1 Nutrient status of paddy under chromium stress
1.1 Introduction
Water pollution is one of the major hazards facing our environment today. Many industries discharge their untreated effluents into open land because of the high cost of treatment and water scarcity for dilution and inadequate sewage treatment facilities. The effective and profitable utilization of industrial effluent needs greater attention. Certain industrial effluents contain a mixture of nutrients, enhance the growth of crop plants but may also have toxic elements which inhibit the growth and yield of crops when they are used for irrigation [1].
The effluent discharging industries are distilleries, sugar mills, pulp and paper mills, detergent, chemical factories, textile dyeing industries, tanneries, electroplating, pharmaceuticals and dairy industries. Among them, tanneries occupy an important place and play a major role in polluting the environment by releasing a variety of pollutants as effluents. About 80% of the tanneries in India are engaged in chromium tanning processes and they use nearly 40,000 tonnes of basic chromium every year. Only a fraction of the Cr is absorbed in the tanning process and a major part of it is discharged through the effluent. It has been reported that these industries release 12,000–20,000 tonnes of Cr in effluent annually [2]. The concentration of Cr in tannery waste is about 20,000 ppm, about 1800 ppm in sludge and 200 ppm in composite effluent, and these are disposed of without any treatment. In addition, the physicochemical analysis of tannery effluent showed that the effluent normally contains 100 to 200 mg/l of chromium [3]. It has also been reported that a higher concentration (200 ppm) of chromium affected severely the physiological and biochemical characters of Albizzia lebbek [4]. The presence of an excess amount of chromium beyond the tolerable limits makes the land unsuitable for crop growth [5] and the fertility is also adversely affected by wastewater emanating from tanneries [6].
Chromium is the seventh most abundant available element on the Earth and 21st in the Earth's crust, with an average concentration of 100 mg/kg. Chromium compounds are commonly used as tanning agents, textile pigments and preservatives, antifouling paints, wood preservatives, metal finishing and in electroplating [7]. The toxic nature of chromium depends upon its valency state: Cr(VI) is more toxic than Cr(III), because of its solubility. Contamination of soil and ground water due to the use of chromium in various anthropomorphic activities has become a serious source of concern to plant and animal scientists over the past decade [8]. Previous studies have reported that chromium caused reduction in root length, shoot length and decreased the number of branches and induced biochemical changes in crops [9]. The toxic effects of chromium on Ocimum tenuiflorum leaves showed increased proline content and chromium significantly reduced the level of photosynthetic pigments, protein, cysteine, ascorbic acid and non protein thiol contents [10]. There are a few reports regarding the impact of chromium on germination and growth behaviour of various crops such as blackgram, soybean, paddy [11], varietal response of Soybean [12], mineral content variation of Amaranthus viridis [13] and changes in chromosome behaviour in blackgram [14] have been published. So, an attempt was made to learn the correlation between chromium accumulation and the uptake of nutrients in paddy (Oryza sativa L.). The removal of chromium from aqueous medium by using aquatic weeds and from polluted soil by terrestrial grasses and weeds were also carried out.
2 Materials and methods
2.1 Collection of seed materials
Paddy (Oryza sativa L.) seeds were obtained from the Regional Rice Research Station, Tamil Nadu Agricultural University, Aduthurai, Thanjavur District of Tamil Nadu.
2.2 Field experiments and chromium solution preparation
The field experiment was conducted with paddy seeds irrigated with different concentrations (control, 2.5, 5, 10, 25, 50, 75, 100 and 200 mg/l) of chromium prepared from the stock solution which was prepared by dissolving 2.95 g of potassium dichromate (K2Cr2O7) in 1000 ml of distilled water.
2.3 Irrigation schedule and sample collection
An equal volume (3 l) of chromium solution was irrigated two times in a week. The bore well water irrigated plot was treated as control. At the time of harvest (120 days), ten samples of paddy were harvested randomly and analysed for their root length, shoot length, total leaf area, fresh weight, dry weight, yield, nutrient content and chromium accumulation.
2.4 Morphological parameters
2.4.1 Root length and shoot length
The root length and shoot length of paddy were measured 120 days after sowing by using a centimetre scale and it is expressed in cm/plant.
2.4.2 Total leaf area
The length and breadth of each and every leaf was measured and the total leaf area was calculated by using the formula L × B × K and it is expressed in cm2/plant, where, L and B are the length and breadth, respectively, and K is the Kemp's constant, (for Monocot 0.9).
2.4.3 Total fresh weight and dry weight
The collected plant samples were washed thoroughly and separated into root and shoot. Their fresh weight was measured using an electronic balance. Then, the samples were kept in a hot air oven at 80 °C for 24 hours, and their dry weight measured using an electrical single pan balance.
2.4.4 Yield parameters
The yield parameters of 1000 grains weight and yield/ha were taken and recorded for both the treated and control plants.
2.5 Nutrient uptake
2.5.1 Nitrogen [15,16]
Two hundred milligrams of dried powdered plant sample was taken in a 100 ml Kjeldahl flask. Two hundred milligrams of salt mixture (potassium sulphate, cupric sulphate and selenium powder mixed in the ratio of 50:10:1) and 3 ml of concentrated sulphuric acid was added. After digestion, 10 ml of distilled water was added and cooled. The diluted sample was decanted into the micro-Kjeldahl distillation flask. To that, 10 ml of 40% sodium hydroxide was added and distilled. The distillate was collected in a conical flask containing 10 ml of 4% boric acid and three drops of mixed indicator (0.3 g bromocresol green and 0.2 g methyl red in 400 ml of 90% ethanol). This solution was titrated against 0.05 N HCl. Nitrogen content was calculated and recorded.
