1 Introduction
The growth and yield of crop plants in different agricultural soils over the world are under the influence of drought and salinity. In these regions, irrigating soils with salty ground water results in higher range of salty and hence more unfertile soils. Under such conditions, plant growth decreases due to the excess uptake of Na+, Cl− and B as well as the nutritional imbalance of nutrients such as N and P (Saqib et al. [1]). Substantial alterations in protein and carbohydrate synthesis and in the activity of proteases and nitrate reductase also resulted under saline conditions (Misra and Gupta, [2]).
For example, although the expression of proteins at the early stages of plant exposure to salinity does not differ among the salt sensitive and tolerant wheat genotypes, at the later stages more differences in the expression of proteins appear (Saqib et al. [1]).
Arbuscular mycorrhizal (AM) fungi can contribute to plant establishment and growth under stress (Deressa and Schenk, [3]; Miransari et al., [4–7]). These fungi are able to develop symbiotic associations with most plant species, (Smith and Read, [8]) resulting in the transfer of carbon and nutrients from the host plant to the fungi and vice versa, respectively (Allen, [9]; Deressa and Schenk, [3]). They have some unique properties making them beneficial to the host plant under different stresses. AM fungi are able to produce a very extensive network of hyphae in the soil when in symbiosis with the host plant. The intraradical colonization of plant roots by AM fungi results in the formation of some specialized structure, including arbuscules (the organelle for the exchange of nutrients with the host plant) and vesicles (the storage organelle), which can significantly enhance the absorbing capacity of the root for water and nutrients (Rillig and Mummey, [10]).
AM fungi can enhance plant growth under salinity stress (SS), especially in soils with low level of P (Garbo et al., [11]; Feng et al., [12]; Mohammad et al., [13]) and are able to enhance plant tolerance under salinity through altering plant physiology and increasing water and nutrient uptake (Auge, [14]; Giri and Mukerji, [15]; Miransari et al., [5]; Miransari [16]; Daei et al., [17]).
For example, mycorrhizal plants absorbed less amounts of Na+ and Cl− or inhibit their transfer to the shoots (Scheloske et al., [18]), resulting in the increased dry weight of cotton by 68% under the salinity of 3 g/kg (Tian et al., [19]). In Vicia faba, inoculated with Glomus clarum at the salinity of 6 dS m−1, Na+ and Cl− were accumulated in the roots and were not transferred to the shoots (Rabie and Almadini, [20]). AM fungi influenced the transfer of K+, Ca2+ and H+ pump and enhanced the transfer of Ca2+ in the apoplast and cell organelles (Rabie and Almadini, [20]). Accordingly, the dry weight of lettuce plants inoculated with AM fungi at the salinities of control, 2, 4, and 12 dS m−1, increased by 3.4, 8.2, 11.7 and 29.3%, respectively, compared with non-inoculated plants, indicating the more effectiveness of AM at the higher levels of stress (Rabie and Almadini, [20]).
With regard to the significance of salinity in crop production, extensive amounts of research work have been conducted. However, use of biological methods, which are of agricultural and environmental significance, to alleviate soil stresses (Miransari et al., [4,5]) including salinity, particularly under field conditions (Miransari and Smith, [21]; Daei et al., [17]), have been much less documented. This is why this research work is unique as almost all the previous research experiments regarding the effects of AM fungi on plant growth under salinity have been performed under greenhouse or controlled conditions (Daei et al., [17]). Hence, the objective was to evaluate the effects of different AM species on nutrient uptake of different wheat cultivars, under field and greenhouse saline conditions. The results of this research work can account for a major part of mycorrhizal effects on wheat growth under salinity.
2 Materials and methods
2.1 Field experiment
2.1.1 Preparation of arbuscular mycorrhizal fungal inoculum
The same method was used to prepare AM fungal inoculum for the field and greenhouse experiments. Inoculum of Glomus mosseae, G. etunicatum and G. intraradices were isolated from the saline soils of Tabriz, Iran (Aliasgharzadeh et al., [22]) and produced in a four-month period on sorghum roots in sterilized sand, under greenhouse conditions (Miransari et al., [4,5]) in the relative humidity of 60% and daily (16 h) and nightly (8 h) temperatures of 28 and 25 °C, respectively. The species belong to the collection of Soil and Water Research Institute, Tehran, Iran.
Sorghum plants were ferti-irrigated using the method of Feldman and Idczac [23]. At the flowering stage, irrigation of pots was stopped and after 14 days, the shoot part was harvested and the mixture of milled plant roots and sand were used as inoculum. After harvesting the plants, the soil pots were counted for the number of spores resulting in almost six spores per gram of soil for each species. For the mixed inoculum treatment, three AM fungi species were mixed thoroughly resulting in 18 spores per gram of soil.
