to E. cordatum for the 2007 breeding season.
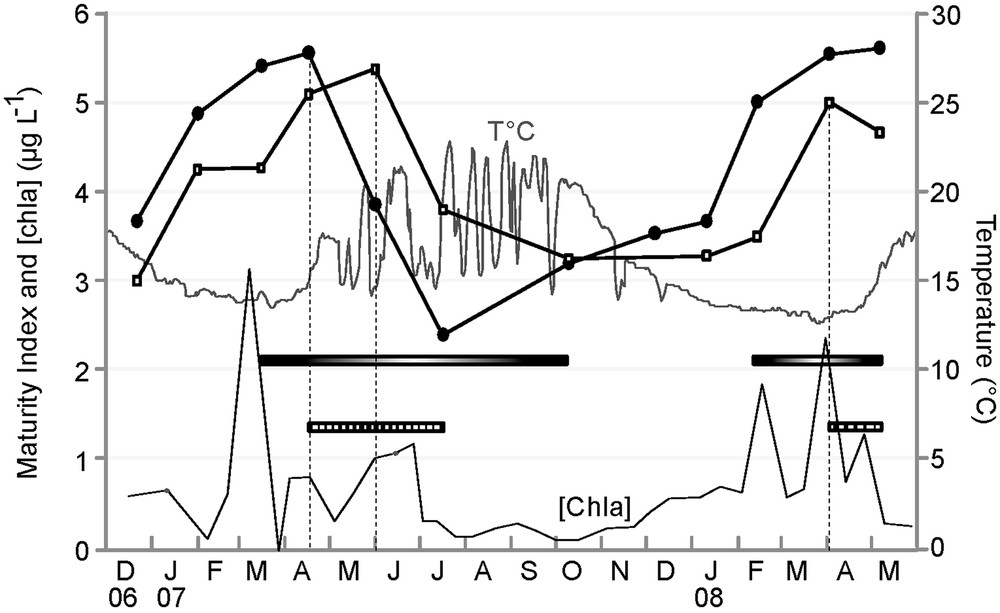
Maturity Index temporal survey of E. cordatum (-●-) vs. E. mediterraneum (-□-), period with ripe E. cordatum, ripe E. mediterraneum, T°C, Chla concentration (μg.L−1).

Maturity Index temporal survey of (a) B1 (-●-) vs. B2 (-□-); (b) males (-□-) vs. females (-●-) in E. cordatum; (c) males (-□-) vs. females (-●-) in E. mediterraneum; (d) males (-□-) vs. females (-●-) in B1; (e) males (-□-) vs. females (-●-) in B2; (f) males of B1 (-●-) vs. males of B2 (-□-); (g) females of B1 (-●-) vs. females of B2 (-□-).
Cross-correlation analysis of Maturity Index time series.
Maturity Index series | Maximal cross-correlation | Respective time lag |
E. cordatum vs. E. mediterraneum (Fig. 3) | 0.75* | 1 |
Clade B1 vs. B2 within E. cordatum (Fig. 4a) | 0.86* | 0 |
♂ vs. ♀ in E. cordatum (Fig. 4b) | 0.78* | 0 |
♂ vs. ♀ in E. mediterraneum (Fig. 4c) | 0.68 | 0 |
♂ vs. ♀ in the clade B1 (Fig. 4d) | 0.79* | 0 |
♂ vs. ♀ in the clade B2 (Fig. 4e) | 0.85* | 0 |
♂ B1 vs. ♂ B2 (Fig. 4f) | 0.97* | 0 |
♀ B1 vs. ♀ B2 (Fig. 4g) | 0.80* | 0 |
Cross-correlation analyses for each sex between E. cordatum and E. mediterraneum confirmed that a time lag of one gives the maximum correlation (not shown).
3.4 Correlation with environmental variables
Marseille seawater temperature has a narrow annual range compared to other temperate regions (Fig. 5).