2.5.2 Phosphorus [15,17]
One gram of dried powdered plant material was digested with 10 ml of acid mixture (nitric acid 750 ml, sulphuric acid 150 ml and perchloric acid 300 ml). The digest was cooled and made up to 50 ml and filtered. One millilitre of the digest was mixed with 2 ml of 2 N nitric acid and diluted to 8 ml. One millilitre of molybdovanadate reagent (25 g of ammonium molybdate in 500 ml of water, 1.25 g of ammonium vanadate in 500 ml of 1 N nitric acid, both were mixed in equal volume) was added, shaken and the absorbance was measured after 20 min at 420 nm in UV-Spectrophotometer. A calibration curve was prepared using potassium dihydrogen phosphate as standard.
2.5.3 Potassium [18]
Dried and ground tissue of paddy (0.5 g) were digested in a 100 ml Kjeldahl flask using 10 ml of concentrated nitric acid, 0.5 ml of 60% perchloric acid and 0.5 ml of sulphuric acid. The inorganic residue was cooled and diluted with 15 ml of distilled water and filtered through Whatmann No. 42 filter paper. The filtrate was made up to 50 ml with distilled water. The filtrate was used for potassium estimation by Flame photometer and standards were prepared with potassium chloride.
2.5.4 Manganese, copper, zinc and iron [19]
One millilitre of sulphuric acid and 15 ml of double distilled water were added to a Kjeldahl flask containing 0.5 g of dried and powdered material was incubated overnight at 80 °C. After that, 5 ml of acid mixture (nitric acid and perchloric acid in the ratio of 3:1) was added and then digested. The digested material was cooled, made up to 50 ml and filtered through Whatmann No. 42 filter paper. The sample was aspirated to an Atomic Absorption Spectrophotometer with air/acetylene flame and the readings were taken for manganese (530 nm), copper (324.6 nm), zinc (214 nm) and iron (568 nm).
2.5.5 Accumulation of chromium [20]
Five hundred milligrams of the dried sample was digested in an acid mixture (HNO3 and HCl), filtered and brought up to 100 ml with distilled water. To 25 ml of the solution, 5 ml of diphenylcarbazide reagent was added and made to 50 ml. The solution was read in an Atomic Absorption Spectrophotometer at 540 nm. A standard curve was prepared by using a known amount of chromium.
2.5.6 Statistical analysis
Experimental results presented are the mean values of the experiments done in triplicates. The data was statistically analysed using a two-tailed T-test (SPSS 14.0) to compare paired means between different chromium treatments at 5% and 1% level of probability.
3 Phytoremediation of chromium by aquatic and terrestrial weeds
3.1 Introduction
Aquatic ecosystems have their own importance in keeping the environment clean. However, they get polluted by receiving huge volume of industrial effluent containing higher concentrations of heavy metals than that required by the biota. Detoxication and recovery of valuable metals are two important goals for the removal of heavy metals from aquatic ecosystem.
There are several methods for removing heavy metals from bodies of water. They can be ineffective and expensive. A simple, cost-effective, ecofriendly technology is needed today. Aquatic plants are considered to be simple, ecofriendly technology applicable for the removal of heavy metals from the aquatic medium [21].
In addition to water pollution, the problem of soil pollution contaminated by industrial effluents is getting a greater dimension. Changes in the soil properties were found to be inhibitory for the growth and yield attributes of crops [22]. So, it is necessary to implement some suitable biotechnological methods to reduce pollutants and heavy metals from contaminated soil. Phytoremediation is one of the best ecotechnologies in which the combined use of plants, soil amendments and agronomic practices are employed in order to remove the pollutants from the environment and to decrease their toxicity [23].
It is an efficient clean up technology for a variety of pollutants [24]. This technology makes use of the naturally occurring processes by which plants and their microbial rhizosphere flora degrade and sequester organic and inorganic pollutants. Organic and inorganic pollutants that can be phytoremediated include plant macronutrient, trace elements, non-essential elements and radioisotopes [25–27].
The uptake and accumulation of pollutants vary from plant to plant and also from species to species within a genus [28]. It is possible for detoxification of heavy metal from polluted areas by growing plants. The technology is based on the ability to absorb, sequester and or to transform a contaminant [29]. The cleaning of the contaminated site by the cultivation of selective hyper accumulator plants has emerged as a promising, cost-effective, simple technology to handle this problem. Hyper accumulators are plants commonly grown on metalliferrous soils and able to complete their life cycle without any symptoms of metal phytotoxicity [30]. Unfortunately, these plants usually produce less biomass than other plant species.
4 Materials and methods
4.1 Collection of aquatic plants
Aquatic plants such as Eichhornia crassipes, Pistia stratiotes and Salvinia natans were collected from the local freshwater pond. The plants of equal height and weight were selected and used for the removal of Cr from aquatic medium. Similarly, Cyperus rotundus, Ludwigia parviflora, Cyperus kylinga and Marsilea quadrifolia were collected and used for phytoremediation study.
4.2 Removal of chromium from aqueous medium
The collected water plants such as Eichhornia, Pistia and Salvinia were allowed to grow for 30 days in plastic containers containing 5 l of 100 mg/l chromium solution. The pH of the metal solution was adjusted to 6.0–6.5 which was considered suitable for the growth of aquatic plants. The experiment was conducted in the Botanical Garden of Botany Department, Annamalai University, Tamil Nadu. These aquatic plants were periodically harvested at 5, 10, 20 and 30 days of intervals. They were dried in a hot air oven and the dried plant materials were powdered and digested. The acid digested plant materials were used to estimate the accumulation of chromium as mentioned earlier [20].
