1 Introduction
Plant form is ultimately defined by the structure of the walls encasing each cell. Leaves, flowers, stems, and roots all begin as a single cell or small groups of cells whose expansion is regulated differently from the cells in the surrounding tissues, leading to organ outgrowth. Furthermore, plants use regulated cell expansion to adapt their form to environmental conditions. For example, in the hypocotyl of a germinating seedling, light, temperature, and abiotic stresses strongly influence the extent and the direction of cell expansion. Uniform cell expansion in all directions is termed isotropic expansion, whereas cell expansion along a preferred axis, or in a preferred direction, is termed anisotropic expansion. The majority of plant cells expand anisotropically. Growth anisotropy is determined by the structure of the cell wall. Thus, plant growth and organ formation are intimately linked to the architecture and the metabolism of the cell wall. Here, we will review our current understanding of the factors that regulate cell wall deposition and growth anisotropy.
2 Anisotropic expansion at the cell level – Paul Green's hypothesis
The cell wall is composed of long, load-bearing cellulose microfibrils embedded in a viscoelastic matrix of pectins, hemicelluloses, and structural proteins. The mechanical forces operating on a given cell can be summarized as follows (Fig. 1):
- 1. Turgor pressure exerts a uniform, outward-pushing force;
- 2. Cellulose microfibrils in the cell wall exert a counter-acting, resistance force.
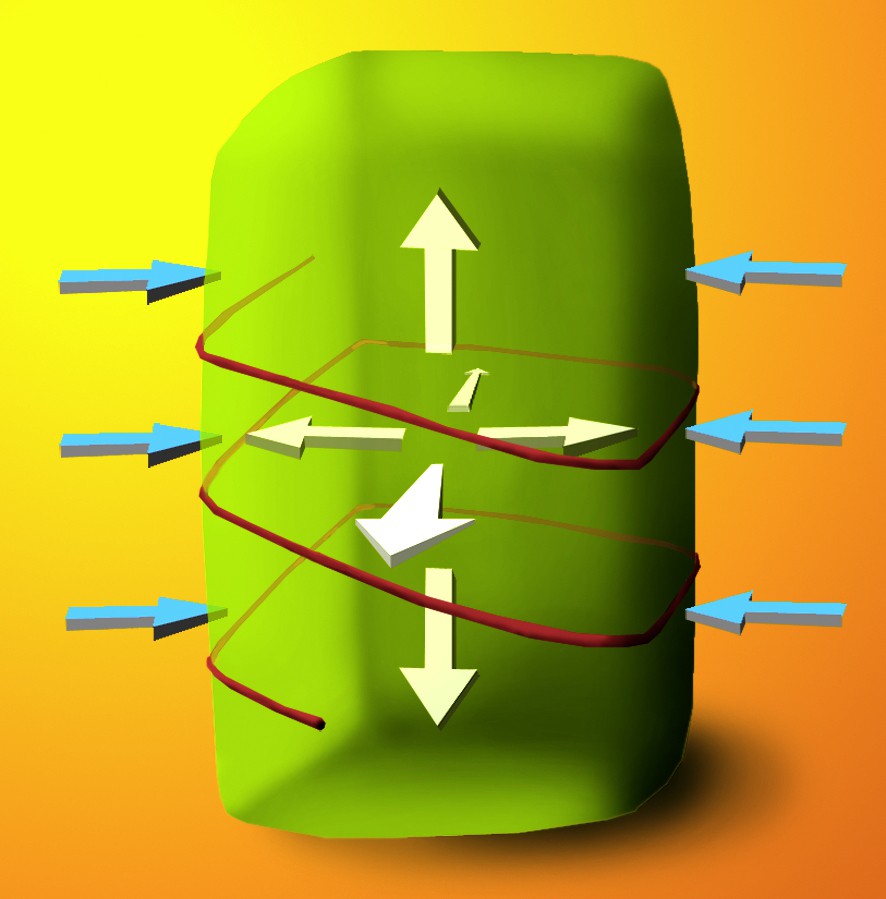
Schema illustrating Paul's Green Hypothesis. A plant cell is subjected to a constant, uniform force from turgor pressure (white arrows), which pushes out against the cell wall. The cell wall resists this force in an anisotropic manner (blue arrows), depending on the orientation of cellulose microfibrils (here, a single transverse microfibril is shown for simplicity). In this example, longitudinal cell expansion is favoured, rather than radial expansion.
