1 Introduction
Indiscriminate discharge of raw and partially treated industrial effluents into the aquatic system leads to deterioration of the environment. Among these pollutants the commonest toxins are organic salts of many heavy metals like, copper, zinc, lead, nickel, cadmium, silver and mercury. Some of the heavy metals are biologically important. In trace quantities heavy metals such as iron, copper, manganese, magnesium and zinc are essential for the metabolism of organisms [1]. Essential metals always function in combination with organic molecules, mostly with proteins either bound to metallo-proteins or loosely bound to metal–protein complexes [2]. If the threshold concentration of these metals increases in the environment they may interfere with the metabolic activity in organisms. Hence, the non-essential elements viz. mercury, cadmium, silver and lead are toxic to aquatic organisms even at very low concentrations.
Polluted water is one of the most significant sub lethal responses of fish [3]. Aquatic population can be affected by aquatic pollutants not only directly but their active retreat out of polluted areas can also result in disturbance of their migrations and distribution patterns [4]. Therefore a reduction of their normal area of habitat as well as their resources can occur [5]. From another point of view, the ability of fish to detect and avoid toxic substances is one of the forms of phenotypic adoption allowing them to survive in altered environment [6].
The rapid pace of industrialization and anthropogenic inputs have contaminated many ecosystems [7] especially the aquatic ecosystem, which receives a wide range of pollutants. Pollution of aquatic habitats seems to be an inevitable problem of universal nature and the intrusion of various pollutants into the aquatic environment affects the survival growth and reproduction of the biological organisms present in the environment. As fish being exclusively aquatic a number of potentially hazardous xenobiotics confront fish life in the sphere of their activities of which, a category of special interest is that of the heavy metals [8].
Heavy metals are extensively used in industries like electroplating, medicine, pesticide and battery manufacturing [9]. Recently, an increase in the concentration of heavy metals (Cd, Cu, Fe, Ni, Mn, Zn, Pb, and Hg) has been reported in water of Vasai Creek, Maharastra [10]. Heavy metal salts constitute a serious type of pollution in freshwater and being stale compounds they are not readily removed by oxidation. Precipitation of other processes can affect the activity in recipient animal [11].
Nickel is one of these heavy metals. The main sources of nickel come from hydrogenation of oil industry and paint factories, motor vehicle, aircraft industry, printing and in some cases the chemical industry. It is also used extensively in electroplating as nickel sulphate and nickel hydroxide is used in nickel–cadmium batteries [12]. In aquatic ecosystem, dissolved Ni concentrations are generally between 0.005 and 0.01 mg l−1 [13]. The toxicity of Ni to aquatic life has been shown to vary significantly with organism species, pH and water hardness [14]. Nickel toxicity is generally low [15] but elevated concentration can cause sub lethal effects. In fresh water fish, recorded Ni accumulation values ranged from 10 to 120 μg g−1 [16] and distribution of Ni varies significantly between the different tissues [17].
Chelating agents are the most versatile and effective antidotes for metal intoxication. These compounds are usually flexible molecules with two or more electronegative groups that form stable coordinate covalent bonds with the cationic metal atom. The body then excretes the complexes thus formed. The greater the numbers of these ligands, the more stable the metal chelator complex. The chelating ligands include functional groups such as –OH, –SH and –NH, which can donate electrons for coordination with the metal [18]. Chelating therapy is widely used in the management of metallic poisoning. Chelating therapy involves applying chelating agents into the blood stream for the purpose of eliminating from the body undesirable substances such as heavy metals, chemical toxins, mineral deposit and fatty plaques.
Calcium disodium complex – Ethylene diamine tetra acetic acid (CaNa2 EDTA) is an efficient chelator of many divalent and trivalent metals, especially for chelation of cadmium [19]. It is a stable water soluble metal chelate, mainly used in food industry, agriculture and horticulture as micronutrient, and calcium is present in a chelated form. It penetrates cell membranes and chelates extra cellular metal ions more effectively than intracellular ions [20]. The structure of CaNa2 EDTA is shown in Fig. 1.