4.3 Removal of chromium from polluted soil by plants
The naturally growing grasses and weeds viz., Cyperus rotundus, Cyperus kylinga, Marselia quadrifolia and Ludwigia parviflora were selected for this phytoremediation study, because of their tolerant capacity to grow in polluted sites. The field experiment was conducted in 1 m × 1 m sized plots. Ten seedlings of the same height were selected and allowed to grow in the polluted sites. Uniform irrigation (3 l of bore well water) was done two times in a week. After 30 days, these plants were harvested and dried in a hot air oven. These dried plant samples were powdered and digested to estimate the chromium accumulation.
5 Results and discussion
5.1 Growth and yield performance
Plant growth and development are essential processes of life and propagation of the species. They are continuous and mainly depend on external resources present in soil and air. Growth is chiefly expressed as a function of genotype and environment, which consists of both external and internal growth factors. The presence of Cr in the external environment leads to changes in the growth and development pattern of the plant [8].
The field experiment was conducted with paddy seeds irrigated with various concentrations of chromium solution up to 200 mg/l concentration. As the effluent contains normally 100–200 mg/l of Cr, it promoted our interest to know the impact of this higher concentration of Cr on growth and yield response of paddy. For this reason, these high concentrations of Cr have also been used in field experiments. The growth and yield parameters were recorded at the time of harvest and compared to control plants (Table 1 and Plate 1).
Growth and yield parameters of paddy (Oryza sativa L.) grown under various concentrations of chromium (120 days).
Chromium Concentration (mg/l) | Morphological growth parameters | Yield parameters | |||||||
Root length (cm/plant) | Shoot length (cm/plant) | Total leaf area (cm2/plant) | Fresh weight (g/plant) | Dry weight (g/plant) | 1000 seed weight (g) | Yield (kg/ha) | |||
Shoot | Root | Shoot | Root | ||||||
0 | 19.52 | 107.2 | 970.35 | 8.015 | 14.12 | 2.00 | 5.116 | 24.78 | 8035.160 |
2.5 | 18.16 | 105.5 | 885.28 | 7.685 | 13.16 | 1.986 | 5.016 | 23.16 | 7816.250 |
5 | 17.72 | 98.2 | 832.16 | 6.448 | 10.51 | 1.885 | 4.728 | 22.15 | 7132.168 |
10 | 17.05 | 90.6 | 820.00 | 5.236 | 9.68 | 1.600 | 4.416 | 19.17 | 6550.085 |
25 | 16.18 | 88.2 | 680.26 | 4.728 | 8.52 | 1.328 | 3.962 | 16.21 | 5018.368 |
50 | 14.60 | 75.8 | 538.22 | 4.436 | 7.14 | 1.168 | 3.665 | 15.42 | 4636.175 |
75 | 13.50 | 62.2 | 495.80 | 4.136 | 6.15 | 1.126 | 2.888 | 13.21 | 3210.162 |
100 | 12.50 | 54.6 | 376.20 | 3.168 | 5.08 | 1.011 | 2.162 | 11.21 | 2085.320 |
200 | 10.76 | 46.5 | 288.10 | 2.785 | 4.17 | 0.968 | 1.726 | 11.0 | 1735.355 |

Paddy (Oryza sativa L.) treated with different concentrations of chromium.
5.1.1 Root length and shoot length
The root length and shoot length of paddy decreased gradually with the increase of chromium concentrations (2.5, 5, 10, 25, 50, 75 and 100 mg/l). The root length of paddy varied from 19.52 to 10.76 cm/plant respectively between the control and treatments (200 mg/l). Similarly, the shoot length significantly varied from 107.2 to 46.5 cm/plant between control and the treatments (200 mg/l) respectively.
The depression in growth was more marked at the 200 mg/l concentration of chromium. The general response of decreased root growth due to chromium toxicity could be due to inhibition of root cell division or cell elongation or combination of both to the extension of cell cycle in the roots. It resulted in the limited exploration of the soil volume, uptake and translocation of nutrients and water, and induced mineral deficiency in plants [31]. It has been already reported that chromium causes retardation of growth up to 80% in both the root and shoot of tomato and brinjal [9]. The plant height reduced significantly in wheat cv. UP 2003 grown in sand with 0.5 M sodium dichromate [32]. The reduction in plant height might be mainly due to the reduced root growth and consequent less nutrients and water transport to the aerial parts of the plant.
5.1.2 Total leaf area
The total leaf area is a component of length, breadth and number of leaves, which reflects in the process of photosynthesis. The highest total leaf area was recorded in control plants and the lowest total leaf area was recorded in chromium-treated plants. The total leaf area gradually decreased with the increase in 200 mg/l chromium concentrations. The total leaf area (970.35 and 288.10 cm2/plant) varied significantly from control plant to 200 mg/l concentrations of chromium treated crop. A steady decrease in leaf area at higher concentrations can be attributed either to reduction in the number of cells in the leaves stunted by salinisation or reduction in cell size [33]. The reduced cell size and decreased intercellular spaces responsible for reduction in leaf area. The variation in size of areoles is also another factor which is responsible for reduction in total leaf area by chromium treatment [34,9].
5.1.3 Total fresh weight and dry weight
The fresh weight and dry weight of crops are mainly based on their growth performance of a particular crop. Growth directly influences the weight of plants. The highest dry weight (5.116 g/plant) in shoot of paddy was registered in control and the lowest dry weight (0.968 g/plant) was registered in 200 mg/l chromium treated paddy root. The total dry weight of root and shoot of paddy decreased gradually with the increase in chromium concentrations. It was mainly due to the inhibition of water uptake and enlargement of root cells. There was a distinct reduction in dry biomass at flowering stage in white mustard when Cr(VI) was given at the rates of 200 or 400 mg/kg soil [35,36]. The decreased biomass might also be due to disturbed carbohydrate and nitrogen metabolisms and reduction in protein synthesis or low photosynthetic reactions under excess Cr conditions [35,37].