It has been established that turgor pressure remains constant or decreases during plant growth [1]. Therefore, growth is not thought to be controlled by changes in turgor pressure, but rather by the yielding of the cell wall. The first observations of cellulose microfibrils in the plant cell wall [2,3] revealed that during certain stages of growth, the microfibrils are deposited parallel to one another, generating highly organized arrays. Secondly, it was noted that the orientation of these arrays is not constant throughout the wall [4].
These observations led Paul Green to hypothesize that anisotropic cell expansion is a result of the oriented deposition of cellulose microfibrils in the wall [5]. For example, consider a cell where cellulose microfibrils are deposited circumferentially (“as hoops on a barrel,” as Green described it in 1962 [6]): the interactions between the cellulose microfibrils and the other wall components will create a strong resistance to turgor pressure along the transverse axis of the cell, whereas the wall will be more extensible along the longitudinal axis (Fig. 1). This wall structure will thus favour longitudinal expansion. In contrast, if the cellulose is deposited in a longitudinally aligned array, the wall structure will be more conducive to transverse expansion, since the cellulose microfibrils may move apart in their viscoelastic matrix. The first direct measurements confirming Paul Green's hypothesis came from landmark studies of Probine and Preston [7,8], who demonstrated that the mechanical anisotropy of cell walls is due to the orientation of crystalline cellulose microfibrils.
3 Construction of the cell wall and the multi-net growth hypothesis
Paul Green's hypothesis to explain anisotropic cell expansion is closely linked to another, earlier hypothesis called the multi-net growth hypothesis [9], which states that:
- • The cell wall is an inert, passive structure, subject to mechanical strain from turgor pressure and active cell growth;
- • The cell wall grows only through secretion (apposition) of new wall material onto its inner surface;
- • Cellulose microfibrils are newly deposited strictly in a transverse orientation;
- • As the cell elongates, stress in the wall causes initially transverse cellulose microfibrils to undergo passive reorientation. The microfibrils first become randomly aligned at oblique angles, and eventually come to rest in a longitudinal orientation in the oldest wall layers.
It is now well-documented that the cell wall does indeed grow by apposition of new wall material to the inner surface, and that deeper wall layers are progressively remodelled [10]. However, a number of plant species exhibit helicoidal or crossed-polylamellate wall architecture in expanding cells [11,12], which is difficult to reconcile with the claim that cellulose microfibrils are always deposited transversely.
These observations led Roland et al. to propose the ordered fibril hypothesis [13]. In contrast to the multi-net growth hypothesis, the ordered fibril hypothesis states that wall lamella with varying microfibril orientations result from direct control of cellulose microfibril orientation at the time of deposition, with limited changes in orientation occurring during cell elongation. Thus far, experimental evidence from hypocotyls and stems of higher plants strongly supports the ordered fibril hypothesis, whereas the multi-net hypothesis in its original form appears to hold true for elongating root cells and the filamentous algae that first inspired it.
4 Going a step further … the microtubule-microfibril alignment hypothesis
As soon as it was established that cellulose microfibrils are deposited in precise orientations in the cell wall, interest turned to discovering by what mechanism their orientation could be regulated. In 1962, Paul Green postulated that putative “long cytoplasmic elements” that “can be disorganized by colchicine” specify the orientation of cellulose microfibrils in the cell wall [6]. One year later, Ledbetter and Porter discovered the existence of these “long cytoplasmic elements” in higher plants and named them cortical microtubules [14]. Strikingly, cortical microtubules showed parallel alignment with the most recently deposited cellulose microfibrils in the wall. These observations, confirmed and challenged by a profusion of studies throughout the following decades (reviewed in [15]), gave rise to the microtubule-microfibril alignment hypothesis.
In 1984, two researchers put forth the following criticism of the microtubule-microfibril alignment hypothesis [12]: “If, however, this mechanism was responsible for building a multi-turn helicoid, it would seem to involve an improbably large number of alternating depolymerizations and repolymerizations of the fields of microtubules…” However, within 10 years time, confocal imaging revealed reorientation of arrays of microtubules in Pisum sativum epidermal cells [16], and quantification of microtubule treadmilling offered an explanation for the mechanism of the rapid remodelling of the array [17]. Even more convincingly, parallel microtubule arrays adjacent to the outer epidermal cell wall were found to undergo global reorientations in a rotary pattern [18]. These consecutive changes in microtubule orientation may coincide with the observed changes in orientation of cellulose microfibrils from one cell wall layer to the next in helicoidal walls. In conclusion, the dynamics of cortical microtubules are perfectly compatible with their role as a guiding force for cellulose deposition.
