1 Introduction
Germination begins in dry seed with water imbibition followed by activation of biochemical systems leading into rupture of the covering layer and culminates with the emergence of radicle [1,2]. This process is inhibited by substrate salinity because of: (i) inadequate imbibition; (ii) ionic toxicity; (iii) interference with metabolism; (iv) destruction of enzymes; and (v) imbalance of growth regulators [3]. Seeds of halophytes are generally more tolerant to salinity and temperature stress during their storage in the soil and usually germinate during spring in temperate regions or after monsoon rains in sub-tropical areas when temperature and soil salinity is reduced [4]. At the time of germination, however, these seeds also become more sensitive to small perturbations in salinity, water, light and temperature regimes [3,5].
Ethylene, a known modulator of auxins, gibberellins, cytokonins, and abscisic acid during seed maturation [6,7], is released during seed germination but disagreements exist about its precise role in breaking dormancy and/or inducing germination [2,8]. It has been argued whether this gas production is a requirement for germination or it is just a consequence of reactions during germination as a major peak of ethylene evolution coincides with the completion of germination, but ethylene production is detectable very early during imbibition and before radicle emergence [6].
It is fairly well documented that ethylene removes primary dormancy [9–13], secondary dormancy [14], light induced dormancy [10], and that it reduces salinity, high temperature and osmotic stress on seed germination [15–24]. However, it has also been observed that an exogenous supply of ethylene enhances seed germination in some cases but may be ineffective or even inhibitory in other species [11].
Increasing salinization and pressure on existing fresh water resources has led to a focus on halophytes for the utilization of saline resources [25]. Seed germination, which is essential in establishing a good plant population, is drastically reduced by salinity and improving it through ethylene pretreatment under saline conditions may lead to favorable consequences. The present study was conducted on seeds of 22 halophyte species to ascertain whether the application of ethephon, an ethylene releasing compound, can alleviate the salinity effects on germination. This will provide insights into the involvement of ethylene in seed germination and may consequently lead to better management strategies for raising cash crop halophytes.
2 Materials and methods
Experiments were conducted using a number of halophytes (listed below) including salt secreting dicots (S. No. 1–5), salt secreting monocots (S. No. 6–9), stem succulents (S. No. 10–13) and leaf succulents (S. No. 14–22). These species were used to study the effect of ethylene (10 mM ethephon) on relieving innate and salinity induced seed dormancy. These seeds, which were collected from the coastal areas of Karachi, Pakistan [26], the salt marshes of Ohio County, USA and from the Great Basin Desert of Utah, USA, [27] were separated from the inflorescences and were surface sterilized using the fungicide Phygon (Hopkins Agricultural Chemical Co., Madison, Wis.). Depending on the salt tolerance of a particular species, the salinity levels used for germination are mentioned below along with the description of each species.
- (i) Cressa cretica L. (Convolvulaceae) is a dominant plant species in coastal and inland salt marsh communities of Pakistan, usually occurring in mono specific stands along the landward edge of marshes. Seeds were collected from salt flats at Karachi University campus and germinated in 100, 200 and 400 mM NaCl.
- (ii) Atriplex prostrata L. (Chenopodiaceae) is widely distributed in inland and coastal salt marshes of USA and Europe. Seeds were collected from a brine spill location in Athens County, Ohio, USA and germinated in 100, 200 and 300 mM NaCl.
- (iii) Atriplex stocksii Boiss. (Chenopodiaceae) is a short, robust perennial shrub which occurs in Mediterranean, South Asian and SE Asian regions. In Pakistan it is found in coastal as well as inland areas. Seeds were collected from salt flats at the Karachi University campus and germinated in 100, 200 and 300 mM NaCl.
- (iv) Atriplex rosea L. (Chenopodiaceae) is a weedy species of Eurasian origin which is also widespread in North America. Seeds were collected from a salt marsh at Faust, Utah, USA, 50 km south of the Great Salt Lake and germinated in 300, 600 and 900 mM NaCl.
- (v) Limonium stocksii (Boiss.) O. Ktze (Plumbaginaceae) is a perennial woody shrub distributed in coastal areas of Karachi, Pakistan, producing large numbers of seeds twice a year. Seeds were collected from a salt flat at the upper end of Manora creek near Hawks Bay, Karachi and germinated in 100, 200 and 300 mM NaCl.
- (vi) Triglochin maritima L. (Juncaginaceae) is a herbaceous perennial grass widely distributed in inland and coastal salt marshes of North America. Seeds were collected from a salt marsh located about 50 km south of Great Salt Lake at Faust, Utah and were germinated in 100, 200 and 400 mM NaCl.
- (vii) Aeluropus lagopoides (L.) Trin. Ex Thwarts (Poaceae) is a grass found landward on the salt marshes near Karachi. Seeds were collected from Manora creek near Sandspit, Karachi and germinated in 100, 200 and 400 mM NaCl.
- (viii) Urochondra setulosa (Trin.) C.E. Hubbard (Poaceae) is distributed in coastal regions of Sudan, Ethiopia, Somalia, Pakistan and Asia. Seeds were collected from a coastal population at Hawks Bay, Karachi and germinated in 300, 600 and 900 mM NaCl.