5.1.4 Yield
Most physiological and biochemical processes are severely affected by chromium and, as a consequence, the yield of crop are equally affected [38]. The highest 1000 seed weight (24.78 g/plant) and yield (8035 kg/ha) were recorded in the control plants. The lowest seed weight (11.0 g/plant) and yield (1735 kg/ha) were recorded in the crop irrigated with 200 mg/l of chromium.
The increase in chromium supply from 2.5 to 200 mg/l, markedly decreased the grain weight and their economic yield (kg/ha). An almost 80% reduction in grain weight was observed at 200 mg/l chromium when compared to control plants. The depression in grain yield as a result of differential chromium supply was very high and ranged between 82% and 92% from 0.05 to 1 mM Cr [38]. Nitrogen, one of the primary essential nutrients involved as a constituent of biomolecules such as nucleic acids, nitrogen bases, coenzymes and proteins. Any deviation in nitrogen content would severely inhibit the growth and yield of plants [39].
5.2 Nutrient status
Cr is a toxic, non-essential element to plants. The toxic effects of Cr are primarily dependent on the metal speciation which determines its uptake, translocation and accumulation [5]. The changes in uptake of nutrients such as N, P, K, Mn, Cu, Zn and Fe and chromium accumulation in paddy root and shoot are presented in Figs. 1–7.
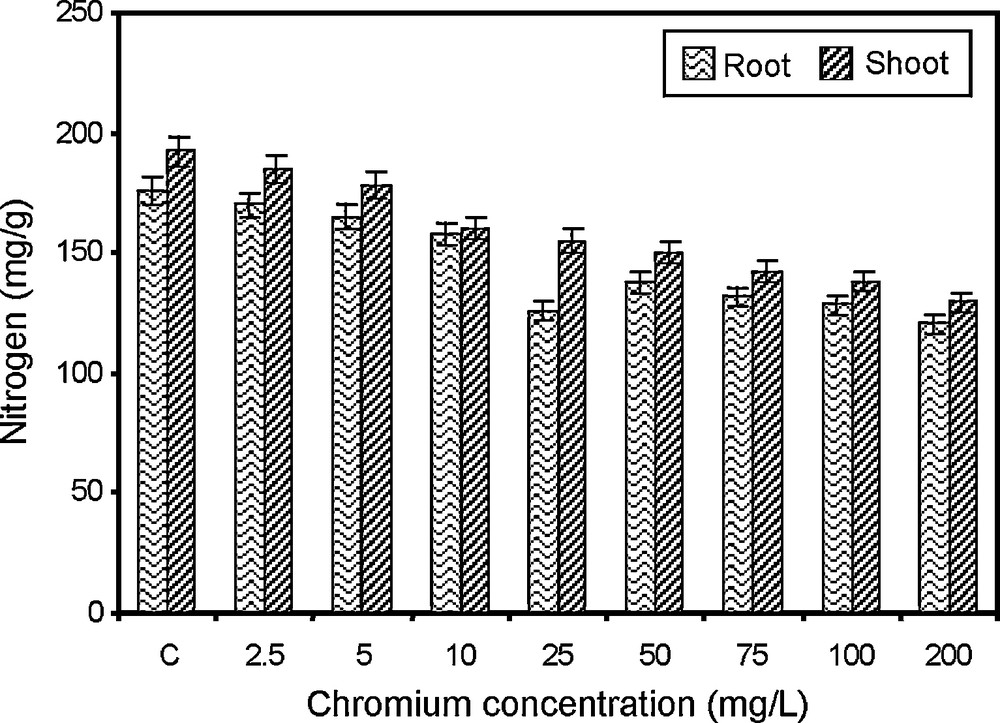
Changes in nitrogen content of paddy grown under various concentrations of chromium.

Changes in phosphorus content of paddy grown under various concentrations of chromium.

Changes in potassium content of paddy grown under various concentrations of chromium.

Changes in manganese content of paddy grown under various concentrations of chromium.

Changes in copper content of paddy grown under various concentrations of chromium.
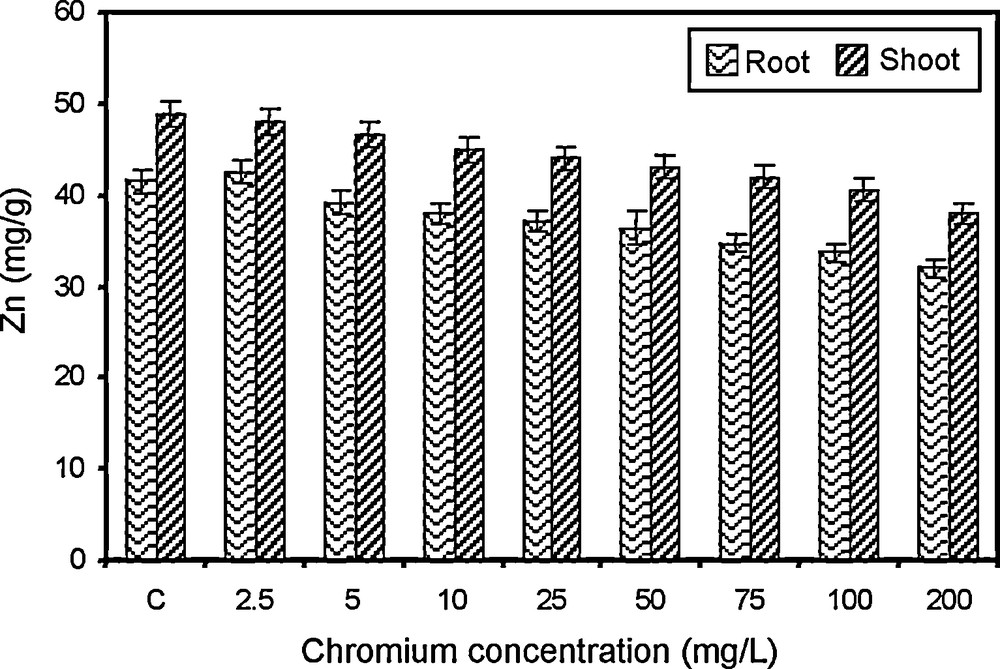
Changes in zinc content of paddy grown under various concentrations of chromium.