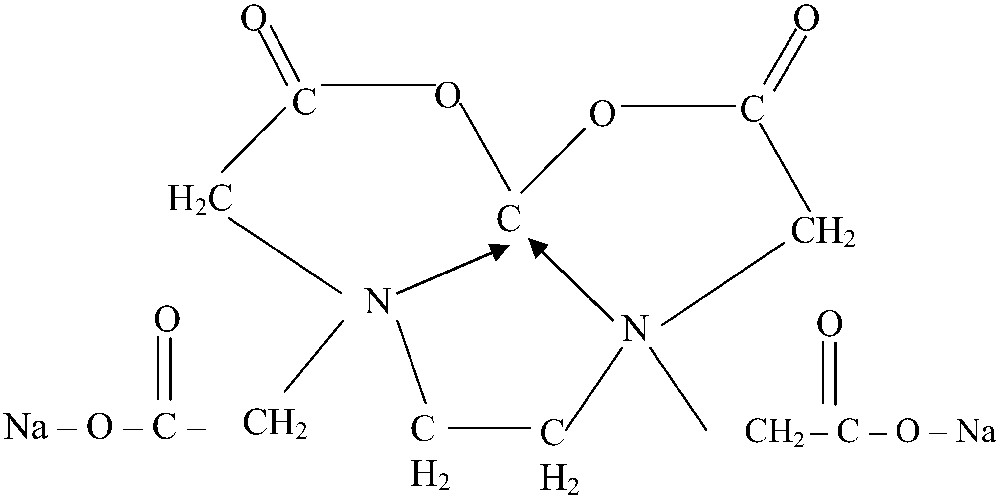
Structure of calcium di sodium-ethylene diamine tetra acetic acid.
Earlier researchers have concentrated mainly on adult fishes and not much of work has been done on fingerlings, which are more susceptible to environmental contamination. Also, the fingerlings have higher metabolic rates (per gram of body tissue) and therefore are able to take up metals via food and water more rapidly than adult fish. With this in view, in the present study an attempt has been made to investigate the toxic effects of nickel and the chelating influence of CaNa2 EDTA on the selected organs of freshwater, Cirrhinus mrigala (Ham.).
2 Materials and methods
2.1 Collection and maintenance of fish
Healthy specimens of Cirrhinus mrigala were collected from the farm located in Puther; Nagai, Q. Milleth District, Tamil Nadu, 15 km away from the University campus. They were stocked and maintained in the laboratory conditions (
2.2 Physical and chemical properties
Nickel is a silvery white ferromagnetic metallic element in Group VIII of periodic table. Nickel has the specific density of 8.90 g/cm3 and a melting point of 155 °C and boiling point of 28–37 °C. It is insoluble in water, soluble in dilute Nitric acid and Aquaregia and slightly soluble in Hydrochloric acid and Sulphuric acids. Nickel usually has an oxidation state of 2, but also occurs as relatively stable Tri- and Tetravalent ions [21]. Nickel forms complexes (Chelates) that are insoluble in water but soluble in organic solvents. These compounds are often very stable and play an important role in trace analysis.
2.3 Experimental protocol
Following a 24 hr renewal bioassay system for the analysis of sub lethal toxicity did the toxicant exposure. 3 groups of 20 fish each were exposed separately to nickel chloride (35 ppm; 10% of 96 h LC50) and nickel + CaNa2 EDTA (1:3 ratio, APHA, 1999) solution. The experimental medium was prepared by dissolving nickel chloride at 35 ppm in well water having dissolved oxygen 5.8 ppm, pH 7.4, water hardness 30.3 mg/l [22] and water temperature
2.4 Calculation of LC50
The LC50 value was obtained by the Probit analysis. Method was based on the observed percentage of test animals surviving at concentrations lethal to more than half and less than half of the test subjects [23]. The plotted dose response data reveal that the relationship of dose to response is non-linear. The Probit model [24] transforms the axis of the percentage of mortality to the Probit scale and the dose axis to the logarithm of the dose. By performing the transformation, the Probit model [24] transforms the dose-response curve to a straight line, which can be used for the calculation of the lethal concentration. The experimental data were analyzed by taking the Probit value of the percentage of mortality to the Y-axis and the logarithm value of the administered dose on the X-axis. A straight line was obtained on plotting the experimental data (Fig. 2). The LC50 can be interpolated from the curve by drawing a horizontal line from the 50% mortality point on the ordinate to the concentration-response curve and then drawing a vertical line from its point of intersection with the curve to the abscissa. The vertical line is then read off the graph. The percentages of mortality for different concentration and the obtained 96 hrs LC50 for nickel chloride are given in Table 1.