Heath further developed the microtubule-microfibril alignment hypothesis by proposing that the enzymes synthesizing cellulose are motile [19]. He postulated that cortical microtubules guide their movement, thus accounting for the correlations in microtubule and microfibril alignment. Researchers recognized that if both cellulose synthase complexes and microtubules were dynamic, this could account for common discrepancies in the observed co-alignment of cellulose microfibrils and microtubules. Whenever microtubules and cellulose microfibrils were not found parallel, it was possible that the microtubules had reoriented faster than a new wall layer could be deposited.
The most convincing experiment in support of the microtubule-microfibril alignment hypothesis would test the orientation of cellulose microfibril deposition and the orientation of cortical microtubules in the same cell, in real-time. This experiment was accomplished in 2006 by Paredez et al. [20]. These researchers generated transgenic plants co-expressing CFP-tagged alpha-tubulin1 to label cortical microtubules, and YFP-tagged CESA6 to label the primary cell wall cellulose synthase complex. The results of this study beautifully supported the microtubule-microfibril alignment hypothesis, as cellulose synthase complexes were observed to migrate along cortical microtubules (Fig. 2) [20]. When the microtubules changed orientation, so did the trajectories of the complexes. Interestingly, depolymerization of microtubules using oryzalin did not alter the velocity of the complexes, but simply disorganized their trajectories, illustrating that microtubules are necessary for guidance, but not movement of cellulose synthase complexes.
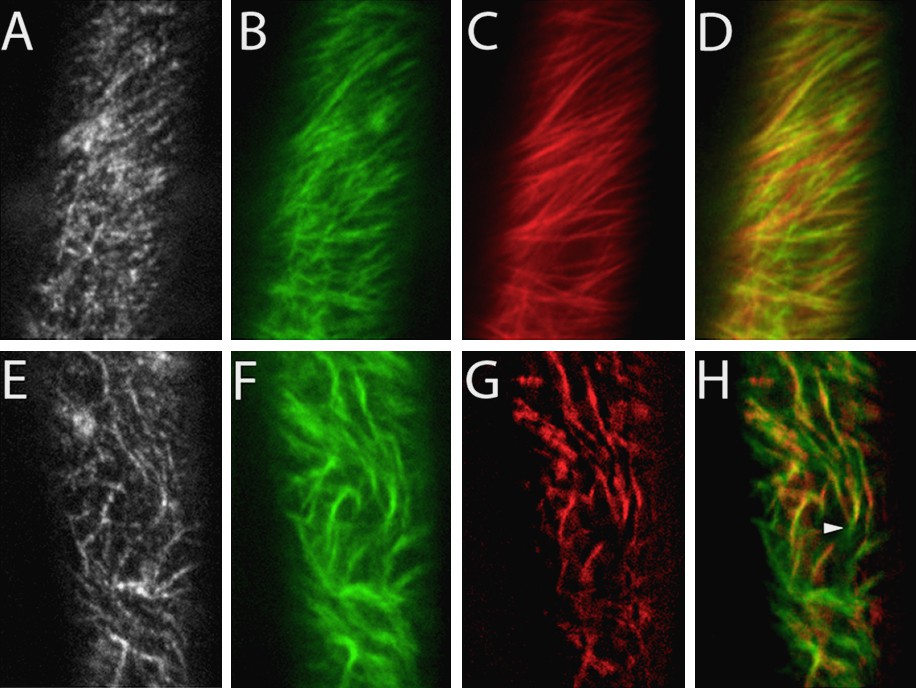
Cortical microtubules provide tracks for cellulose synthase complexes. (A–D): In control cells, cellulose synthase complexes labelled with YFP-CESA6 (A, B) have linear trajectories, as shown by averaging together the frames of the time series (B). Cortical microtubules labeled with CFP-TUA1 (C) form parallel arrays with the same orientation as the complex trajectories (overlay, D). (E–H): Following treatment with 10 μM oryzalin to partially depolymerize microtubules, the cellulose synthase complex trajectories (F) adopt the same curvature as the remaining microtubules (G), as shown in the overlay (H). Reproduced with permission from [20].