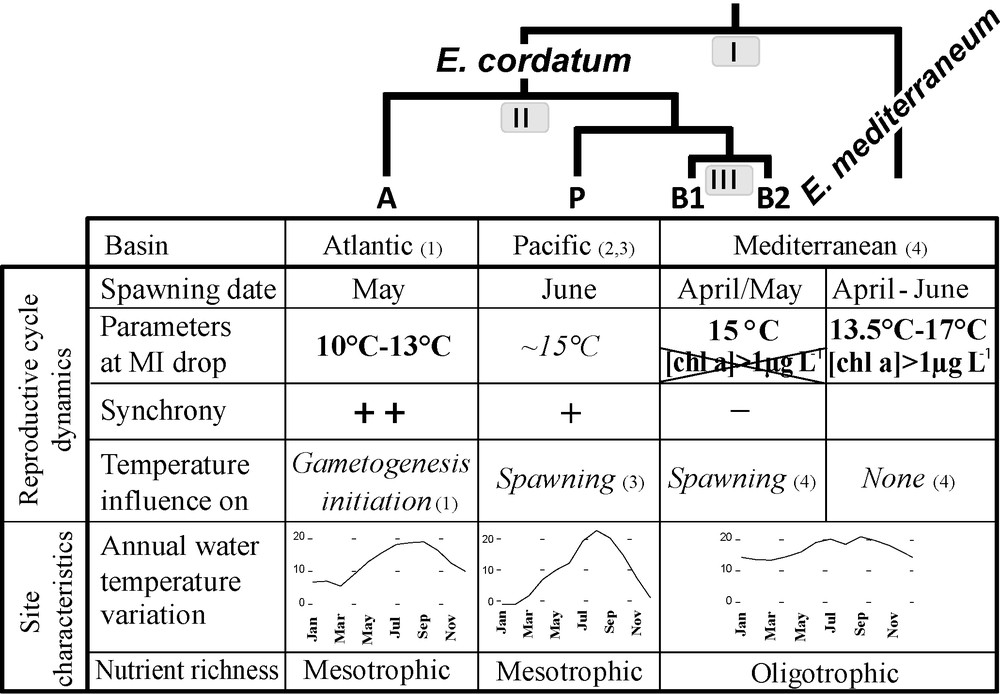
Summary table of reproductive cycle characteristics and environmental parameters in the distinct Echinocardium lineages studied. Bold letters identify well supported results; italic letters refer to suggestions. Mean annual temperature variations of the studied sites are given as indications (data from Wimereux were provided by “Service d’observation en milieu littoral, INSU-CNRS, Wimereux”, and data from Vostok bay by Yakovleva, in press). Node numbers on the cladogram refer to the three parts of the discussion. Numbers in brackets refer to the original study, (1) Nunes and Jangoux, 2004 [26]; (2) Yakovlev, 1987 [29]; (3) Nakamura, 2001 [50]; (4) the present study.
In E. cordatum, the initiation of gametogenesis occurred during the phase of water warming, and last individuals at stage 4 were encountered in periods characterized by a stabilisation of the water temperature at its lowest value (January 2007 and February 2008). Maturity Index dropped in mid-April 2007, indicating that the spawning season began when water temperature rose from 14 °C to 16 °C, but was not recorded in 2008 while water temperature did not overstep 15 °C before May. Ripe and partly spawned individuals were encountered during the whole period of water warming (from March to October, Fig. 2). For E. mediterraneum, the Maturity Index decreased at the end of May 2007, a period characterized by strong seawater temperature variations and a monthly average of 17 °C, and in April 2008 when the water temperature was stabilised at its lowest, around 13 °C.
The Mediterranean Sea is considered as an oligotrophic environment. Though chlorophyll a concentration in the water column remains low almost throughout the year (≤ 3 μg.L−1), it shows seasonal variations. During the study, there were five periods during which chlorophyll a concentration bloomed above 1 μg.L−1 (Fig. 3). The decrease of the Maturity Index coincided with periods of “high” chlorophyll a concentration ([chla] > 1 μg.L−1) for E. mediterraneum (June 2007 and April 2008) but not for E. cordatum. Indeed, E. cordatum's MI dropped in mid-April 2007 when [chla] was still low (< 1 μg.L−1), but not in February/March 2008, when [chla] picked past 2 μg.L−1, despite the presence of stage 6 individuals.
4 Discussion
4.1 Possible differences in environmental determinism for the nominal species E. cordatum and E. mediterraneum (Fig. 5 node I)
E. cordatum and E. mediterraneum displayed similar annual reproductive cycles. Both had a sex-ratio that did not differ significantly from unity, as observed in the English Channel [26], in the Sea of Japan [29] and in the Seto Inland Sea [50]. During the 17 months of the study, they displayed similar Maturity Index trends, though with significant differences. For the first time, a time lag in the annual MI cycle was revealed between the two species. Since the two species occurred in syntopy, the time shift observed might be under endogenous determinism and the influence of exogenous factors should differ.