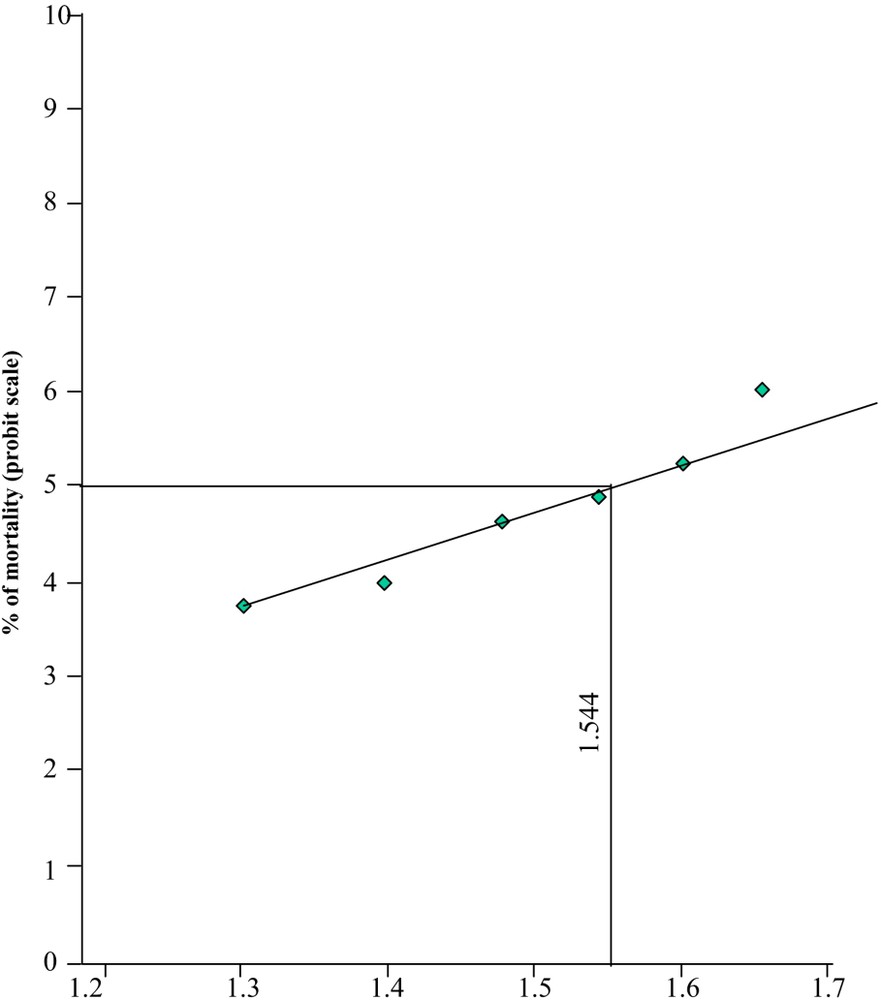
Probit of mortality of different concentration of nickel chloride.
Percentage of morality of Cirrhinus mrigala exposed to different concentrations of Nickel chloride.
Sl. no. | Concentration of NiCl2 (mg/l) | Log concentration | Mortality | % of mortality | Probit scale |
1. | 20 | 1.301 | 2 | 10 | 3.7182 |
2. | 25 | 1.397 | 3 | 15 | 3.9636 |
3. | 30 | 1.477 | 7 | 35 | 4.6147 |
4. | 35 | 1.544 | 9 | 45 | 4.8743 |
5. | 40 | 1.602 | 12 | 60 | 5.2533 |
6. | 45 | 1.653 | 17 | 85 | 6.0364 |
7. | 50 | 1.699 | 20 | 100 | 0.0000 |
2.5 Biochemical analysis
After the expiry of 28 days of exposure, 3 fishes each from the respective experimental as well as control groups were sacrificed. First pair of Gills (from both sides) liver, Kidneys, and pieces of muscle was excised from the experimental as well as control fish and were subject to various studies.
2.5.1 Estimation of total protein
Protein content in the selected tissue viz. gill, liver, kidney and muscle was estimated by following the method of Lowry et al. [25]. The tissue was isolated and 2% homogenate of each of the tissue was prepared in 10% TCA. The protein content is expressed in mg/g wet weight of the tissue.
2.5.2 Estimation of reduced glutathione
Reduced glutathione was determined according to the method of Moron et al. [26]. This method was based on the reaction with 5,5′-dithio-(bis)-2-nitrobenzoic acid (DTNB) to give a compound that absorbs at 412 nm. To 1 ml of the homogenate was added 1 ml of distilled water and 1 ml of 5% Trichloroacetic acid. The precipitate was removed by centrifugation. To an aliquot of the supernatant 2 ml of DTNB was added and 0.5 ml of phosphate buffer to a final volume of 3 ml. The absorbances was read at 412 nm in Spectronic – 20 (Bausch & Lomb) against a reagent blank. A series of standards (100–500 μg reduced glutathione) was also run in a similar manner. The amount of reduced glutathione was expressed as μg/mg protein for tissue.
2.5.3 Estimation of glutathione peroxidase (GPx)
The activity of Glutathione peroxidase was assayed by the method of Rotruck et al. [27]. With some modification a known amount of enzyme preparation was allowed to react with H2O2 in the presence of reduced glutathione. After a specified period of enzyme action, the remaining reduced glutathione content was measured by the method of Moron et al. [26].
To the mixture of 0.2 ml of phosphate buffer, 0.2 ml of EDTA, 0.1 ml of sodium azide and 0.2 ml of the enzyme preparation (tissue homogenate) were added and incubated at 37 °C for two minutes. Then 0.2 ml of reduced glutathione and 0.1 ml of H2O2 were added to the mixture and at 37 °C exactly for 10 minutes. The reaction was arrested by the addition of 0.5 ml of 10% TCA. Reduced glutathione content was estimated in the supernatant obtained after centrifugation. A blank was prepared similarly to which 0.2 ml of the enzyme was added after the incubation period. The activity of glutathione peroxidase was expressed as μg pf GPx utilized/min/mg protein.