2.1.2 Wheat cultivars
Three wheat cultivars (Table 2), Roshan (R, local) and Kavir (K, genetically modified), and one mutated line, Tabasi (M) were used for the experiment (Daei et al., [17]). R is a spring cultivar, semi-early maturated, relatively tolerant to lodging, drought and grain senescence and the weight of its one thousand grains is 46 g. K is also a spring cultivar, early maturated, tolerant to lodging, grain senescence and soil salinity and the weight of its thousand grains is 36 to 40 g. M is characterized by higher yield, complete standing and tolerance to lodging. The M (T-65-7-1) was produced using some preliminary experiments. After determination of the related dose and seed moisture adjustment (11-13%), using Cobalt (Co60) source, seeds were radiated with γ radiation with a 200 g dose at 55 rad.min−1. The experiment was conducted in the year 2006–2007 in a 1500 m2 research farm in Shahryar, Alborz province, Iran.
The characteristics of wheat varieties used in the field and greenhouse experiments.
Field | Greenhouse | ||
Variety | Characteristics | Variety | Characteristics |
Roshan (R, local) | Is a spring cultivar, semi-early maturated, relatively tolerant to lodging, drought and grain senescence and the weight of its one thousand grains is 46 g | Chamran (CM) | Semi-resistant to salinity |
Kavir (K, genetically modified) | A spring cultivar, early maturated, tolerant to lodging, grain senescence and soil salinity and the weight of its thousand grains is 36 to 40 g | Line 9 (L9) | Resistant to salinity |
Mutated line, Tabasi (M) | Is characterized by higher yield, complete standing and tolerance to lodging |
2.1.3 Experimental procedure
Previous to planting wheat cultivars, soil physical and chemical properties and water chemical properties were determined (Daei et al., [17]). The experiment was a factorial based on a completely randomized block design in four replicates. Each replicate consisted of 12 treatments (plots). AM treatments including control (Blank), G. etunicatum (M1), G. mosseae (M2), and G. intraradices (M3) and wheat cultivars of inbred K, R and M were used as the experimental factors.
Before seeding, N (urea) and P (Triple Super Phosphate) fertilizer were applied at the total of 138 and 10 kg, respectively. The plots were surface fertilized with P and half of the N dose, which was then incorporated into the soil. The remaining part of N was applied twice at the stem and ear producing stages. Each plot measured 6 × 2 m with six rows, 25 cm apart. To avoid interactions between the plots, the side rows were not planted. Plots and replicates were 1.5 and 3 m apart respectively.
The seeds were inoculated underneath with AM fungal inoculum at 200 propagules (Most Probable Number, MPN method, Feldmann and Idczak, [23]) per seed (Miransari et al., [24]; Daei et al., [17]; Samarbahksh et al. [25]). The field was surface-irrigated with the saline water with respect to climatic and soil conditions and plant requirements at the appropriate time. Weeds were manually removed. At harvest and after ignoring the sidelines and the sampled parts, the total yield in each plot was determined using a handy combine.
To measure nutrient concentration in plant shoots, ten plots were randomly selected from each plot and after washing with tab and distilled water, were dried in oven at 70 °C for 72 h. Plant samples were then milled. In addition to N measurement (using the wet oxidation method), Ca, Mg, Fe, Cu and Mn (using atomic absorption spectrophotometry) Na, and Cl (using chloridemeter) counteraction were also measured. To determine root colonization, from each plot ten plants were harvested and after combining the samples using the method of Phillips and Hayman [26], one-gram root samples were stained (Giovannetti and Mosse, [27]) using the gridline–intersection method (Daei et al., [17]).
2.1.4 Statistical analysis
Data were subjected to analysis of variance using SAS (SAS Institute Inc., [28]), and mean comparison of treatments were also determined (Steel and Torrie, [29]). Data were normally distributed and hence, no transformation required.
2.2 Greenhouse experiment
2.2.1 Soil and water analyses
Soil physical and chemical properties were determined (Table 1). Soil texture was determined using the hydrometer method, pH and electrical conductivity (EC, dS/m) of a saturated paste and organic C using the Walkey and Black method [30] were determined. Total N using the Kjeldahl method (Nelson and Sommers, [31]), soluble P in sodium bicarbonate (Olsen, [32]), extractable K using ammonium acetate (Knudsen et al., [33]), Fe, Zn, Cu and Mn concentration using DTPA extractant and atomic absorption spectrometry (Baker and Amachar, [34]), Na using flame photometer and Cl in soil solution were determined (Richards, [35]). EC and pH of irrigation water was determined using electrical conductivity – and pH – meter, respectively (Rhoades, [36]). The concentration of Ca2+, Mg2+, carbonate and bicarbonate, and Cl were determined with the titration method, and K and Na using flame photometer (Richards, [35]).