- (ix) Sporobolus ioclados (Nees × Trin) Nees (Poaceae) is a perennial grass widely distributed from sand dunes of the Arabian seacoast to the salt flats and saline deserts in northern Pakistan. Seeds were collected from salt flats at the Karachi University campus and germinated in 100, 200, and 400 mM NaCl.
- (x) Allenrolfia occidentalis (S. Wats) Kuntze (Chenopodiaceae) is a perennial species, which colonizes extremely saline habitats of temperate desert basins. Seeds were collected from a salt playa east of Goshen, NW Utah, and germinated in 200, 400 and 800 mM NaCl.
- (xi) Arthrocnemum macrostachyum Willd. (Chenopodiaceae) is a perennial shrub, commonly found in tropical salt marshes, and is frequently inundated with sea water. Seeds were collected from the Manora channel near Sandspit, Karachi and germinated in 200, 400 and 800 mM NaCl.
- (xii) Salicornia utahensis (Tiderstorm) Munz (Chenopodiaceae) is a perennial plant which occurs in inland salt playas of central Utah and can tolerate up to 5% salts. Seeds were collected from Goshen, Utah and germinated in 300, 600 and 900 mM NaCl.
- (xiii) Salicornia rubra Nels (Chenopodiaceae) is a salt tolerant annual species occurring in salt playas of the Great Basin desert of the western USA. Seeds were collected from salt flats situated at Goshen, Utah and germinated in 300, 600 and 900 mM NaCl.
- (xiv) Salsola imbricata Forssk. (Chenopodiaceae) is a xero-halophyte widely distributed in salt deserts and coastal areas of Pakistan. Seeds were collected from the Karachi University campus and germinated in 200, 400 and 800 mM NaCl.
- (xv) Kochia scoparia (L.) Schrader (Chenopodiaceae) is an annual herb commonly found in all counties of Utah. Seeds were collected from a salt marsh situated at Faust, about 50 km south of the Great Salt Lake, Utah and germinated in 300, 600 and 900 mM NaCl.
- (xvi) Halogeton glomeratus (Bieb.) C.A. Mey (Chenopodiaceae) is a succulent annual herb widely distributed in inland salt deserts of the western USA. Seeds were collected from a salt flat located 4 km north west of Faust, Utah and germinated in 300, 600 and 900 mM NaCl.
- (xvii) Salsola iberica Senen and Pau (Chenopodiaceae) is widely distributed in inland salt deserts of Utah and other similar areas in North America. Seeds were collected from a saline flat situated at Faust, 50 km south of Great Salt Lake, Utah and germinated in 300, 600 and 900 mM NaCl.
- (xviii) Sarcobatus vermiculatus (Hook.) Torr. (Chenopodiaceae) is a shrub found widely distributed in saline substrates of the Great Basin desert, Utah. Seeds were collected from salt flats near the southern end of the Great Salt Lake in Utah and germinated in 300, 600 and 900 mM NaCl.
- (xix) Suaeda moquinii (Torrey) Greene (Chenopodiaceae) is widely distributed in salt marshes of the western USA. Seeds were collected from a salt marsh situated at Faust, about 50 km south of Great Salt Lake, Utah and germinated in 300, 600 and 900 mM NaCl.
- (xx) Suaeda fruticosa (L.) Forssk. (Chenopodiaceae) is a perennial shrub found in highly saline soils distributed from southern Pakistan to the northern Himalayan valley of Chitral. Seeds were collected from salt flats at Karachi University campus and germinated in 100, 200 and 400 mM NaCl.
- (xxi) Ceratoides lanata (Pursh) J.T. Howell (Chenopodiaceae) is a winter browse for elk and deer, and is widely distributed in brackish water playas of northern Utah. Seeds were collected from a salt marsh situated some 50 km south of the Great Salt Lake at Faust, Utah and germinated in 300, 600 and 900 mM NaCl.
- (xxii) Zygophyllum simplex L. (Zygophyllaceae) is a leaf succulent ephemeral which is widely distributed in subtropical deserts of Africa and Asia. In Pakistan it is found from the coastal areas of Sindh and Balochistan to the plains of Punjab. Seeds were collected from salt flats at the Karachi University campus and germinated in 25, 75 and 125 mM NaCl.
Germination was carried out in 50 mm × 9 mm tight-fitting plastic Petri dishes with 5 mL of test solution. Each dish was placed in a 10 cm diameter plastic Petri dish as an added precaution against loss of water by evaporation. Four replicates of 25 seeds each were used for each treatment. Seeds were considered germinated at the emergence of a radicle. Germination was carried out in a growth chamber at an alternating temperature regime of 20–30 °C where the higher temperature coincided with the 12-h light period (Sylvania cool white fluorescent lamps 25 μmol m−2 s−1, 400–750 nm) and the lower temperature coincided with the 12-h dark period. Ethephon was added at 10 mmol/L and NaCl levels were as mentioned above with the species descriptions. The controls contained the solution without salinity and ethephon treatments. Percent germination was recorded every alternate day for 20 d.
Germination data were arcsine transformed before statistical analysis to ensure homogeneity of variance. Data were analyzed using the Student's t-test () [28].