2.5.4 Estimation of lipid peroxidation
The levels of lipid peroxidation in liver, gill, kidney and muscle tissue were estimated by the methods of Hogburg et al. [28]. In this method Malondialdehyde and other Thiobarbituric acid reactive substances (TBARS) were measured by their reactivity with Thiobarbituric acid (TBA) in acidic condition. A pink color was read at 535 nm. Tissue homogenate was prepared in Tris-HCl buffer (pH 7.5). 1.0 ml of the tissue homogenate was combined with 2.0 ml of TBA–TCA–HCl reagent and mixed thoroughly. The mixture was kept in a boiling water bath (60 °C) for 15 minutes. After cooling, the mixture was centrifuged at absorbance of the chromophore at 535 nm against the reagent blank. Values were expressed as mM/100 g tissue.
2.6 Statistical analysis
The data obtained from the biochemical analysis as well as accumulation studies were subjected to standard statistical processing based on random sampling of four different samples of experimental as well as control groups of each tissue at each sampling period. The observations were analyzed using Student ‘T’ test.
3 Results
3.1 Acute toxicity study
The acute toxicity values for the fish, Cirrhinus mrigala exposed to nickel were determined at different time intervals. The LC50 values for 24, 48, 72 and 96 hrs were estimated as 20, 25, 30, 35, 40, 45 and 50 ppm respectively (Table 1). The relationship between the median lethal concentrations (LC50) of nickel with the exposure periods is given in the toxicity curve (Fig. 2).
3.2 Acute bioassay of Cirrhinus mrigala exposed to nickel chloride
Acute toxicity tests are generally used to assess the potential hazards of chemical contamination in aquatic organisms. In fishes and other aquatic organisms, the effects of xenobiotics that have occurred within a short period of time are considered to be acute and relatively scarce. Data obtained in acute toxicity tests are generally used to compare the sensitivity of different species to potentialities of chemicals using LC50 values and thereby to decide safe environmental concentration. In the present study, the LC50 values for different exposure hours were studied. The percentage of mortality in different concentrations was also studied. It was observed that there is no mortality in the control as well as in the lowest concentrations below 96 hrs.
The logarithm of 50% lethal concentration is obtained by finding the value on the abscissa for straight line which assumes the probit value 5. The concentration resulting in 50% mortality and slope of the probit line were calculated for specific period of exposure as described by Finney (1971). The percent mortality data were subjected to probit values and plotted against log dose concentrations resulting in a straight line (Fig. 2). The LC50 value for 24, 48, 72 and 96 hrs of exposure periods were estimated as 20, 25, 30, 35, 40, 45, 50 ppm respectively (Table 1). Results thus clearly indicate the relationship between log concentrations and expected probit which is found to be positive and linear and the percentage mortality has been increased with an increase in toxicant concentrations and also with the increased exposure periods.
3.3 Biochemical changes
Exposure of Cirrhinus mrigala to sub lethal concentration of nickel chloride had induced various biochemical alterations. These alterations were manifested variously in different tissues. The prominent biochemical changes studied in the present work may be summarized as alterations in the total protein, reduced glutathione content along with lipid peroxidation content and glutathione peroxidase content of the selected tissues viz., gill, liver, kidney and muscle. In general, the alterations observed in the biochemical parameters of the tissues studied are listed. Histopathological observations are shown in Plates 1–3.