Fig. 5 summarizes various parameters of the reproductive cycle of the identified genetic entities in the light of their phylogenetic relationships.
As stated by Orton [65], seawater temperature has often been proposed as one of the major factors controlling the timing of marine invertebrates’ reproductive cycles. In E. cordatum it has been thought to have various effects on both gametogenesis and spawning. Nunes and Jangoux [26], on the basis of a 4-year survey of gonad and maturity indices of more than 700 E. cordatum, suggested a triggering role in gametogenesis initiation as well as in ending the breeding season (Fig. 5). “A drop in water temperature followed by a regular increase might initiate gametogenesis” while a temperature higher than 16 °C could be a “threshold cue ending the breeding season” by inhibiting spawning and/or completion of gametogenesis. They observed that spawning occurred when the water was between 10 and 13 °C (Fig. 5). For Yakovlev's data [29], based on almost 800 individuals studied over 8 years, as well as for Nakamura's [50] study of the autoecology of E. cordatum performed between 1998 and 2000, a water temperature of about 15 °C could be a triggering factor of spawning (Fig. 5).
In our study, for the 2007 breeding season, E. cordatum's spawning season began in mid-April, when water temperatures overstepped 15 °C for the first time in the year (Figs. 3 and 5). In 2008, the spawning did not start before April, while the water was still under 14 °C. Yet our data support the observation of Yakovlev (1987) and Nakamura (2001) cited above.
If water temperature influences E. cordatum's reproduction, it may possibly influence that of E. mediterraneum likewise. Yet for this species, the beginning of the spawning season (MI drop) corresponded to very different water temperatures regimes in 2007 and 2008 [monthly average of 17 °C in a highly changing period and monthly average of 13.5 °C in a stable period respectively (Figs. 3 and 5)], making a major role of temperature in the control of spawning unlikely. On the other hand, the beginning of the spawning season for E. mediterraneum concurred with periods of higher chlorophyll a concentration in the water column, i.e. periods of high food supply for the planktotrophic larvae ([Chla] > 1 μg.L−1) (Figs. 3 and 5). In contrast, in 2007, E. cordatum's spawning season started in mid-April, i.e. after the bloom of February and before the spring bloom of June, and in 2008, despite the presence of stage 6 individuals during the chl a spring blooms (from February to May 2008), spawning did not begin until May 2008, ruling out a triggering role of chl a on E. cordatum spawning (Fig. 5). The hypothesis that the availability of phytoplanktonic prey for its feeding larvae could trigger spawning in E. mediterraneum seems plausible as conditions favorable for the development and survival of offspring are often proposed as being the ultimate mediator of reproductive cycles for echinoderms [11,66]. Starr et al. experimentally demonstrated that chlorophyll a could induce gamete release, and that exudates of various phytoplankton species could trigger spawning in Strongylocentrotus droebachiensis [67,68]. Similarly, they noted that in situ spawning in S. droebachiensis in the St Lawrence Estuary initiate when chlorophyll a concentrations increase from low values to significant levels (< 1 mg.m−3) for a period of a few days [69], which is comparable to the threshold we proposed above. However, exogenous control over Echinocardium spp. reproduction need not be restricted to a single factor (but may consist of several factors which interact in synchronizing reproductive activities), even though one may appear dominant. Experiments in controlled environments coupled with a comparative study between the larvae of E. cordatum and E. mediterraneum could highlight possible morphological or behavioural variations that may account for the differences observed [70–72].
4.2 Possible differences in endogenous determinism among E. cordatum cryptic species (Fig. 5 node II)
With the studies of Nunes and Jangoux [26] and Yakovlev [29], an overall view of the reproductive pattern of E. cordatum is now available, from the Atlantic to the Pacific and Mediterranean basins.
The beginning of the spawning season differs between the three biogeographic areas. The Marseille population starts to reproduce earlier in the year (April), compared to Wimereux (May) and to Vostok (June/July). Since these biogeographical areas coincide with the cryptic species distribution (B1 & B2 in the Mediterranean Sea, A in the English Channel, and P in the Pacific [47]), it is impossible to determine whether these differences in reproduction timing are controlled by exogenous factors exclusively (delay in water warming along the geographical range) or if endogenous factors also play a role (intrinsic differences between cryptic species). Indeed, different populations of the same species often have different spawning periods (reviewed in [57], Table III). For example the edible sea urchin Paracentrotus lividus reproduce in April in Tunisia and in May/June in Brittany [55,73]. Unlike E. cordatum, this species is not a complex of cryptic species [74,75], and the time shift reported in reproduction seems to be mainly driven by environmental factors [55].