3 Results
Germination was highly variable even in the absence of salinity, ranging from poor (e.g. about 30% in C. cretica and S. ioclados species) to almost 100% in certain other species, i.e. C. lanata, K. scoparia, A. occidentalis, S. vermiculatus, L. stocksii, A. lagopoides, U. setulosa, etc. (Figs. 1 to 5). Differences were also observed between species of the same genus. For instance, S. imbricata (90% germination) was better than S. iberica (59%); S. moquinii (100%) was better than S. fruticosa (69%), and A. rosea (89%) and A. prostrata (80%) were better than A. stocksii (42%).
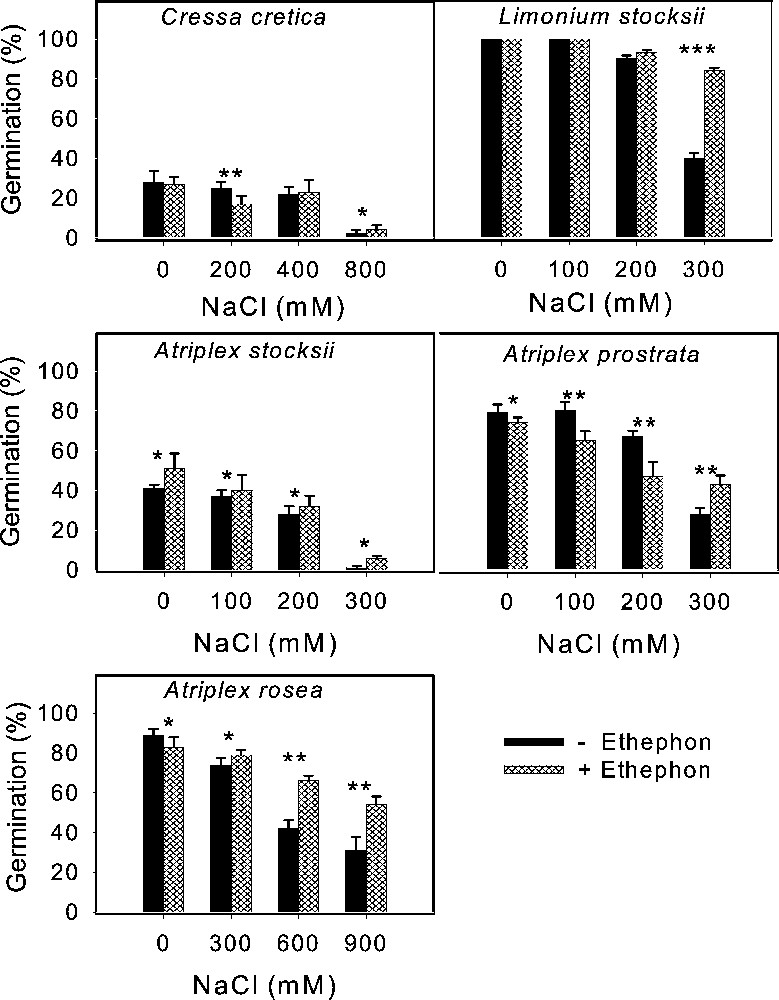
Effect of ethephon on the final germination (mean ± SE) of secreting dicot seeds treated with various concentrations of NaCl. Asterisks indicate significant differences for Student's t-tests (, , ).
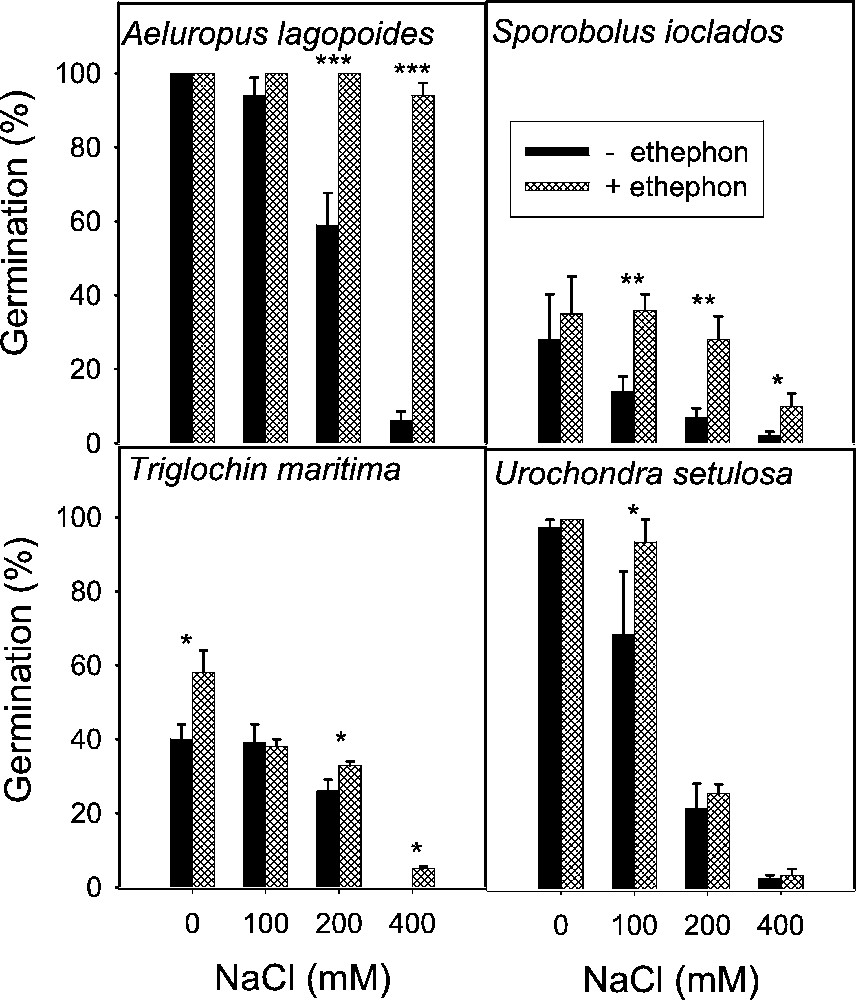
Effect of ethephon on the final germination (mean ± SE) of secreting monocot seeds treated with various concentrations of NaCl. Asterisks indicate significant differences for Student's t-tests (, , ).