Histopathological studies. GILL. A. Control gill of Cirrhinus mrigala. CR – Cartilaginous Rod, GF – Gill Filament, PGA – Primary Gill Arch, PGL – Primary Gill Lamellae, SGL – Secondary Gill Lamellae. B. Gill of Cirrhinus mrigala exposed to sub lethal concentration of Nickel chloride. DCC – Disintegrated Chloride Cells, DPC – Disintegrated Pillar Cells, FSGL – Fusion of Secondary Gill Lamellae, HP – Hyperplasia, SPPC – Swollen and Pycontic Pillar Cells, V – Vacuoles. C. Gill of Cirrhinus mrigala exposed to sub lethal concentration of Nickel chloride and CaNa2 EDTA. NSL – Normal Secondary Lamellae, NPC – Normal Pillar Cells. Masquer
Histopathological studies. GILL. A. Control gill of Cirrhinus mrigala. CR – Cartilaginous Rod, GF – Gill Filament, PGA – Primary Gill Arch, PGL – Primary Gill Lamellae, SGL – Secondary Gill Lamellae. B. Gill of Cirrhinus mrigala exposed ... Lire la suite
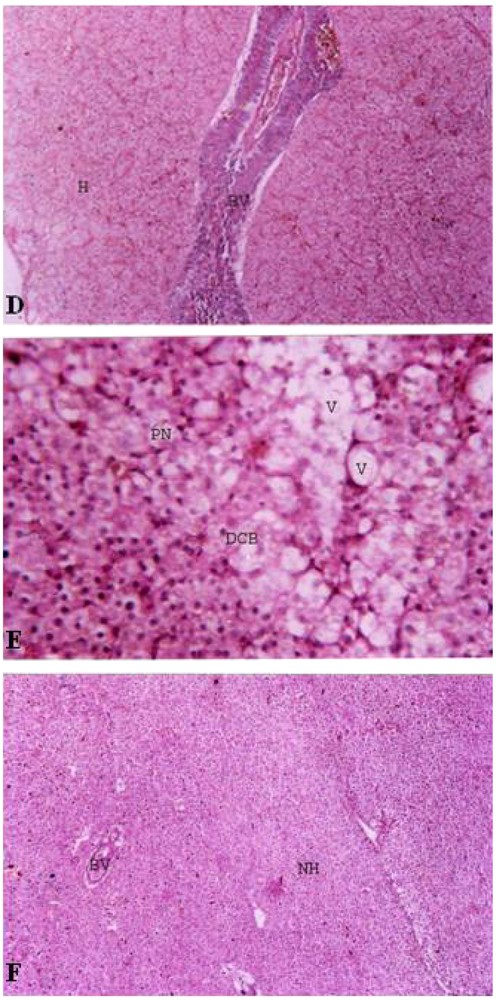
Histopathological studies. LIVER. D. Control Liver of Cirrhinus mrigala. BV – Blood Vessels, H – Hepatocytes. E. Liver of Cirrhinus mrigala exposed to sub lethal concentration of Nickel chloride. DCB – Disintegrated Cell Boundary, PN – Periphery Nucleus, V – Vacuoles. F. Liver of Cirrhinus mrigala exposed to sub lethal concentration of Nickel chloride and CaNa2 EDTA. BV – Blood Vessels, NH – Normal Hepatocytes.
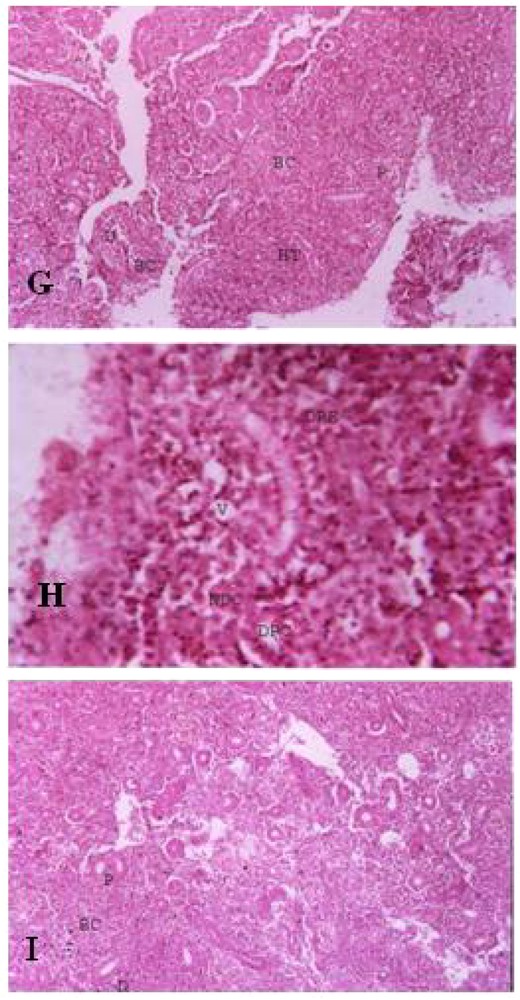
Histopathological studies. KIDNEY. G. Control Kidney of Cirrhinus mrigala. BC – Bowman's Capsule, D – Distal Convoluted Tubule, P – Proximal Convoluted Tubule, HT – Hemopoietic Tissue. H. Kidney of Cirrhinus mrigala exposed to sub lethal concentration of Nickel chloride. DPC – Disintegrated Proximal Convoluted Tubule, DRE – Desquamated Renal Epithelial Cells, NDC – Necrotic Distal Convoluted Tubule, V – Vacuoles. I. Kidney of Cirrhinus mrigala exposed to sub lethal concentration of Nickel chloride and CaNa2 EDTA. BC – Bowman's Capsule, D – Distal Convoluted Tubule, P – Proximal Convoluted Tubule. Masquer
Histopathological studies. KIDNEY. G. Control Kidney of Cirrhinus mrigala. BC – Bowman's Capsule, D – Distal Convoluted Tubule, P – Proximal Convoluted Tubule, HT – Hemopoietic Tissue. H. Kidney of Cirrhinus mrigala exposed to sub lethal concentration of ... Lire la suite
3.3.1 Impact of nickel chloride toxicity on total protein content
Sub lethal toxicity of nickel has a profound effect on the total protein of all the tissues studied. In the control group of Cirrhinus mrigala the level of protein content in gill, liver, kidney and muscle were
Level of protein content in different tissues of Cirrhinus mrigala exposed to Nickel chloride and Nickel chloride + EDTA.