Water and soil analyses, greenhouse experiment.
SAR | TC | Mg | Ca | Na | K | TO | Cl | CO3 | HCO3 | TDS | EC (dS m−1) | pHW | |||
mg L−1 | |||||||||||||||
0.459 | 4.959 | 0.961 | 3.22 | 0.74 | 0.033 | 5.15 | 1.15 | 0 | 4 | 35.90 | 0.359 | 7.51 | |||
Soil texture | P | K | Na | Ca | Mg | Fe | Mn | Zn | Cu | Cl | SP (%) | TN (%) | OC (%) | EC dS m−1 | pHW |
mg kg−1 | |||||||||||||||
Loamy | 4.8 | 338 | 112 | 402 | 187 | 2.17 | 6.62 | 0.91 | 1.38 | 164 | 32.7 | 0.08 | 0.56 | 1.7 | 7.21 |
2.2.2 Salinity treatments
Usually in saline soils, sodium and calcium chloride are the dominant salts (Keshavarz, [37]), hence, they were selected for the preparation of salinity treatments: saline solutions (containing the equal ratio of both salts) of 4, 8 and 12 dS/m were used. According to the ECe of soil and EC of irrigation water, the EC for each salinity treatment was determined. For the 4 dS m−1 treatment after one time irrigation and for the treatments of 8 and 12 dS m−1 after four times of irrigation every three days using the saline solutions, the favourite ECe was established in the pots.
2.2.3 Planting
To use as the growing medium the surface layer (0–30 cm depth) of a field (not cultivated for years) around Karaj, Iran was collected. After sieving the soil and mixing it with perlite (2:1 ratio), 10 kg pots were prepared. Fungal inoculum, at 100 g, was used in the middle of each pot. Ten sterilized seeds of wheat varieties (Table 2), Chamran (CM) and Line 9 (L9, Bank“S”/Vee“S”) as the semi-resistant and resistant varieties to salinity, respectively, were planted at each pot around the inoculum. They were introduced by the Seed and Plant Improvement Institute of Karaj, Iran.
At the three-leaf stage, two plants were removed from each pot and according to soil analysis, pots were ferti-irrigated by 17 g P (super phosphate triple), 0.89 g K (potassium sulfate) and 100 g N (urea, at planting, stemming and grain filling). To avoid salinity shock to the plants, pots were gradually treated with salinity treatments. Using 15 extra pots, the weekly soil sampling for the monitoring of soil salinity, to reach the final salinity level (SL), was conducted. Hence, the final salinities of 3.9, 7.85 and 12.1 dS/m were attained in the pots. The pots were then irrigated with distilled water and according to the pot weights, soil moisture was held at about 80% of the field capacity moisture. Plants were grown for four months under controlled conditions in a greenhouse under natural and artificial light and day and night temperatures of 20 and 17 °C, respectively.
2.2.4 Sampling and analyses
The two plants, harvested from each pot at stemming, were washed with distilled water and dried in the oven at 72 °C. Plant samples were then milled and analysed for the concentrations of N, K, P, Ca, Mg, Mn, Cu, Fe, and Zn, Na and Cl (Emami, [38]). Plant dry matter, root colonization percentage (Giovannetti and Mosse, [27]), and mycorrhizal efficiency (Boan et al., [39]) were also determined.
2.2.5 Experimental design and statistical analyses
The experiment was conducted as a factorial on the basis of a completely randomized block design in four replicates using the same inoculum, which had been tested under field conditions. Factors of: AM species, including control (Blank), G. intraradices (Gi), G. mosseae (Gm), G. etunicatum (Ge) and a mixture of the three AM species (mix); SL's of 4, 8, 12 dS/m, and; spring wheat varieties of CM and L9 were used as the experimental factors. Using SAS (SAS Inc., [28]) analysis of variance and Duncan's multivariate mean comparison at P = 0.01 were performed (Steel and Torrie, [29]).
3 Results
3.1 Field experiment
Analysis of variance indicated that while wheat cultivars significantly affected the concentrations of N, Ca and Mn, the effect of AM fungi on the concentration of all nutrients was significant. However, only N concentration was significantly affected by the interaction effect of wheat varieties and AM fungi (Table 3). Cultivar R had the highest concentration of shoot N (significantly different from K). M resulted in the highest concentration of Mg, significantly different from R. The highest concentrations of Ca, Cu and Mn were related to cultivar K, significantly different from R (Table 3).
Analysis of variance for different parameters, affecting the concentration of shoot nutrients and mean shoot concentration of different nutrients, averaged for different AM species and for different wheat genotypes, under field conditions.