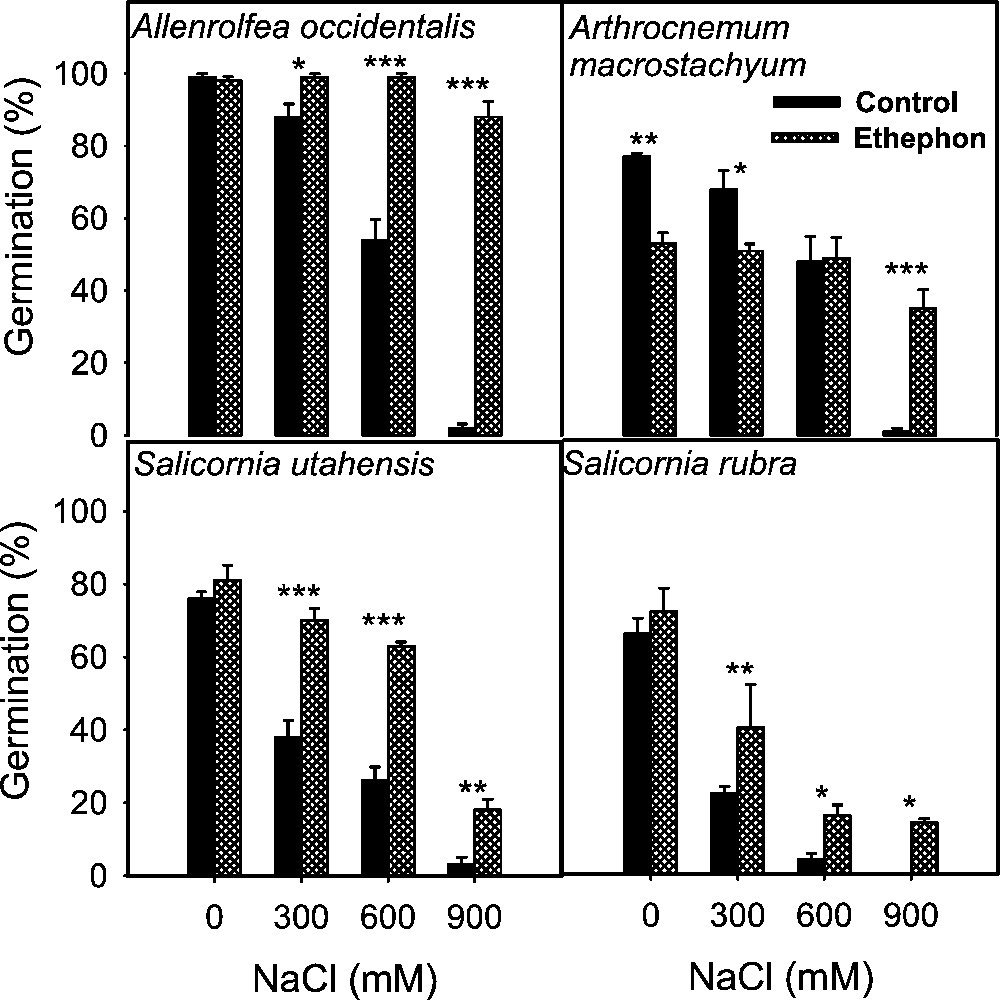
Effect of ethephon on the final germination (mean ± SE) of stem succulent halophyte seeds treated with various concentrations of NaCl. Asterisks indicate significant differences for Student's t-tests (, , ).