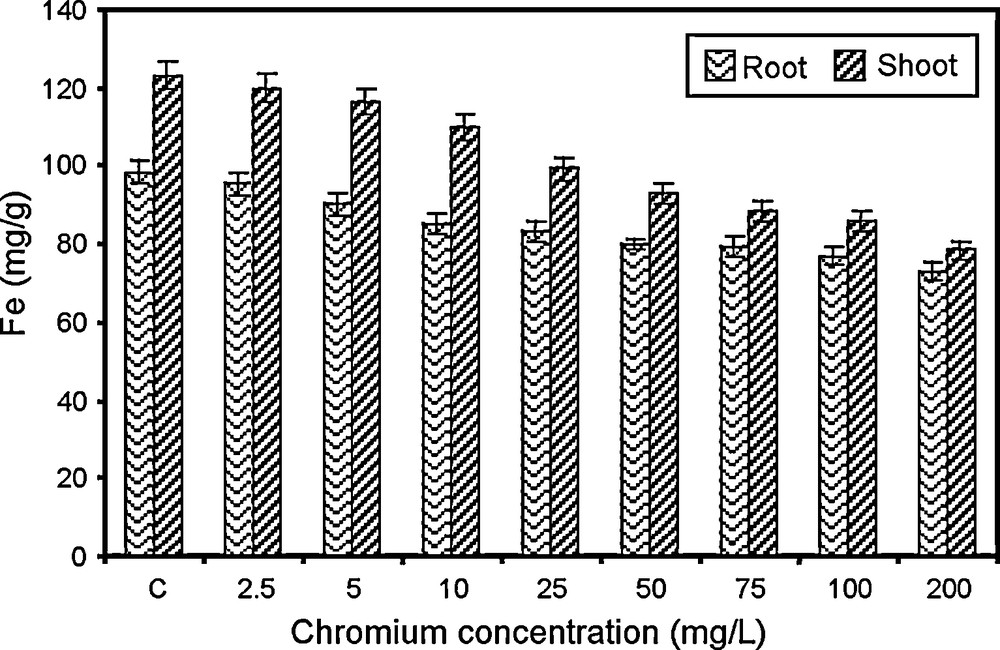
Changes in iron content of paddy grown under various concentrations of chromium.
The uptake of macronutrients (N, P, K) and micronutrients (Mn, Cu, Zn, Fe) gradually decreased with the increase in chromium concentrations. The reduction in N, P, K and other elements could be due to the reduced root growth and impaired penetration of the roots into the soil due to Cr toxicity. Cr had synergistic interactions in roots, but it showed an antagonistic effect in the stems and leaves. In the fruits, Cr treatment had no effect on Fe, Mn, Cu and Zn contents. The variation in uptake of mineral nutrition in tomatoes under Cr stress was reported [40]. Chromium in high concentration can displace the nutrients from physiologically important binding sites and thus might decrease the uptake and translocation of the essential elements [41]. In the present investigation, addition of Cr(VI) led to reduction in levels of nutrients in root and shoot of paddy. It indicates that Cr(VI) toxicity causes malfunction in the uptake of mineral nutrients which leads to deficiency in mineral nutrient.
5.2.1 Nitrogen
Nitrogen is an important constituent of protein and protoplasm and in many essential compounds. Nitrogen content in shoot and root showed a decreasing trend with increasing concentrations (2.5, 5, 10, 25, 50, 75 and 100 mg/l) of chromium (Fig. 1). The highest nitrogen content in shoot (192.3 mg/g dry wt.) and root (176.0 mg/g dry wt.) were recorded in control plants. Similarly, the lowest nitrogen content in shoot (130.0 mg/g dry wt.) and root (126.0 mg/g dry wt.) were registered in the 200 mg/l chromium-treated crop. Nitrogen content of paddy decreased significantly with the increase of chromium concentration. Similar inhibition of nitrogen metabolism due to excess of metals was also observed [42]. Chromium treatment affects nitrogen uptake and assimilation, which is evident from the decline in the concentration of nitrogen in wheat leaves. The uptake of nitrogen from soil was also inhibited by the presence of heavy metals [43].
5.2.2 Phosphorus
Phosphorus forms an important component of nuclear protein. A decrease in phosphorus content of paddy shoot and root were observed with increasing concentrations of chromium (Fig. 2). Phosphorus content of root and shoot of paddy varied significantly with chromium treatments. Similar differences in phosphorus accumulation due to metal stress were reported in soybean [44], greengram [45], groundnut [46] and Convolvulus arvensis [47]. The reduction in phosphorus content in the paddy may be due to: (i) blockage in mobilization of phosphorus from soil and root system to the aerial system due to chromium [48]; (ii) the toxic effects of chromium on root cells which impaired phosphorus absorption process, or to decrease availability of phosphorus with the addition of chromium in soil [49]; and (iii) chromium can competitively inhibit either the uptake or transport of phosphorus by the roots [50].