Mean of sum squares | |||||||
SV | d.f. | N | Ca | Mg | Fe | Cu | Mn |
Replicate | 3 | 0.0016 | 0.0046 | 0.0015 | 184.269 | 6.436 | 0.004 |
Wheat | 2 | 0.039** | 0.013** | 0.0017 | 150.518 | 1.191 | 0.014* |
AM | 3 | 0.092** | 0.018** | 0.0022* | 2529.508* | 1.777* | 0.018** |
Wheat x AM | 6 | 0.072** | 0.005 | 0.0006 | 1451.566 | 0.15 | 0.005 |
Error | 33 | 0.006 | 0.0035 | 0.00065 | 741.026 | 0.487 | 0.003 |
CV | 3.18 | 18.71 | 15.08 | 14.3 | 14.30 | 18.5 | |
Treatment | N (%) | Ca (%) | Mg (%) | Fe (mg/kg) | Cu (mg/kg) | Mn (mg/kg) | |
K | 2.475b | 0.3465a | 0.1680ab | 124.78a | 5.074a | 0.3465a | |
M | 2.551ab | 0.3195ab | 0.1798a | 122.685a | 4.998a | 0.3195ab | |
R | 2.568a | 0.2842b | 0.1590b | 128.725a | 4.568b | 0.2863b | |
Blank | 2.400b | 0.2584b | 0.1536b | 106.60b | 4.426b | 0.2584b | |
Ge | 2.575a | 0.3375a | 0.1717ab | 137.49a | 4.746ab | 0.3375a | |
Gm | 2.586a | 0.3318a | 0.1645ab | 121.33ab | 5.328a | 0.3318a | |
Gi | 2.564a | 0.3442a | 0.1860a | 136.17a | 5.020ab | 0.3420a |
The three species of AM fungi followed the same trend of nutrient uptake, as concentration of N, Ca, Mg Fe, Cu and Mn were not significantly different among them, however significantly different from control for N, Ca and Mn. While inoculation of G. etunicatum resulted in the highest Fe concentrations in the wheat plants, G. mosseae resulted in the highest amount of N and Cu and for G. intraradices the highest concentrations were related to Ca, Mg and Mn. The lowest concentration of Cu was related to G. etunicatum, Ca, Mg, Fe and Mn to G. mosseae and N to G. intraradices (Table 3).
3.2 Greenhouse experiment
The effects of variety, AM fungi and salinity on all the measured parameters were significant at P = 0.01. The interaction effects of plant variety and AM fungi was significant on shoot dry matter, root colonization, N, K, Ca, Fe, Cl and Na uptake at P = 0.05. Parameters including shoot dry matter, root colonization, N, P, K, Ca, Fe, Cl and Na uptake were significantly affected by the interaction effects of plant variety and salinity at P = 0.05. With the exception of Zn and Na uptake, the interaction effects of AM fungi and salinity significantly affected all the other measured parameters. The interaction effects of AM fungi, salinity and plant variety were significantly affective on shoot dry matter, root colonization, N and Cl uptake (Table 4).
Analysis of variance for the effects of different parameters and their interactions on wheat nutrient uptake, under greenhouse conditions.
SV | df | SDM | RC | N | P | K | Ca | |
Variety (V) | 1 | 4.22** | 4914.94** | 11905.19** | 55.92** | 2179001.82** | 82518.53** | |
AM | 4 | 0.77** | 4977.38** | 91501.35** | 866.62** | 177420.71** | 1695.60** | |
Salinity (S) | 2 | 3.70** | 3325.58** | 33607.71** | 719.21** | 33624.90** | 1952.37** | |
V*AM | 4 | 0.11* | 268.62* | 2833.23* | 25.22 | 9432.79* | 244.20* | |
V*S | 2 | 0.12* | 369.73* | 1578.50* | 37.95* | 7126.76* | 213.49* | |
AM*S | 8 | 0.10* | 28.41* | 2526.16* | 31.59* | 3764.11* | 199.97* | |
AM*S*V | 8 | 0.02* | 29.45* | 618.26* | 4.02 | 1676.46 | 113.59 | |
Error | 90 | 0.029 | 7.47 | 186.38 | 10.38 | 1100.34 | 65.38 | |
CV | 4.67 | 5.29 | 5.11 | 8.70 | 6.55 | 9.63 | ||
SV | df | Mg | Fe | Mn | Zn | Cu | Cl | Na |
Variety (V) | 1 | 2644.03** | 0.512** | 4.358** | 0.13** | 0.011854** | 9.33** | 5104.00** |
AM | 4 | 447.87** | 0.393** | 0.272** | 0.17** | 0.01347** | 21138.5** | 278.82** |
Salinity (S) | 2 | 117.48** | 0.119** | 0.077** | 0.04** | 0.00197** | 2746.9** | 15.29* |
V*AM | 4 | 11.31 | 0.10* | 0.0087 | 0.004 | 0.00013 | 1472.5* | 263.38* |
V*S | 2 | 7.27 | 0.006* | 0.0086 | 0.001 | 0.000068 | 515.8* | 16.07* |
AM*S | 8 | 13.44* | 0.014* | 0.012* | 0.002 | 0.0023* | 494.7* | 11.26 |
AM*S*V | 8 | 8.85 | 0.003 | 0.0055 | 0.002 | 0.0001 | 909.9* | 22.62 |
Error | 90 | 8.01 | 0.002 | 0.0051 | 0.002 | 0.00005 | 146.9 | 5.79 |
CV | 9.62 | 5.42 | 8.179 | 12.03 | 6.85 | 7.2 | 5.81 |
Plant variety comparison indicated that under different SL's, L9 significantly increased plant N uptake compared with cultivar CM (Fig. 1). Relative to other treatments, the effect of mix treatment on N uptake for L9 at 8 dS/m of salinity was very similar to the first level of salinity (4 dS/m). It also resulted in the most alleviating effects on the highest level of salinity, compared with control, followed by G. mosseae.