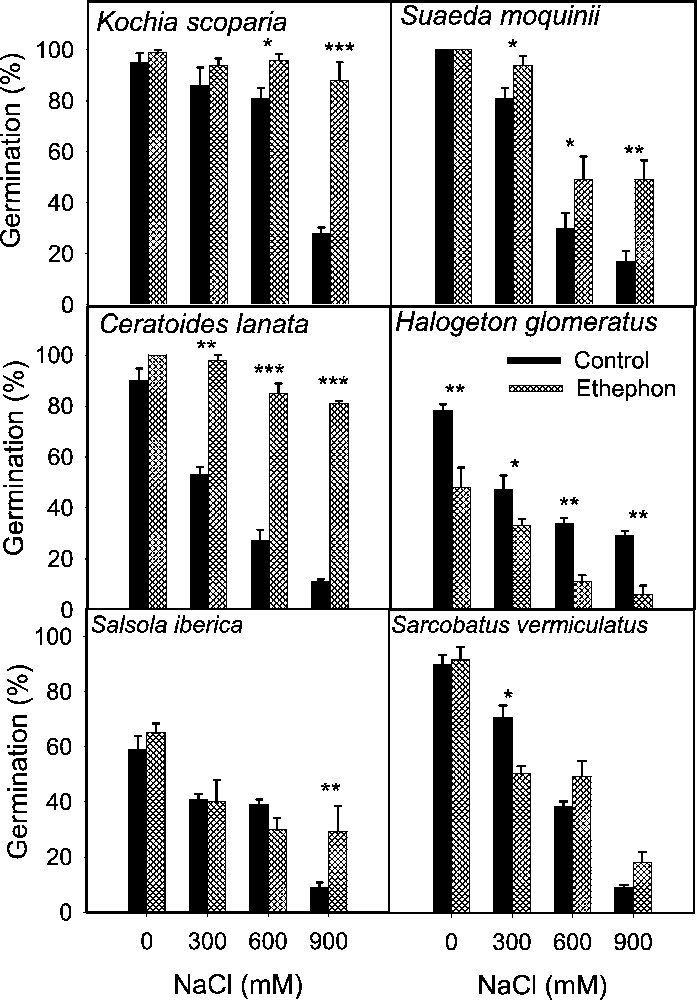
Effect of ethephon on the final germination (mean ± SE) of leaf succulent halophyte seeds treated with various concentrations of NaCl. Asterisks indicate significant differences for Student's t-tests (, , ).
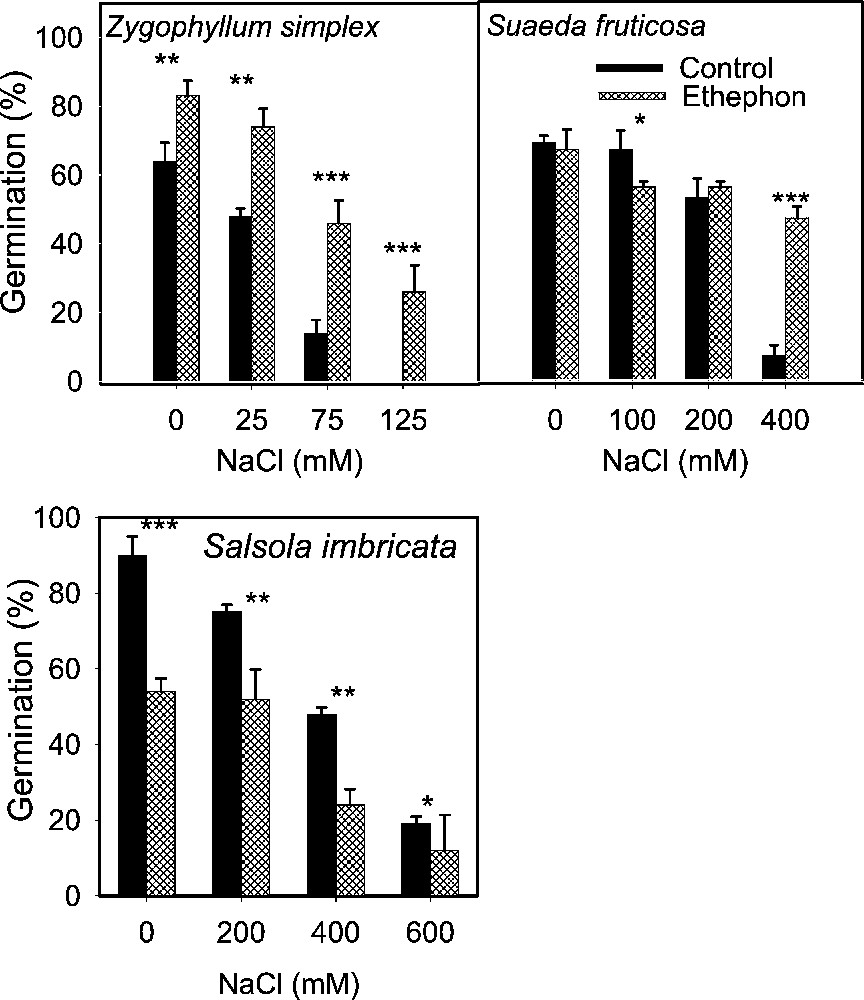
Effect of ethephon on the final germination (mean ± SE) of leaf succulent halophyte seeds treated with various concentrations of NaCl. Asterisks indicate significant differences for Student's t-tests (, , ).