The synchrony of the reproductive cycles also appears to vary with the locality. In Wimereux, consecutive reproductive cycles only overlapped during a very short period (one month), in contrast to our observations in Sormiou where the two cycles overlapped during 5 months (May to October 2007, Fig. 2). In the same way, ripe individuals were only found during 2 to 3 months in Wimereux (April to June), during 3 to 4 months in the Sea of Japan (March to July, except in some years when some individuals maturated anew in October), whereas in Sormiou, they were present during at least 7 months (from March to October in 2007, Fig. 2). This last result is concordant with the conclusions of Fenaux [51] that larvae of E. cordatum and/or E. mediterraneum were present in the water column virtually throughout the year except in December. The lesser synchrony of E. cordatum individuals in the Marseille area may be explained by the less marked annual temperature cycle in this area (Fig. 5), individuals of the same species being more synchronized in reproduction in regions with marked environmental cycles [13,76–79]. However, we cannot rule out the possibility that the differences in synchrony observed may result from genetic differences between the cryptic species inhabiting the different basins.
A major difference that does not appear to be correlated with environmental variation is the way temperature seems to influence the reproductive cycle. Though temperature seems to be the principal exogenous factor influencing reproduction in E. cordatum [26,29,50], the modalities appear to vary between the different geographical basins, and thus among cryptic species.
If different triggering exogenous factors could be responsible for the discrepancy between the reproductive cycles of E. cordatum and E. mediterraneum, the same pattern might occur to a lesser extent between E. cordatum cryptic species, in such a way that the same environmental factor (seawater temperature variations) does not trigger the same step of the cycle (gametogenesis initiation in Wimereux and spawning in Vostok, Seto Inland Sea and Marseille).
As represented on Fig. 5, the greater resemblance of reproductive cycle parameters in the Marseille and Pacific populations fits with their genetic proximity whereas the environmental characteristics of the studied sites are highly different. Indeed, according to genetic analyses, the Mediterranean species (B1 + B2) and the Pacific one (P) form a super-clade opposed to the most ancient diverged Atlantic species (A) [47]. Nevertheless, it has been proposed that when species (especially congenerics) occur sympatrically, as it is the case for B1 and B2, their ecological differences can increase as a result of natural selection [6], and that they can respond to slightly different environmental signals, or somewhat differently to the same signal, thus avoiding wastage of gametes through interbreeding [11].
4.3 No obvious differences for the two sympatric cryptic species in the Mediterranean Sea (Fig. 5 node III)
Our results unambiguously show that the spawning season of B1 and B2 widely overlap, thus gametes of both species may potentially meet in the water column, but fine reproduction time segregation that could not be assessed in this study cannot be ruled out. For example, some sea urchin species (mainly diadematid) are known to follow a lunar spawning cycle superimposed on their annual cycle of gonad growth [11,80], the spawning of the different species occurring at different moon phases while the gonad maturation timing is synchronous. On the other hand, once diluted in seawater, sea urchin spermatozoids have limited longevity (about one hour [81], if not a few minutes [82]). The in situ dynamics of E. cordatum spawning remains largely unknown, and a few hours difference in spawning should be sufficient to prevent gametes from the two cryptic species from cross-fertilising. The question of the modalities preventing hybridization still remains unanswered, and further study of the reproductive barriers (pre- and post-zygotic) would be necessary to solve this paradox.
Conflict of interest statement
Nothing declared.
Acknowledgment
The authors gratefully acknowledge the MEDCHANGE project (http://www.Medchange.org), funded by the “Agence nationale pour la recherche (ANR)”, for allowing access to the temperature time series in Riou.
We are particularly grateful to I.Y. Dolmatov for providing unpublished temperature data for Vostok Bay. We thank M. Harmelin, E. Renard, and M. Verlaque, for valuable comments on the manuscript, and F. Zuberer and all the divers who spent many hours digging in the sand. We also thank M. Paul for correcting English.
This work was partly supported by the GBIRM project (MARBEF network of excellence [contract No. GOCE-CT-2003-505446]).