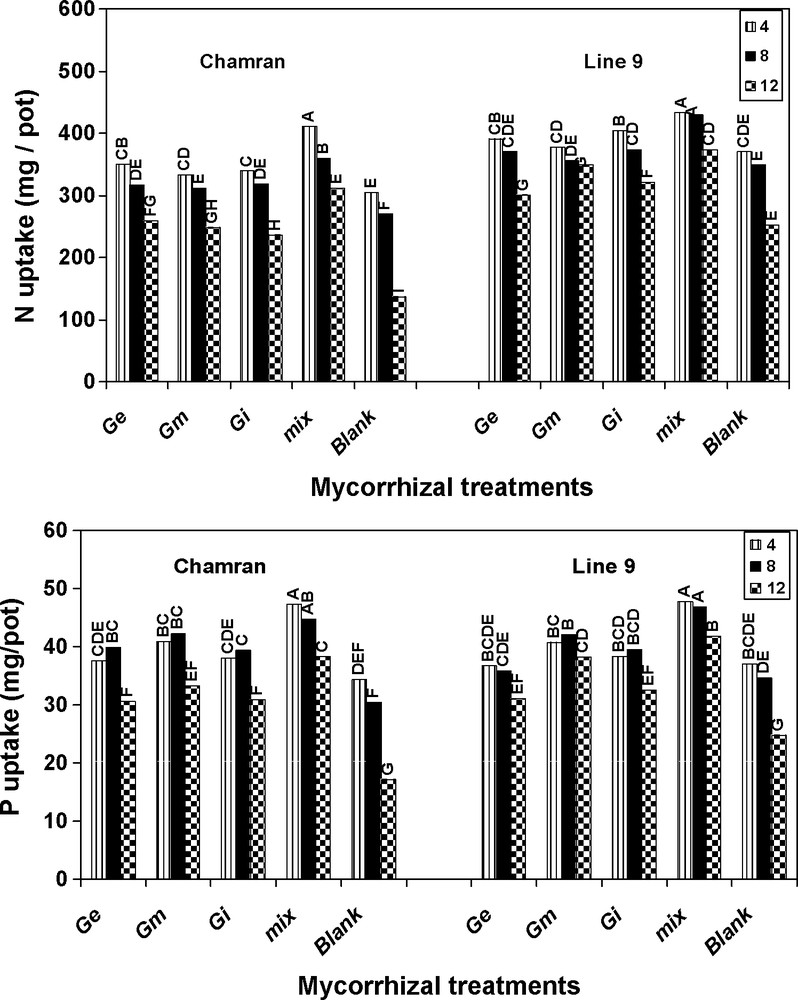
Effects of different arbuscular mycorrhizal treatments on N and P uptake (mg/pot), at different salinity levels, under greenhouse conditions. Ge: Glomus etunicatum, Gm: G. mosseae, Gi: G. intraradices and mix: the combined inoculation of all species. Blank: no inoculation. Means followed by the same letters are not significantly different.
In all cases, the amount of P at the second level of salinity by both cultivars was very similar to that of the first level. Treatment mix resulted in the highest and significant P uptake compared with the other treatments for both cultivars, followed by G. mosseae. Although the highest level of salinity significantly decreased P uptake relative to the other levels, AM species significantly enhanced P uptake at the highest level of salinity compared with control for both genotypes (Fig. 1).
L9 resulted in a higher K/Na ratio relative to CM. With increasing salinity, K/Na ratio decreased. However, compared with control, the ratio was significantly higher even at the highest level of salinity. In addition, different AM treatments significantly increased K/Na ratio relative to the control treatment (Fig. 2). The results of this research work also suggest that AM species significantly increased Zn uptake for both genotypes in comparison with the control treatment even at the highest level of salinity. Higher rate of Zn uptake was resulted by L9. Moreover, there were not significant differences between the first and second level of salinity for different AM species. The mix treatment resulted in the highest Zn uptake by both genotypes and for L9 the increase was significantly higher than the control treatment (Fig. 2).