5.2.3 Potassium
Potassium is essential for the formation of sugars and starch absorbed by the root and is generally transported to the shoot and this transport seems to be controlled by shoot growth [51]. The content of potassium in root and shoot of paddy is gradually decreased with the increasing concentrations of chromium (Fig. 3). The same pattern of response of heavy metal in potassium uptake was also observed in Trifolium repens [52], in Pisum sativum [53], in corn [54] and in Oryza sativa [55]. Changes in the conducting tissue and blockage of the xylem elements may also contribute to a limited translocation of potassium from the root. It may be due to competition of chromium ions with potassium, which in turn exercised a regulatory control on potassium uptake [56].
5.2.4 Manganese
Manganese functions as an enzyme activator in several reactions of respiration and nitrogen metabolism. The chromium concentration markedly decreased the manganese content of root and shoot of paddy. The higher concentration of chromium (200 mg/l) treated paddy showed lower manganese content (Fig. 4). The chromium-induced manganese deficiencies are comparable to the works of Wallace and Abau-Zam Zam [57]. The decrease in manganese content may be due to the competition of chromium with manganese for transport sites in plasma lemma. The increase in chromium concentration might have displaced manganese from the absorption sites in cell walls [58,59].
5.2.5 Copper
Copper is required in very small quantity and acts as catalyst in oxidation-reduction reaction. The copper content of root and shoot of paddy decreased with a steady increase in chromium concentration (Fig. 5). The higher copper content was recorded in control sets while the lowest content was observed in paddy irrigated with 200 mg/l concentration of chromium. The results are in conformity with the reports of Gardea-Torresday et al. [47]. The decrease in copper content may be due, to some extent, to the close association of copper with nitrogen ligands and the close parallel relation in the movement of copper and nitrogen out of the leaves [60,61].
5.2.6 Zinc
Zinc functions as activator of certain enzymes and it is essential for the biosynthesis of plant auxin Indole-3-acetic acid. The zinc content of root and shoot of paddy decreased gradually with concomitant increase in chromium concentration (Fig. 6). The highest zinc content was recorded in control and the lowest content was recorded in 200 mg/l chromium treated crop. The paddy crop showed marked differences in their ability to absorb, translocate and accumulate zinc in various parts and this can be compared with the findings of Gardea-Torresday et al. [47]. The reduction in zinc content to due metal stress was also observed in raddish [62], blackgram [63] and Convolvulus arvensis [47]. The zinc concentration in the root was found to be lower when compared with the shoot. Hence, it is noteworthy that zinc uptake was affected by the chromium treatments but not the translocation of zinc within the plant [40]. Plants subjected to zinc deficiency were reported to undergo changes in diverse metabolism [64].
5.2.7 Iron
Iron is essential for the synthesis of chlorophyll and also plays an important role in respiratory mechanism. Iron content of root and shoot of paddy varied significantly with different concentrations of chromium. There was a gradual decline in iron content with increase in chromium concentrations. The iron content of root is found to be higher than shoot (Fig. 7). Chromium can substitute to some extent for iron in biological system [65]. These findings agreed with the results in white beans [65], in tomato [66]. The reduction in iron content may be due to: (i) the increasing concentration of chromium leading to iron deficiency, because of the competitive occupation of active sites of iron [67]: (ii) the chromium may be competing with iron, which leads to the lowered translocation of iron from the roots to the shoots that resulted in chlorosis [68], and (iii) the reduction in the uptake of iron could be mainly due to the chemical similarity of these ions in solution.
5.2.8 Accumulation of chromium
Chromium uptake by plants is mainly nonspecific probably as a result of uptake of essential nutrients and water. The chromium accumulation in the paddy root and shoot varied significantly due to chromium treatment. The accumulation of chromium increased positively with the concentrations (2.5 to 200 mg/l). The accumulation of chromium in root and shoot of paddy are gradually increased with the increase in chromium concentrations (Fig. 8). It has been reported that translocation of chromium from roots to tops was inhibited in the presence of toxic levels of the metals [33]. Thus, major portion of the element remained in the roots and only a small part was translocated to tops due to metal stress. It has been already reported that Cr distribution in crops had a stable character which did not depend on soil properties and concentration of this element. The maximum quantity of element contaminant was always found in roots and a minimum in the vegetative and reproductive organs [69] reported that Cr was poorly taken up into the aerial tissues, but was held predominantly in the root. The reason of the high accumulation in roots of the plants could be because chromium is immobilized in the vacuoles is less toxic, which may be a natural toxicity response of the plant [70].
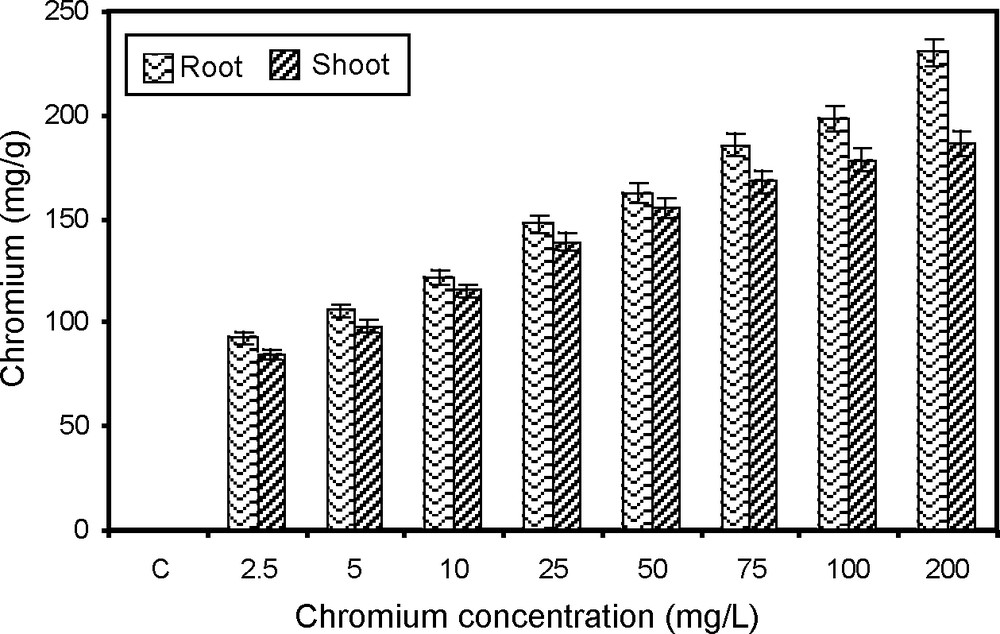
Chromium accumulation of paddy grown under various concentrations of chromium.