Tissues | Control (Group I) | Nickel chloride (Group II) | Nickel chloride + EDTA (Group III) |
Gill | 14.15 ± 0.52 | 12.02 ± 0.52⁎⁎ | 13.15 ± 0.01⁎⁎⁎ |
Liver | 79.30 ± 2.61 | 58.32 ± 0.27⁎⁎⁎ | 77.87 ± 0.51⁎⁎⁎ |
Kidney | 40.55 ± 2.54 | 27.38 ± 0.26⁎⁎⁎ | 38.15 ± 0.24⁎⁎⁎ |
Muscle | 73.10 ± 1.31 | 52.15 ± 0.57⁎⁎⁎ | 70.32 ± 0.25 |
⁎⁎
⁎⁎⁎
Gill: At sub lethal concentration of nickel chloride-treated group the level of protein content was significantly (
Liver: At the sub lethal concentration of nickel chloride treated group the level of protein content in the liver was significantly (
Kidney: At the sub lethal concentration of nickel chloride-treated group the level of protein content was significantly (
Muscle: At the sub lethal concentration of nickel chloride treated group the level of protein content was significantly (
3.3.2 Impact of nickel chloride toxicity on reduced glutathione content
The GSH level in different tissues viz., gill, liver, kidney, and muscle of control and nickel-exposed fish and the antidote EDTA fish under sub lethal concentration of exposure is shown in Table 3. In control fish, the mean GSH level observed in different tissues were
Level of reduced glutathione content in different tissues of Cirrhinus mrigala exposed to Nickel chloride and Nickel chloride + EDTA.
Tissues | Control (Group I) | Nickel chloride (Group II) | Nickel chloride + EDTA (Group III) |
Gill | 1.28 ± 0.06 | 0.85 ± 0.02⁎ | 1.06 ± 0.02⁎⁎⁎ |
Liver | 2.20 ± 0.24 | 1.49 ± 0.18⁎ | 2.02 ± 0.15 |
Kidney | 1.90 ± 0.16 | 1.28 ± 0.05 | 1.79 ± 0.18⁎⁎⁎ |
Muscle | 1.70 ± 0.16 | 1.16 ± 0.02 | 1.49 ± 0.17 |
⁎
⁎⁎⁎
Gill: In the fish exposed to sub lethal concentration of nickel chloride, the GSH level was (
Liver: In the fish exposed to sub lethal concentration of nickel chloride, the GSH level was (
Kidney: In the fish exposed to sub lethal concentration of nickel, the GSH level was reduced to (
Muscle: In the fish exposed to sub lethal concentration of nickel, the GSH level was reduced to
3.3.3 Impact of nickel chloride toxicity on Glutathione peroxidase
The GPx level in different tissues viz., gill, liver, kidney and muscle of control, nickel chloride-treated and nickel chloride and EDTA-treated fish under sub lethal concentration of exposure in shown in Table 4. In control fish, the mean GPx level observed in different tissues were
Level of glutathione peroxidase content in different tissues of Cirrhinus mrigala exposed to Nickel chloride and Nickel chloride + EDTA.
Tissues | Control (Group I) | Nickel chloride (Group II) | Nickel chloride + EDTA (Group III) |
Gill | 0.84 ± 0.03 | 0.28 ± 0.02⁎ | 0.56 ± 0.18⁎ |
Liver | 1.40 ± 0.02 | 0.54 ± 0.02⁎ | 1.12 ± 0.01 |
Kidney | 1.15 ± 0.01 | 0.59 ± 0.02⁎ | 0.86 ± 0.026⁎⁎⁎ |
Muscle | 0.53 ± 0.02 | 0.30 ± 0.02⁎ | 0.58 ± 0.02⁎⁎ |
⁎
⁎⁎
⁎⁎⁎
Gill: When Cirrhinus mrigala was intoxicated with sub lethal concentration of nickel chloride, the GPx level was decreased to
Liver: When Cirrhinus mrigala was intoxicated with sub lethal concentration of nickel chloride, the GPx level was decreased to
Kidney: When Cirrhinus mrigala was intoxicated with sub lethal concentration of nickel chloride the GPx level was (
Muscle: When Cirrhinus mrigala was intoxicated with sub lethal concentration of nickel chloride the GPx level was (
3.3.4 Impact of nickel chloride toxicity on lipid peroxidation content
In the present experimental study the activity of lipid peroxidation has increased significantly after 28 days when Cirrhinus mrigala was treated with sub lethal concentration of nickel chloride. In the control fish of Cirrhinus mrigala the level of lipid peroxidation content in gill, liver, kidney and muscle were
Level of lipid peroxidation content in different tissues of Cirrhinus mrigala exposed to Nickel chloride and Nickel chloride + EDTA.