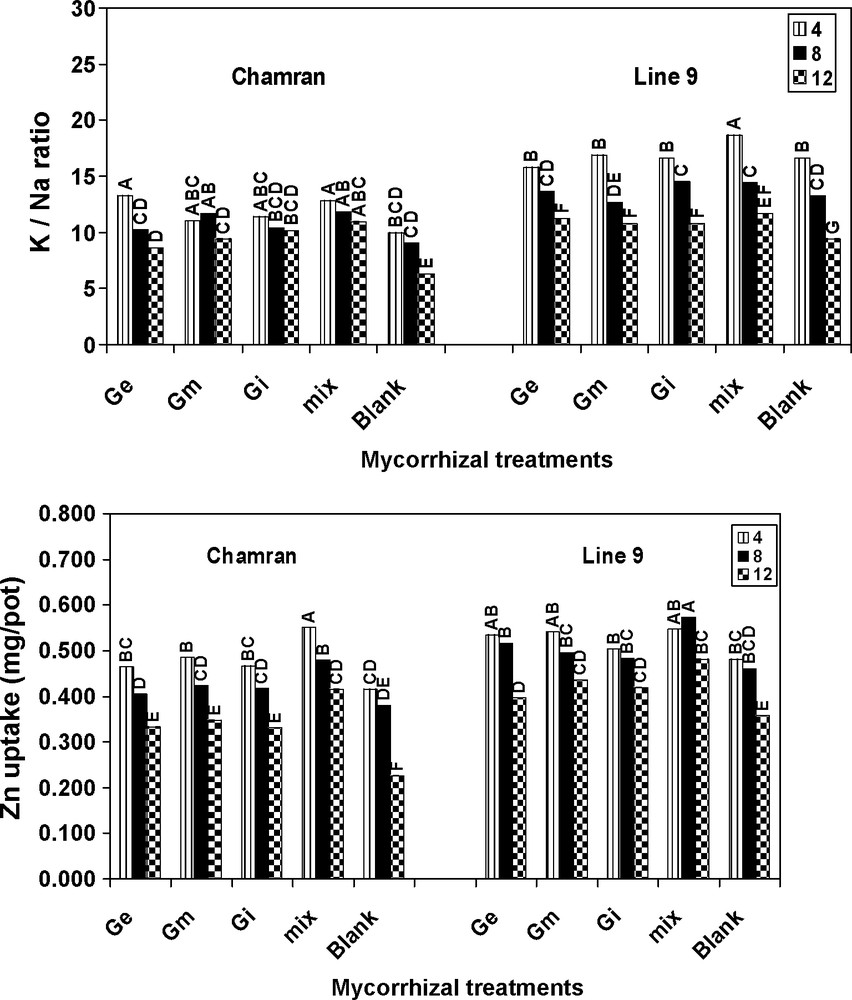
Effects of different arbuscular mycorrhizal treatments on K/N ratio and Zn uptake at different salinity levels, under greenhouse conditions. Ge: Glomus etunicatum, Gm: G. mosseae, Gi: G. intraradices and mix: the combined inoculation of all species. Blank: no inoculation. Means followed by the same letters are not significantly different.
AM fungal effectiveness on shoot dry weight of CM was much more pronounced, particularly at the highest level of salinity, compared with L9 indicating the higher effectiveness of AM on shoot growth of CM under salinity. Treatment mix in CM resulted in the highest increase in shoot growth compared with L9. AM species were able to colonize CM roots more efficiently under different SL's, compared with L9 and control treatment (Fig. 3). AM species also increased K, Ca, Mg, Fe, Mn, Cu and Na uptake, especially in L9. Although the mix treatment partially decreased Na uptake at the highest level of salinity compared with the other levels for CM, chloride uptake was significantly increased with increasing SL's and absorbed at a higher rate by CM, compared with L9 (data not shown).