In this investigation, addition of Cr (VI) led to the reduction in the levels of nutrients in root and shoot of paddy. It indicates that the Cr (VI) toxicity causes malfunction in the uptake of mineral nutrient, which leads to mineral nutrition deficiency [71].
5.3 Phytoremediation of chromium
5.3.1 Removal of chromium from polluted water by aquatic weeds
The data for the uptake and accumulation of chromium using water plants are presented in Fig. 9. In this experiment, certain aquatic macrophytes such as Eichhornia crassipes, Pistia stratiotes and Salvinia natans were employed in the biological treatment. They were allowed to grow in chromium-contaminated water (100 mg/l) for 30 days. As a result, the roots of Eichhornia accumulated 75% chromium than in the leaves (60%). Similarly, lower chromium content was recorded in Pistia root (65%) and leaves (58%) than the Eichhornia. Salvinia accumulate 60% chromium and 55% in root and leaves from the chromium contaminated water. The accumulation of chromium increased with the number of days exposed. Among the plants, Eichhornia accumulated more chromium than the other plants tested. Water hyacinth (Eichhornia crassipes) is a flowering macrophyte whose appetite for uptake of nutrients and explosive growth rate has been put to use in cleaning up municipal and agricultural wastewater. The macrophytic species such as Potamogeton crisspus, Trapa bispinosa, Vallisneria spirallis Eichhornia crassipes, Azolla pinnata and Lemna minor [72,73] have been already reported in the removal of chromium from the aquatic medium.
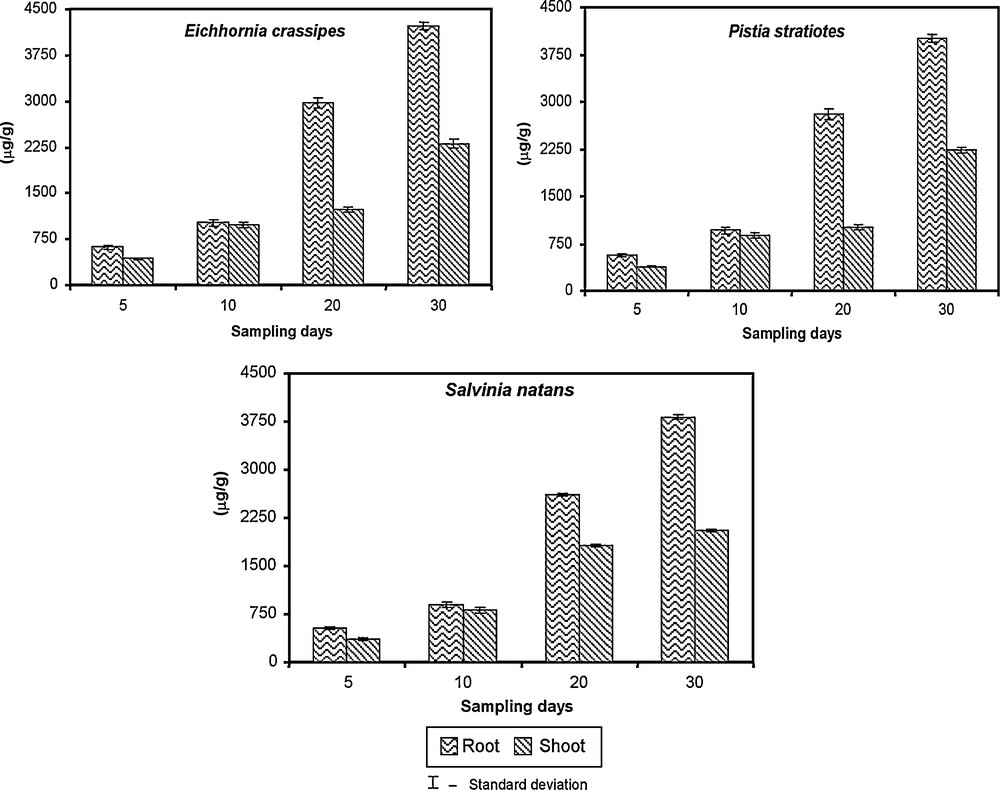
Removal of chromium by using aquatic plants.
5.3.2 Removal of chromium from polluted soil by terrestrial weeds
The data related to uptake and accumulation of chromium using grasses and weeds are given in Fig. 10. The phytoremediation performance of a plant is not only determined by its ability to achieve high metal concentrations within its tissues, but also by its ability to translocate the metal to aerial organs and to produce a high biomass. The efficiency of phytoextraction for remediation of heavy metals from contaminated soil is highly dependent on the bioavailability of metals. In this experiment, two grasses (Cyperus rotundus, Cyperus kylinga)and two weeds (Marselia quadrifolia, Ludwigia parviflora) were used for the phytoremediation study. All the species were allowed to grow up to 30 days in 100 mg/l chromium contaminated soil. These plants were harvested periodically (5, 10, 20 and 30 DAS) and separated into root and shoot to determine the chromium accumulation. The higher accumulation of chromium was recorded in the root of Cyperus rotundus (275.5 μg/g), Ludwigea parviflora (242.0 μg/g), Cyperus kylinga (251.6 μg/g) and Marsilea quadrifolia (214.0 μg/g) than in shoot (248.4, 212.0, 182.0 and 174.50 μg/g) at 30 days of exposure. Among the plants tested, the grass Cyperus rotundus and Ludwigea sp. accumulated more amount of chromium from contaminated soil followed by Cyperus kylinga and Marsilea sp. because of their natural physiological and biochemical abilities. The root tissues accumulate significantly greater concentrations of metals than shoots including high plant availability of the substrate metals as well as its limited mobility once inside the plant [30]. Moreover, a 60% reduction of chromium from the contaminated soil was recorded by using the plants such as Cyperus rotundus and Ludwigea sp. Plants growing in polluted soil absorb nourishments from polluted soil and entry of pollutants to the plant system during the process of absorption may not be ruled out. It was assumed that uptake of pollutants by plants may cause the lowering of pollution load of the soil.