Tissues | Control (Group I) | Nickel chloride (Group II) | Nickel chloride + EDTA (Group III) |
Gill | 0.396 ± 0.03 | 0.786 ± 0.03⁎⁎⁎ | 0.384 ± 0.02⁎⁎⁎ |
Liver | 0.534 ± 0.03 | 0.813 ± 0.026⁎⁎⁎ | 0.530 ± 0.02⁎⁎ |
Kidney | 0.438 ± 0.06 | 0.783 ± 2.60⁎⁎⁎ | 0.429 ± 0.02⁎⁎⁎ |
Muscle | 0.423 ± 0.03 | 0.762 ± 0.05⁎ | 0.411 ± 0.02⁎⁎⁎ |
⁎
⁎⁎
⁎⁎⁎
Gill: The mean LPO level in the gill of fish treated with sub lethal dose of nickel chloride significantly (
Liver: The mean LPO level in the liver of fish treated with sub lethal dose of nickel chloride, significantly (
Kidney: The mean LPO level in the kidney of fish treated with sub lethal dose of nickel chloride, significantly (
Muscle: The mean LPO level in the kidney of fish treated with sub lethal dose of nickel chloride significantly (
4 Discussion
Despite progress made in environmental waste management, heavy metals still pose immense health hazards to humans and biota. Metals are present in very low concentrations in natural aquatic ecosystems [29], usually at the nanogram to microgram per liter level. Recently the occurrence of especially heavy metals in excess of natural loads in the environment has become an increasing concern [30] for aquatic ecosystem ‘health’ and is growing at an alarming rate.
The mechanisms of nickel-induced damage include the production of free radicals that alter mitochondrial activity and genetic information. The intake and excretion of heavy metals like nickel, cadmium, mercury and lead depend on the presence of antioxidants and thiols that aid methylation and metallothionein-binding. Antioxidants have specific roles in the mitigation of heavy metal toxicity. Certain metals when used in conjunction with standard chelating agents can improve the mobilization and excretion of heavy metals.
Acute toxicity is defined as the adverse effect occurring within a short time of administration of a harmful substance. It is useful to know the levels below which it may be considered safe for the occurrence of a particular toxicant in the environment [31].
During the present study the Cirrhinus mrigala exposed to different concentrations of nickel chloride for varying periods of time showed considerable variations in the degree of the toxicant as evidenced from the occurrence of mortality at varying rates (Table 4). It is evident that about 50% of mortality of Cirrhinus mrigala up to 96 hrs was caused by 35 ppm of nickel chloride exposure and is denoted as LC50/96 hrs doses.
The present result indicates that the percentage mortality of the fish for any fixed period increases with increasing concentration of the toxicant and for a particular concentration with an increase in exposure time indicating the regular mode of action of toxicant, which may be due to accumulation and subsequent magnification. Death of the fish may be due to the toxic effect of nickel chloride on the biochemical processes related to cellular metabolic pathways and to other inclusions [32].
A close and careful comparison of LC50 values of the present study with earlier reports can bring to light that LC50 values also depend upon the type of chemical used and the physical and physiological nature of the treated fish. From the present study it is concluded that the reaction and survival of a treated fish depends not only on the biological state of the fish and physio-chemical characteristics of test water but also on the effective concentration levels and time of exposure to nickel toxicants.
Thus knowledge of LC50 values obtained for a series of time intervals is highly useful for the final evaluation of the extent of pollution in an aquatic environment caused by heavy metals, especially nickel. The LC50 values obtained are also useful in establishing the limits and the levels of acceptability of toxic substances by the aquatic life.
Proteins are the building units of the body and are also the most abundant macromolecules in the cells constituting half of their dry weight [33]. They regulate and integrate various physiological and metabolic processes [34] in the body. Further, the protein content of the fish body mainly determines the quality and food value of its flesh. The maximum concentration of protein in the liver clearly shows the importance of liver in the body metabolism as well as its role as the major storehouse of various metabolites [35]. Further, a higher content of the protein in the liver may be attributed to the fact that liver synthesizes most of the plasma protein [36].
The reduction in the protein content after exposure to nickel chloride may be due to protein synthesis, which is considered the primary biochemical parameter for early indication of stress. This synthesizing is influenced by a large number of exogenous substances. They reduce the protein synthesizing capacity of the endoplasmic reticulum in the cell. In the present investigation, a sub lethal concentration of nickel-exposed fish, Cirrhinus mrigala showed a decrease in the protein content of gill, liver, kidney and muscle at the end of 28 days. This may be due to proteolysis, lack of protein biosynthesis or inhibition of translation. A significant decrease in protein content of gill, brain, intestine, liver, kidney and muscle was observed in nickel-treated fish [37]. A study by Jayakumar [38] on exposure to sub lethal concentration of cadmium reveals that the protein content of all the tissues analyzed showed a decreasing trend with variations in the percentage of decrease and a maximum decrease in the tissue of the liver and the gill.