Salinity reduced germination of all species tested irrespective of life form and there was a progressive decrease with increase in substrate salinity (Figs. 1 to 5). The lowest salinity used in each case brought little reduction in germination except in S. rubra, where it reduced more than 50% compared to its control (Fig. 3) whereas other species managed more than 50% germination, with some species reaching 80–100% (Figs. 1–5). A variable but more drastic effect was evident at higher salt levels. The reduction in germination was particularly prominent at the highest salinity level where less than 15% of seeds germinated in most of the species tested. Only A. prostrata, A. rosea and H. glomeratus showed somewhat better tolerance at this salinity level, exhibiting about 40% germination compared to their respective controls (Figs. 1 and 4). At the intermediate salt level most of the species performed well with more than 50% germination. At this salinity however, germination was inhibited by about 70% in U. setulosa, S. ioclados, S. utahensis, S. moquinii, C. lanata and Z. simplex and by more than 85% in L. stocksii and S. rubra, which were very badly affected (Figs. 1 to 5).
Application of ethylene failed to bring sizeable improvement in innate dormancy in most of the halophyte seeds studied except for T. maritima and Z. simplex (Figs. 2, 4). Ethylene in the growth medium was, however, generally responsible for alleviating salinity induced reduction in germination except in S. imbricata and H. glomeratus, where germination was inhibited substantially even in the presence of ethylene with or without salinity (Figs. 4 and 5). The ethylene effect became more prominent with increasing salinity, improving germination gradually at the lowest and intermediate salt levels, and was highly visible at the highest salinity in most cases (Figs. 4 and 5).
4 Discussion
Success of any plant population is dependent on the ability of its seeds to cope with environmental conditions and have enough individuals each season reaching maturity to ensure the continuity of the lineage. Seeds of halophytes as a group are no different from other plant groups except that they have to endure high soil salinity and high temperature stress during storage in the soil. Seeds of those halophytes which could survive these extremes without losing viability would be able to maintain their population [5].
Seed germination of halophytes reported here can be grouped under three categories based on salt tolerance under saline conditions. Group one contains species whose seeds can germinate in about 300 mM NaCl, including A. prostrata, A. stocksii, T. maritima and Z. simplex. A second group consisting of species with seeds germinating in salinity about 600 mM NaCl included L. stocksii, A. lagopoides, S. ioclados, S. fruticosa and U. setulosa. A third group with species where seeds could germinate in up to 1000 mM NaCl included A occidentalis, A. macrostachyum, A. rosea, C. lanata, C. cretica, H. glomeratus, K. scoparia, S. rubra, S. utahensis, S. iberica, S. imbricata, S. vermiculatus and S. moquinii. Seeds of most of the halophytic species survive high salinity and temperature stress while in the seed bank and germinate readily when temperature and salinity stress are removed [5]. This proved true in the present study with the exception of S. vermiculatus, H. glomeratus and S. iberica (Fig. 4). The results presented also support previous reports that species are less tolerant at seed germination than at subsequent growth stage.
For the sake of convenience and ease of presentation, the effect on germination (with the control set at 100%) is tabulated showing responses at the lowest, intermediate and highest salinity levels which are represented as S1, S2 and S3 irrespective of the salt concentration in each case (Table 1). Here, S1 can vary from 25 mM NaCl in the case of Z. simplex to 300 mM in K. scoparia, and H. glomeratus etc. Similarly S2 ranges from 75 to 600 mM and S3 from 125 to 900 mM. The salt sensitivity at germination is evident from here, as S3 brought the overall germination down to 13% of control (Table 1, salinity means). Only H. glomeratus and Atriplex species exhibited some resistance and managed to germinate around 40% of the seeds at the highest salinity level. Comparison between categories of halophytes on the basis of life forms showed salt secreting dicots having the best germination (66%) followed by leaf succulents (61%), salt secreting monocots (57%) and stem succulents (51%) (Table 1, species means).
Seed germination (percent of control) at the lowest (S1), intermediate (S2) and highest salinity (S3) levels irrespective of real salinity concentrations (without ethylene) in halophytes with various physiological adaptations. Abbreviations: Salt secreting dicots (SSD), salt secreting monocots (SSM), leaf succulents (LS), and stem succulents (SS).