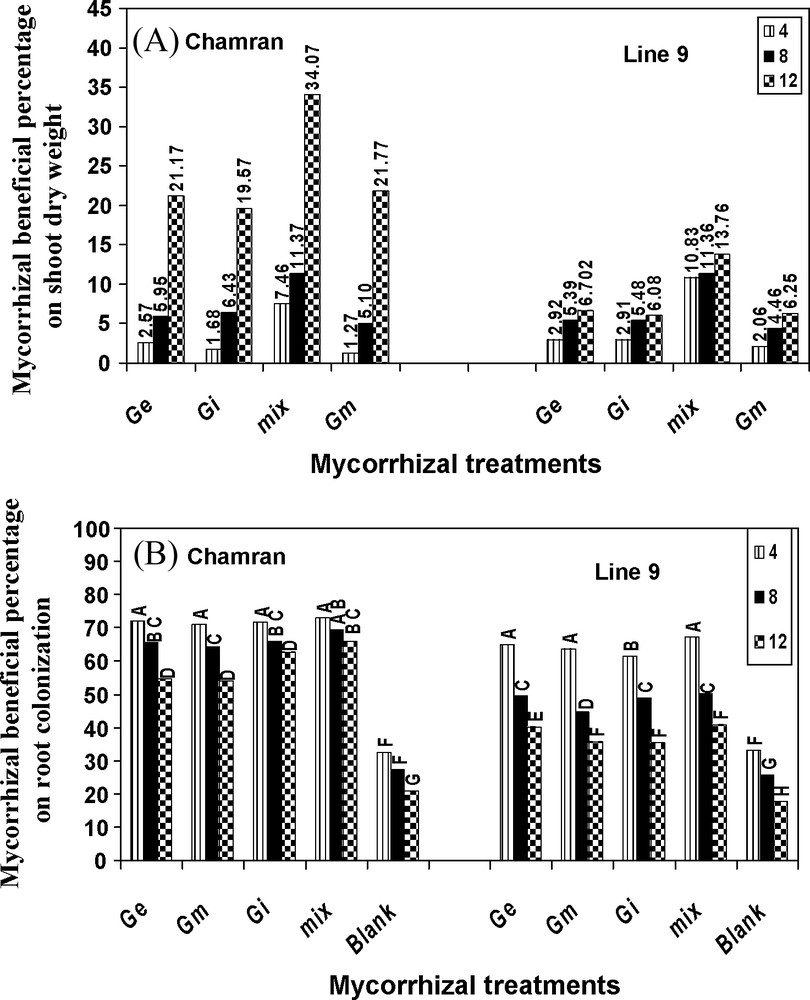
Effects of different arbuscular mycorrhizal treatments on AM beneficial percentage on shoot dry weight (A) and root colonization (B), under greenhouse conditions. Ge: Glomus etunicatum, Gm: G. mosseae, Gi: G. intraradices and mix: the combined inoculation of all species. Blank: no inoculation. Means followed by the same letters are not significantly different.
4 Discussion
In the present research work, different species of AM fungi: G. etunicatum, G. mosseae and G. intraradices enhanced the absorbing capacity of different wheat cultivars for N, Ca, Mg, Fe, Cu and Mn, in a salty soil, irrigated with a very saline ground water under field conditions. In addition, macro- and micronutrient uptake, plant growth, and root colonization were also affected by different species of AM under greenhouse conditions. Researchers have stated that AM fungi are able to enhance plant nutrient uptake under stresses such as salinity (Al-Karaki et al., [40]; Feng et al. [12], Porras-Soriano et al., [41]; Shokri and Maadi, [42]). However, what makes this research work unique is the use of a very salty ground water in a salty land for the cultivation of wheat plants inoculated with AM fungi. This can indicate the ability of AM fungi even under such stressed conditions. Accordingly, it verifies the necessity of using AM fungi for plantation of wheat under such conditions resulting in enhanced ecosystem efficiency (Miransari, [43]).
Although the symbiosis between AM fungi and the host plant is non-specific, however, under stresses such as salinity, there may be combinations of AM-host plant, which are more efficient (Daei et al., [17]). Hetrick et al. [44] stated that different wheat cultivars are able to perform differently irrespective of their mycorrhizal symbioses, however, other researchers indicated that the higher root colonization and hence, higher nutrient uptake, are the most important reasons for the greater performance of mycorrhizal tolerant varieties under salinity (Cantrell and Linderman, [45]; Al-Karaki, [46]; Daei et al., [17]; Miransari, [16]). In addition, AM species are able to increase plant growth under salinity by affecting root activities, enhancing plant photosynthesis and adjusting osmotic potential (Al-Karaki, [46]; Miransari et al., [5]; Daei et al., [17]).
Mechanisms affecting the performance of wheat cultivars to absorb nutrients include the rate of root nutrient uptake and their utilization by plants, as well as the amount of nutrient translocation to the plant (Hart et al., [47]). Researchers have also found that divalent cations are bound by the cell wall making them resistant to desorption even at the presence of high concentration of other cations (DiTomaso et al., [48]; Lasat et al., [49]).
In the present study, the significant interactions also indicate that the effects of AM species on the alleviation of salt stress are under the influence of other factors including SL and crop varieties. According to the results of both experiments, which are also in accordance with the results of other researchers (Audet and Charest, [50]; Subramanian et al., [51]) and of our group (Miransari et al., [4,5]), the effects of AM fungi on the alleviation of SS became greater with increasing the level of stress. It should also be mentioned that the soils tested in our experiments are of very little biological activities as they have not been under cultivation, hence, in case if there are any indigenous AM species in the soil, their effects on plant growth and their interactions with the introduced species are ignorable.