At present, Paul Green's hypothesis, Roelofsen and Houwinks’ multi-net growth hypothesis, Roland's ordered fibril hypothesis, and the microtubule-microfibril alignment hypothesis present a picture of the mechanisms operating during plant growth, from the starting point at cortical microtubule organization to the end result of cell wall anisotropy. However, it cannot be stressed enough that our model may only hold true for the outer face of the epidermal wall of Arabidopsis thaliana etiolated hypocotyls between 2- and 3-days-old. We can only speculate if the same hypothesis applies to other tissues and growth conditions. We still know very little about growth in the tissue context, and know even less about growth in a whole plant context. Considering the tools we have presently available, and the intriguing questions that remain to be answered, research on cellulose synthesis is in its prime.
5 Other tissues and alternative hypotheses
For those tissues where microtubules do not seem to guide the movement of cellulose synthase complexes (such as in tip-growing cells; [21]), a geometrical model has been proposed to explain helicoidal cell wall architecture [22]. The geometrical model states that the orientation of cellulose microfibrils is the result of a self-organizing process specified by simple parameters: the density of cellulose synthase complexes in the plasma membrane, the distance between individual microfibrils within a wall layer, the ratio of cellulose microfibrils to other wall components, and cell geometry. Interestingly, upon complete removal of microtubules by prolonged oryzalin treatment, YFP-CESA6 trajectories maintained an organization in parallel arrays with a oblique orientation to the long cell axis [23]. This suggests that this self-organizing process corresponds to a default mechanism, which may operate in certain cell types or under certain growth conditions, but which in most cell types is overridden by a microtubule-directed orientation mechanism.
6 Going yet another step further … how are cortical microtubules oriented?
Given the experimental evidence, we can now say with a measure of confidence that plant form originates from anisotropic cell expansion, which is at least partly regulated by the deposition of cellulose microfibrils in specific orientations in the cell wall. The orientation of cellulose deposition in diffuse-growing cells is specified by the organization of cortical microtubules adjacent to the plasma membrane. However, anyone who might be tempted to say we now understand plant growth should ask how cortical microtubules are oriented.
Interestingly, the first researchers to develop hypotheses about how cell wall anisotropy is generated considered the impact of stress directions [24,9]. After a hiatus of several years, the importance of mechanical factors in growth is beginning to be revealed. Just last year, Hamant et al. reported that cortical microtubules in the shoot apical meristem are oriented parallel to the predicted axis of maximal stress [25]. It will be interesting to test whether the rotary movements of microtubules on the outer surface of hypocotyl epidermis [18] reflect changes in maximum stress directions or are the result of independent processes [26,27].
7 Anisotropic expansion at the intracellular level
With all the attention given to the microtubule-microfibril alignment hypothesis, one may be tempted to think that cortical microtubules are the only factors regulating cellulose deposition. However, before microtubules can guide the movement of cellulose synthase complexes, the complexes must arrive in the plasma membrane. Thus, delivery of cellulose synthase complexes to their site of activity is a key regulatory step in cellulose synthesis. Study of the processes involved in the exocytosis/endocytosis of cellulose synthase complexes is yielding important new insights on the regulation of cellulose synthesis, and on intracellular trafficking in general.
Following their synthesis and assembly at the endoplasmic reticulum, cellulose synthase complexes traffic to the Golgi apparatus, as shown both by electron microscopy [28,29] and confocal microscopy [20]. In higher plants, the Golgi apparatus exists as multiple individual stacks that circulate through the cell at several micrometers per minute via the acto-myosin system [30]. Disruption of the movement of Golgi stacks using actin-depolymerizing drugs also disrupts the uniform distribution of cellulose synthase complexes in the plasma membrane [29], illustrating that the actin cytoskeleton is also important for ordered cellulose synthesis.
Studies of the trafficking of cellulose synthase complexes also revealed surprising new roles for cortical microtubules in the secretion and internalization of cellulose synthase complexes. Delivery of cellulose synthase complexes to the plasma membrane is preceded by the pause of a Golgi stack at the cell surface. Surprisingly, the pause sites are coincident with cortical microtubules [29]. These intriguing results suggest that there may be physical interactions between the complexes and cortical microtubules, even while the complexes are in the Golgi membrane. Furthermore, when the complexes are internalized, they remain physically tethered to cortical microtubules, again suggesting these two elements can interact [29,31]. Transient physical interactions between cortical microtubules and cellulose synthase complexes could represent the mechanism for microtubule-microfibril alignment (Fig. 3).