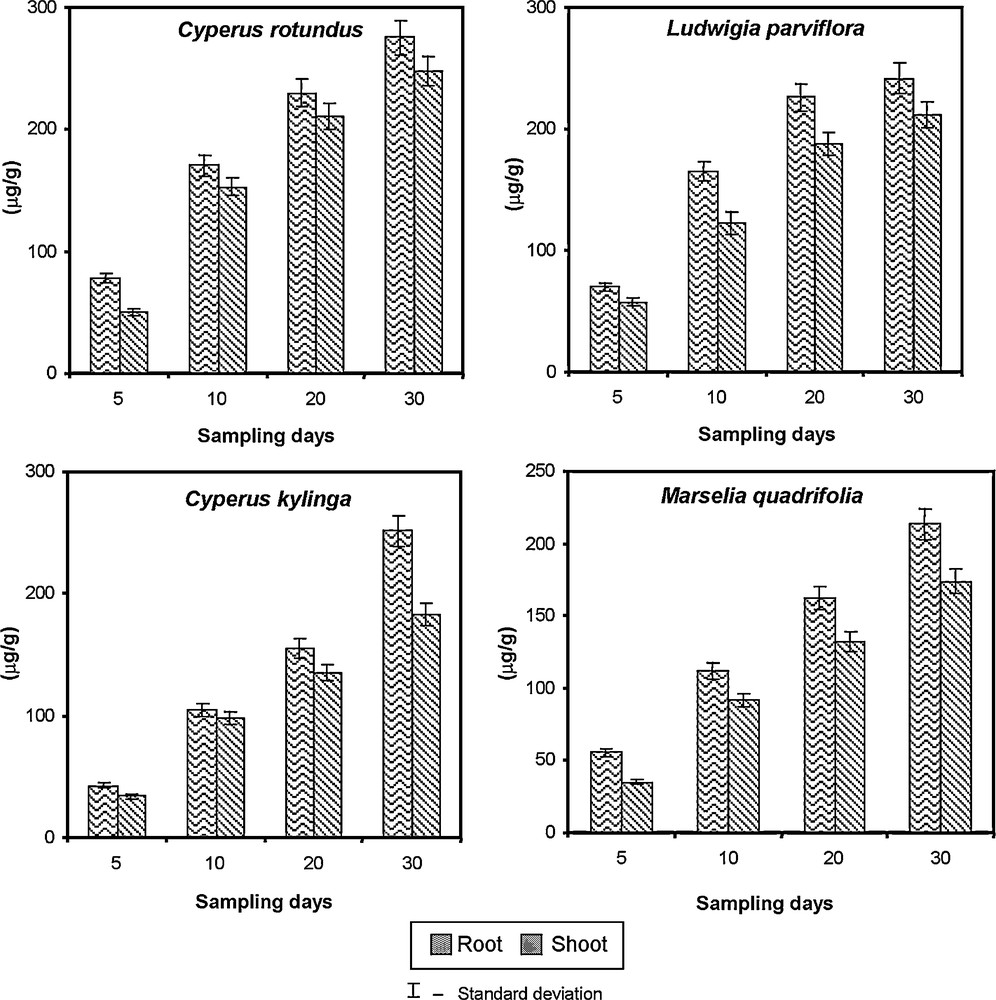
Removal of chromium from polluted soil by using grasses and weeds.
Brassica juncea and B. nigra have a better ability to take up heavy metals from soil substrates and to transport and concentrate these metals in their shoots. The plants for the phytoextraction process should tolerate and accumulate high levels of heavy metals in its harvestable parts. It must have a rapid growth rate and a potentiality to produce a high biomass in the field [74].
Besides hyperaccumulator plant species like Salix viminalis, Brassica juncea, Lolium perenne, Zea mays and Helianthus annuus, characterized by high content of heavy metals in biomass and have good remediation capacity [25].
The naturally growing dominant herbaceous species can also be used for this purpose because they have the adaptability to survive under stress of industrial effluents. The dominance of herbaceous flora in contaminated soil may be due to the fact that shrubs are more stable and less influenced by any small environmental conditions. The plants must have the ability to translocate chromium from the root to the shoot in order to continuous absorption of chromium from substrates. It can reduce chromium concentration and thus reduce toxic potential to the root and translocation to the shoot is one of the mechanisms of resistance to high Cr concentration [75]. The mechanisms involved in heavy metal tolerance may range from exclusion, inclusion and accumulation of heavy metals depending on the plant species [76,77]. It was found to be economically valuable to grow naturally growing weeds and grasses in order to soil effectively at larger scale in shorter time [78].
6 Conclusion
It can be concluded that the chromium contaminated water and soil are phytoremediated by naturally growing aquatic and terrestrial weeds. This phytoremediation technology can be employed wherever possible in order to detoxify the contaminated water and soil medium.