It can also be stated that there is a necessity for the recognition of salt tolerant genes and hence conferring such abilities to non-tolerant cultivars as was tested in this research work using local and the genetically modified varieties. Such differences in the AM species and wheat cultivars may be attributed to different morphological and physiological properties among different host plants and AM species resulting in more beneficial symbioses for some AM-host plant combinations (Miransari, [52]).
In previous reports, under salinity, mycorrhizal M was able to absorb fewer amounts of Na+ and Cl− (Daei et al., [17]), which has also been indicated by other researchers (Giri and Mukerji, [15]; Scheloske et al., [18]; Al-Karaki, [46]). It was suggested that in mycorrhizal plants, Na+ may be kept inside root cell vacuoles and intraradical fungal hyphae and may not be allocated to the shoots (Cantrell and and Linderman, [45]). The dilution effect, which is resulted by the enhanced growth of mycorrhizal plants, may also explain the lower amounts of Na+ in mycorrhizal plants under salinity (Jarrell and Beverly, [53]; Al-Karaki, [40]).
Daei et al. [17] indicated that under salinity, mycorrhizal M absorbed higher amounts of P, K and Zn and produced higher rate of root and yield. Similarly in their work, the species G. etunicatum and G. mosseae were proved to be the most efficient species.
It should also be mentioned that under stress, more C is allocated to the roots, resulting in more efficient plant performance (Miransari and Smith, [21,54]; Miransari et al., [4,5]) and higher rate of root colonization and hence, symbiosis (Daei et al., [17]). Our previous studies showed that the higher P absorption in mycorrhizal plants under different conditions, for example salinity, among its other important roles, produce higher amounts of roots (Miransari et al., [4,5]), which can be very beneficial under stresses (Miransari and Smith, [21]).
M genotype resulted in the higher K/Na ratio and the difference was significant between inoculated and non-inoculated varieties, which can be another reason for the higher tolerance of M under salinity. Rabie and Almadini [20] also observed that mycorrhizal plants are able to absorb higher rates of K under salinity relative to the control treatments, which can be very advantageous under SS.
The differences in the abilities of different plant genotypes for the exclusion of Na+ and K+/Na+ adjustment can also indicate the different performance of plant genotypes under salt stress (Munns and James, [55]). It is known that for the balance of nutrients in the tonoplast and plasma membrane, the process of ion pump can adjust the allocation of salt to the cell (Zhu, [56]). Hence, it can be expressed that the nutritional balance in mycorrhizal plants may also be related to the effects of AM fungi on the process of cellular ion pumping.
In addition, under greenhouse conditions, the two cultivars performed differently and although L9 resulted in higher nutrient uptake, the beneficial effects of AM fungi on CM growth was higher than L9 possibly due to a higher root colonization of AM species. However, the less root colonization by AM species and higher nutrient uptake of L9 under different SL's indicate that AM root colonization of L9 was more efficient than CM.
The superior performance of the mix treatment in many cases compared with the effects of individual AM species indicates the synergistic effects of AM species and hence, higher enhanced plant nutrient uptake and growth. The significant differences between different SL's with control treatments regarding plant nutrient uptake and growth indicate that even at the highest level of salinity, AM species are able to alleviate the stress on plant nutrient uptake and growth through their unique specifications. For CM, the mix treatment alleviated the effect of salt on plant growth through decreasing Na uptake. Sodium and Cl behaved absolutely differently and the results indicate that the alleviating effects of AM species on plant growth under salinity are more related to the adjusted Na uptake rather than Cl uptake. According to our results, mycorrhizal plants had no control on plant Cl uptake.
5 Conclusions
According to the results of this research work, with the use of AM fungi (for example, the mix treatment) it is likely to cultivate wheat in a saline soil (EC = 7.41 ds/m) using salty water (EC = 13.87 dS/m) under field and greenhouse conditions (EC = 12 dS/m). This is actually the part that makes this research work unique. Different species of AM fungi including G. etunicatum, G. mosseae and G. intraradices significantly enhanced plant nutrient uptake and growth by different wheat cultivars and G. etunicatum and G. mosseae were the most efficient species. The results also indicate that the selected combination of AM species and wheat cultivar can result in the highest rate of nutrient uptake by wheat plants under saline conditions. The synergistic effects of the tested AM species on the growth of wheat plants are indicated by the results of these experiments. Alleviation of salt stress on wheat growth by AM species is more through adjusting Na rather than Cl uptake. The great potential of AM fungi to alleviate stresses such as salinity on plant growth, especially under field conditions are verified by our results.
Disclosure of interest
The authors declare that they have no conflicts of interest concerning this article.