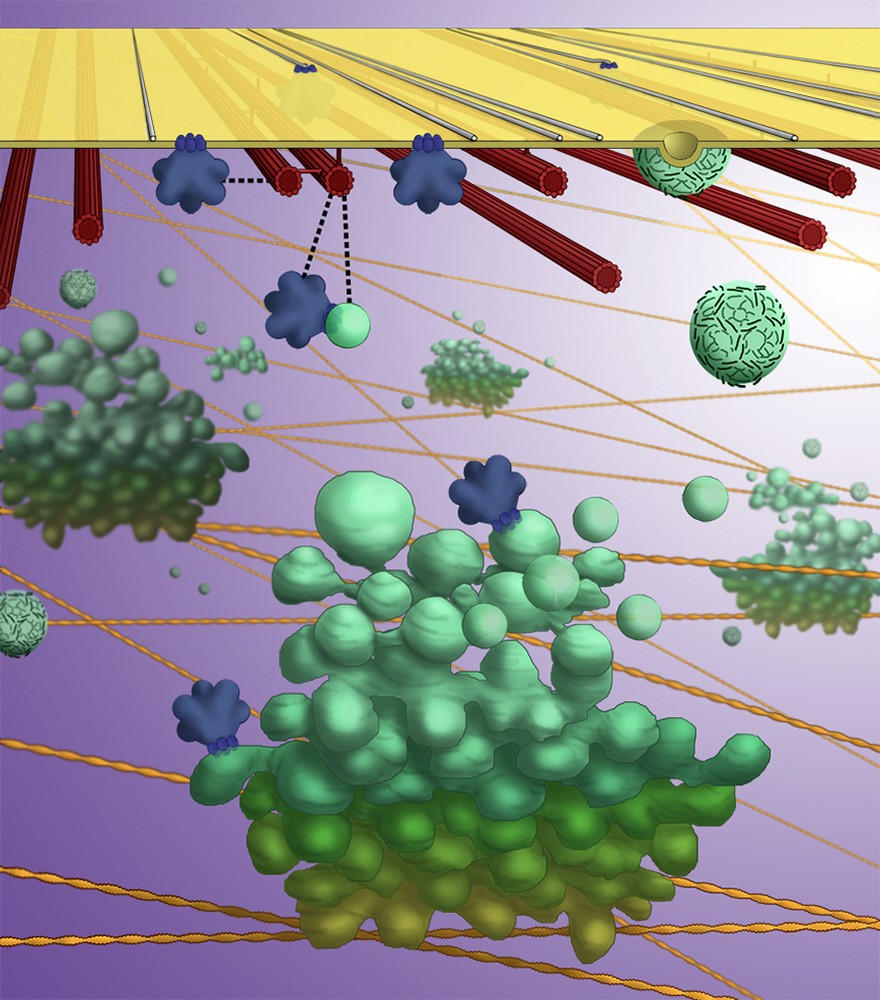
Artist's illustration of a current model for cellulose synthase complex trafficking. Golgi stacks (green) circulate through the cell, powered by myosin motors along actin filaments (yellow). Golgi stacks pause on cortical microtubules (red) for the secretion of cellulose synthase complexes (blue), which then may transiently interact with cortical microtubules as they migrate through the plasma membrane. Cellulose synthase complexes are internalized into microtubule-tethered vesicles, distinct from clathrin-coated vesicles (cyan).
8 Conclusions and perspectives
In conclusion, cell wall synthesis is a complex, dynamic process that depends on the communication of spatial information. Growth anisotropy may originate in the organization of the cytoskeleton and its interactions with organelles such as the Golgi apparatus. These molecular cues are finally translated into the ordered movement of cellulose synthase complexes at the plasma membrane, ultimately determining the orientation of cellulose microfibrils and the direction of growth.
The origins of growth anisotropy and the interactions between the cytoskeleton and organelles are the least understood steps in the process of wall construction. Yet, understanding these questions holds the most promise for increasing our understanding of a great number of biological processes that depend on these same elements. Indeed, the cytoskeleton and intracellular trafficking play essential roles in almost every process in the cell, from cell division, to differentiation and the establishment of polarity. Many exciting discoveries surely await researchers in this field.