Species | Life form | Salinity | Species mean | |||
0 | S1 | S2 | S3 | |||
Cressa cretica | SSD | 100 | 89 | 88 | 7 | 64 |
Atriplex prostrata | SSD | 100 | 100 | 68 | 40 | 77 |
Atriplex stocksii | SSD | 100 | 100 | 86 | 5 | 73 |
Atriplex rosea | SSD | 100 | 83 | 47 | 35 | 66 |
Limonium stocksii | SSD | 100 | 75 | 12 | 2 | 48 |
Mean | 100 | 89 | 60 | 18 | 66 | |
Triglochin maritima | SSM | 100 | 100 | 65 | 0 | 66 |
Aeluropus lagopoides | SSM | 100 | 94 | 59 | 6 | 65 |
Urochondra setulosa | SSM | 100 | 70 | 24 | 3 | 49 |
Sporobolous ioclados | SSM | 100 | 50 | 25 | 7 | 46 |
Mean | 100 | 78 | 43 | 4 | 57 | |
Ceratoides lanata | LS | 100 | 59 | 26 | 13 | 50 |
Kochia scoparia | LS | 100 | 90 | 85 | 29 | 76 |
Halogeton glomeratus | LS | 100 | 86 | 42 | 37 | 66 |
Salsola imbricata | LS | 100 | 83 | 53 | 21 | 64 |
Salsola iberica | LS | 100 | 69 | 66 | 15 | 63 |
Sarcobatus vermiculatus | LS | 100 | 78 | 42 | 10 | 58 |
Suaeda moquinii | LS | 100 | 81 | 30 | 17 | 57 |
Suaeda fruticosa | LS | 100 | 100 | 77 | 10 | 72 |
Zygophyllum simplex | LS | 100 | 60 | 30 | 0 | 48 |
Mean | 100 | 78 | 50 | 17 | 61 | |
Allenrolfea occidentalis | SS | 100 | 80 | 51 | 0 | 58 |
Arthrocnemum macrostachyum | SS | 100 | 86 | 61 | 14 | 65 |
Salicornia rubra | SS | 100 | 33 | 7 | 0 | 35 |
Salicornia utahensis | SS | 100 | 50 | 34 | 4 | 47 |
Mean | 100 | 62 | 38 | 4 | 51 | |
Composite mean | 100 | 78 | 49 | 13 |
Halophyte seeds germinate better under non-saline conditions, and the percent of seeds that germinate decreases with an increase in salinity. However, halophyte seeds may germinate at salinity concentrations much higher than the tolerance range of glycophytes [5]. Higher salinity in the medium causes osmotic and ionic effects on the species. It appears that faced with salinity, seeds of halophytes may maintain a high water potential to attain an appropriate level of hydration and protect the embryo from the deleterious effects of salt ions. Germination inhibition at higher salinity in halophytes could be due to failure of attaining minimum hydration required for germination or to protect enzymes from higher salinity, or may be due to inadequacy of seeds to manage oxidative stress created due to high water and/or ionic effect [29]. However, another important cause of germination inhibition may be the imbalance created in plant growth regulators in seeds during imbibition or later stages of activation of metabolic machinery. Seeds are known to produce inhibitors (phenolic inhibitors: [30]), particularly abscisic acid (ABA) [31]. Those seeds which failed to germinate under saline conditions due to the production of high levels of inhibitors and/or ABA could be induced to germinate with the application of growth promoters like gibberellins, ethylene and Brassinosteroids [6]. However, if the germination inhibition is caused by low availability of water or via interference with metabolism, perhaps the application of compatible solutes like proline and betaine could help by protecting enzymes from water and ionic stress [5]. Where seed germination under saline conditions is inhibited by inadequate management of oxidative stress, application of ascorbic acid may alleviate salinity effects [29].
A surge in ethylene production concomitant with, or shortly preceding, radicle emergence has been observed in a number of species [8,32–37]. The role of ethylene in the removal of dormancy and initiation of germination is debated [12]. Indeed, the very close temporal association between ethylene evolution and germination suggests a physiological link, but makes it difficult to establish the cause–effect sequence without the use of inhibitors of ethylene synthesis and perception [34,37]. Gianinetti et al. [8] showed that ethylene is not required for dormancy maintenance or release, nor is it necessary to initiate germination. Corresponding results were reported by Adkins and Ross [38] for intact oat caryopses, by Kepczynski and Karssen [18] for Amaranthus caudatus, by Machabee and Saini [32] for Chenopodium album, and by Petruzzelli et al. [33] for pea seeds. It appears that there is no general requirement for ethylene produced in the intact seeds to initiate dormancy breakage or germination [8,37].
The germination obtained in ethylene treated seeds when calculated as a percent of respective controls and presented in Table 1 above (i.e. representing salinity levels as S1, S2, S3 irrespective of salt concentration in each case) elaborated the observed beneficial effect of ethylene treatment on germination inhibited by salinity (Table 2, salinity means). It is evident from this table that ethylene treatment enhanced the germination three fold under S3 conditions compared to the control (302 against 103) and showed substantial improvement at S2 (182 against 103 in control). This increase was, however, nominal (124 against 103 in control) at S1 conditions. Comparison between categories of halophytes on the basis of life forms further substantiated the above findings. It was observed that promotion due to ethylene in salinity-inhibited germination was the highest in stem succulents (200, Table 2) having the lowest normal germination (51%, Table 1). A similar alleviating effect, but in decreasing order and magnitude, was observed in salt secreting monocots (178, Table 2 against 57% germination, Table 1), leaf succulents (165, Table 2 against 61% germination, Table 1) and salt-secreting dicots (158, Table 2 against 66% germination, Table 1). Seed germination was inhibited more when ethylene was included with salinity in the case of H. glomeratus and S. imbricata.
Seed germination (percent of control) at the lowest (S1), intermediate (S2) and highest salinity (S3) levels calculated as percentage of ethylene untreated seed at the same salinity level (with ethylene) in halophytes with various physiological adaptations. Abbreviations: salt secreting dicots (SSD), salt secreting monocots (SSM), leaf succulents (LS), and stem succulents (SS).