The higher rate of GSH reduction recorded in the gill, liver, kidney and muscle of fish exposed to the sub lethal concentration of nickel chloride indicates primarily the higher amount of nickel chloride accumulated and metabolized by the liver. The gill shows minimum reduction of GSH level when compared to liver, kidney and muscle. The maximum reduction of reduced glutathione was recorded in all tissues after 28 days of exposure. Such reduction in GSH may result in the variability of alteration in membrane integrity, which is evidenced in the present histopathological observation.
Glutathione the most abundant non-protein thiol synthesized in the liver acts as a substrate for the enzyme glutathione peroxidase and also serves as a scavenger of different free radicals [39,40]. Kosower and Kosower [41] stated that the reduced glutathione (GSH) plays an important role in peroxidation (LPO) and provides a defense mechanism for tissues against the reactive oxygen species (ROS). The depletion of reduced glutathione (GSH) in the tissues was induced by heavy metals and it increases the metal toxicity in the tissues by increasing the free radical formation. The increase in free radicals such as H2O2 and HCHO through their metabolic reactions results in cell and tissue injury [42,43].
Activity of GSH-Px was significantly decreased in the liver and kidney of cadmium treated fish and this decrease was not prevented by concomitant selenium administration [43]. Glutathione (GSH) an important antioxidant and donor substrate for GSH-Px is also involved in the detoxification of heavy metals soon after they enter the cell. Jamall and Sporwis [44] have also reported the inhibition of heart cytosolic GSH-Px by Ca2+ in rats receiving selenium supplements. The therapeutic agents may protect against acute organ dysfunction and cellular injury thereby preventing the leakage of enzymes. The alteration in the permeability of the cell membrane and the inhibition of the ion-transporting system results in disturbances in the fluid movement. Thus the antioxidants administered prove their efficacy in inhibiting their efflux in the blood. Analysis of glutathione-related enzymes indicated lowering of the activity of Glutathione peroxidase and Glutathione reductase in the liver of rats. The reduced GPx activity is perhaps due to a lowered GSH content in liver and could be an adaptive mechanism attempting though unsuccessfully to maintain GSH level in liver. The decline of GPx activity leads to inefficient disposal of peroxides and reduced formation of oxidized glutathione [45]. By way of conclusion, the reduction in the enzyme glutathione peroxidase can be taken as a meaningful biochemical index of nickel chloride toxicity.
Sub chronic CdCl2 exposure for 30 days in fish Clarias batrachus showed decrease in Triiodothyronine, Thyroxine concentration and enhanced lipid peroxidation [46]. Cadmium treatment induced lipid peroxidation in the liver and in kidney in both Channa punctatus and Clarias batrachus [47]. Isamah et al. [48] have reported that the high level of iron in the fish species of the river Warri might impose stress on Malapterurus electricus, Tilapia mariae and Thysia consorgii due to an enhanced level of lipid peroxidation. The results of the present study suggest that lipid peroxidation may be a more appropriate early marker of oxidative stress in liver of this species.
The increased lipid peroxidation activity of liver indicated tissue injury and failure of antioxidant defense mechanisms which prevent the formation of excess free radicals [49]. Significant increase in the lipid peroxidation and decrease in the antioxidant enzyme activities have been reported in Cyprinus carpiomorpha exposed to copper sulphate and zinc sulphate in different concentrations for 24 hours [50]. Changes in the cellular defense system observed in the present study indicated that nickel chloride may pose more of a risk to the aquatic environment. The changes in the activities of some antioxidant enzymes and the level of lipid peroxidation are considered as indices of pollution of fish from Warri and Ethiopia rivers [48]. The results of the present investigation demonstrated that fish from polluted water are subjected to oxidative stress of multifarious dimensions.
5 Conclusion
It can be concluded from our present results that chelation therapy with CaNa2 EDTA proved significantly better results in restoring biochemical variables and pathological features immediately after a sub lethal exposure of nickel chloride in fish. Studies regarding their recovery could offer a solution to save fish living in an ecologically damaged habitat. The present investigation shows that the sub lethal levels can bring about changes in the structural and biological functions of the test animals. These changes indicate the healthy status of fish as well as the purity of the water in a particular environment. However, further work is needed before a final recommendation could be made. The findings suggest that a more detailed study of CaNa2 EDTA efficacy is warranted to conduct a randomized-controlled trial of chelation therapy in nickel-induced toxicity. Until such studies are performed, it would be premature to advocate chelation treatment on the basis of this study alone.
Vous devez vous connecter pour continuer.
S'authentifier