Species | Life form | Salinity | Species mean | |||
0 | S1 | S2 | S3 | |||
Cressa cretica | SSD | 86 | 92 | 105 | 180 | 116 |
Atriplex prostrata | SSD | 94 | 90 | 87 | 131 | 100 |
Atriplex stocksii | SSD | 126 | 108 | 108 | 180 | 130 |
Atriplex rosea | SSD | 93 | 107 | 157 | 174 | 132 |
Limonium stocksii | SSD | 100 | 133 | 350 | 600 | 296 |
Mean | 100 | 106 | 161 | 253 | 156 | |
Triglochin maritima | SSM | 145 | 100 | 127 | – | 124 |
Aeluropis lagopoides | SSM | 100 | 106 | 169 | 200 | 144 |
Urochondra setulosa | SSM | 102 | 136 | 118 | 133 | 122 |
Sporobolous ioclados | SSM | 125 | 257 | 400 | 500 | 321 |
Mean | 118 | 150 | 204 | 278 | 178 | |
Ceratoides lanata | LS | 108 | 181 | 362 | 683 | 334 |
Kochia scoparia | LS | 104 | 109 | 119 | 285 | 154 |
Halogeton glomeratus | LS | 61 | 49 | 33 | 21 | 41 |
Salsola imbricata | LS | 60 | 69 | 60 | 63 | 63 |
Salsola iberica | LS | 110 | 100 | 177 | 322 | 177 |
Sarcobatus vermiculatus | LS | 100 | 73 | 136 | 200 | 127 |
Suaeda moquinii | LS | 100 | 116 | 163 | 288 | 167 |
Suaeda fruticosa | LS | 97 | 84 | 105 | 271 | 139 |
Zygophyllum simplex | LS | 155 | 233 | 282 | – | 223 |
Mean | 99 | 113 | 160 | 299 | 168 | |
Allenrolfea occidentalis | SS | 100 | 125 | 186 | 0 | 137 |
Arthrocnemum macrostachyum | SS | 76 | 85 | 86 | 350 | 149 |
Salicornia rubra | SS | 109 | 182 | 400 | – | 230 |
Salicornia utahensis | SS | 106 | 184 | 242 | 600 | 283 |
Mean | 98 | 144 | 228 | 475 | 200 | |
Composite mean | 103 | 124 | 182 | 302 | – |
Ethylene is reported to alleviate salinity effects on seed germination of some species but not in others. Ethylene is naturally produced during the last step of germination helping the radicle to grow faster and complete germination, or, perhaps in some species, facilitating rupture of the seed coat. Seed germination requires higher turgor in the protruding radical to pierce through the barrier however, seeds of halophytes while germinating under high saline conditions will suffer due to the loss of turgor as well as higher ABA production. Application of ethylene may restore turgor and counter the effects of ABA produced due to high salinity.
If the germination is prevented by other than physiological reasons then ethylene may not have any role, or a higher concentration of growth promoting substance might synergistically inhibit germination in the presence of ethylene. The phytohormone ethylene promoted the germination of non-dormant and dormant seeds of several species [9,11]. Ethylene is also known to alleviate thermodormancy and salt enforced dormancy [4,20]. Ethylene and ABA are reported to modulate the physiological effects caused by salinity [39]. Ethylene production during germination is reported to increase under saline conditions in crop species [40–42]. An increase in ACC level and a decrease in ACC conversion to ethylene is also reported suggesting a reduction in ACC oxidase activity.
Recent studies revealed a close connection between ethylene and ABA signaling pathways during seed germination and plant root growth [42–45]. Ethylene negatively regulates ABA action during seed germination. Ruggiero et al. [46] also showed that impaired ABA biosynthesis resulted in higher ethylene accumulation, and reduced ABA accumulation during osmotic stress may cause increased sensitivity to ethylene in the loss-of-function mutation of NCED3 [46]. Therefore, the communication between ABA and ethylene hormone signaling may direct developmental decisions under adverse environmental conditions in Arabidopsis [47,48]. Lin et al. [49] showed that ABA2-overexpressing transgenic plants with elevated ABA levels exhibited seed germination delays and greater tolerance to salinity than wild type when grown on agar plates and/or in soil. However, the germination delay was abolished in transgenic plants showing ABA levels over 2-fold higher than that of wild type grown on 250 mM NaCl. The data suggest that there are distinct mechanisms underlying ABA-mediated inhibition of seed germination under diverse stress. The mechanisms causing an antagonistic effect on ABA inhibition of seed germination remain to be illustrated. One of the possibilities is the induction of ethylene production that is also stress inducible and has an antagonistic effect on ABA action [50]. Alternatively, we still cannot exclude the possible effect of osmotic and ion toxicity, which might reduce or override ABA inhibition of seed germination at high NaCl conditions.
5 Conclusion
Literature available so far on the subject indicates the paucity of information on the mechanism of halophyte seed germination under saline conditions. Halophyte seeds which could germinate under saline conditions approaching twice the salinity of seawater may offer clues to understand management of seed germination under such conditions and may lead to more efficient cultivation practices. Ethylene, ABA and GA are believed to operate in a cascade during seed germination. Therefore, it is important that the expression of these compounds controlling halophyte seed germination gains a better understanding. This is true not only for these growth regulators but other factors such as brassinosteroids and osmotic/oxidative stress.