1 Introduction
Osmotic regulation in mammals is in part under the control of the neurohormones vasopressin (VP) and oxytocin (OT) that are produced by magnocellular neurons in the hypothalamus constituting the hypothalamo-neurohypophysial system (HNS). The somata and dendrites of the neurons accumulate mainly in the supraoptic (SON) and paraventricular (PVN) nuclei of the hypothalamus and their axons project to the neurohypophysis or neural lobe (NL) of the pituitary. Over the past 50 years, the rat HNS has served as model for the study of most aspects of neurosecretion and many electrophysiological and cell biological properties of the neurons have been defined under different physiological conditions. Moreover, it is well established that when these neurons are strongly or chronically solicited, their major synaptic inputs controlling their activity as well as associated glial astrocytes, undergo a remarkable morphological plasticity [1].
Desert rodents appear as unique models for the study of neurosecretion and of HNS peptides, in particular. They have an impressive ability to adapt to extreme osmotic environments. Heat and aridity, water deprivation, high insolation and convection with consequent high evaporation characterize their biotope. For desert mammals, hydro-osmotic regulations are more important than for any other mammal because of their dry environment and the high salt content of their diet. Several physiological studies have described the surprising renal concentrating power displayed by desert rodents [2], which is linked to the particular structure of their kidneys with their long renal papilla [3,4]. In addition, it has been reported that the concentration of VP in the NL and plasma of these species is significantly higher than in laboratory rats [5–7]. Such characteristics allow such animals to survive prolonged periods of drought and thus inhabit extremely arid areas successfully.
Two species that exist in particularly difficult conditions of water shortage and state of highly chronic dehydration in the Algerian desert are Gerbillus tarabuli and Psammomys obesus. The former is nocturnal and granivorous, whereas the latter is diurnal and herbivorous. Both species obtain water from their food, eating leaves and stems of chenopod plants with high salt content; they rarely drink. Because of such drastic conditions, it is highly probable that the HNS of these species displays particular characteristics linked to highly activated neurosecretion. Nevertheless, because of difficulties in the capture and subsequent maintenance of these animals in the laboratory, relatively few studies have been conducted until now.
In the present study, we obtained sufficient animals to allow morphological and immunocytochemical analyses of their VPergic and oxytocinergic systems. We also used electron microscopy to determine whether their hypothalamic magnocellular nuclei display any of the characteristics typical of the activity-dependent morphological remodeling of the HNS until now described in laboratory rodents, like the rat and the mouse [8,9]. Finally, we determined whether their HNS expresses plasticity-related glycoproteins, and in particular, the highly sialylated isoform of the Neural Cell Adhesion Molecule (PSA-NCAM) that our earlier studies [9–11] showed to be indispensable for neuronal and glial transformations, at least in the rat and mouse HNS under different stimuli of neurosecretion.
2 Materials and methods
2.1 Animals
Wild adults from male and female P. obesus and G. tarabuli species were captured in the desert (near Beni Abbès, Southwest of Algeria) from March to June. Captured animals were housed in a research facility (Unité de Recherches sur les zones Arides de Beni Abbès) for three days before sacrifice. During the 3-day captivity, animals were exposed to a temperature of 22 ± 2 °C and a light-dark cycle (12L-12D) and fed ad libitum with chenopod plants (Psammomys) and barley seeds (Gerbillus). Seventeen G. tarabuli (35–50 g) and 15 P. obesus (80–100 g) were used for these studies. All manipulations conformed to local and international guidelines on ethical use of animals.
2.2 Tissue fixation
Animals were deeply anesthetized with urethane (1.5 mg/kg), injected intracardially with heparin and then perfused with fixative. One group of animals was perfused with solution composed of freshly prepared 4% paraformaldehyde and 0.1% glutaraldehyde in sodium phosphate buffer (0.1 M, pH 7.4, 200–300 ml during 20 min, at room temp). After postfixation in 4% paraformaldehyde overnight (4 °C), brains were removed and cut on vibratome to obtain serial frontal slices (about 30–50 μm) which underwent immunolabeling for light microscopy.
Another group of animals was perfused with a freshly prepared solution of 4% paraformaldehyde and 2.5% glutaraldehyde in 0.1 M sodium phosphate buffer (pH 7.4; 200–300 ml during 20 min, room temp). Following perfusion, brains were removed and postfixed for 2 h in the same solution. Blocks containing the SON were then dissected from frontal slices of the brain. These tissues were prepared further for electron microscopy.
2.3 Immunohistochemistry
For single immunolabeling, standard immunofluorescence and immunoperoxidase techniques were performed on free-floating sections of brains according to procedures described in detail in earlier studies [12–14]. Briefly, after rinsing in Tris-buffered saline (TBS), sections were treated with casein (0.5% in PBS) for 1 hr to block non-specific sites. They were then incubated for 24–48 hr at 4 °C with: (i) polyclonal rabbit antibodies against either VP (diluted 1:5000–1:10 000) or against OT (1:400–1:1000) [15] (gift of A. Burlet); or, (ii) monoclonal mouse IgM antibody that specifically recognizes PSA on NCAM (diluted 1:2000–1:8000) (gift of G. Rougon), (see [16] for production and specificity). Affinity-purified anti-rabbit or anti-mouse immunoglobulins (IgG) conjugated to fluorescein isothiocyanate (FITC, Biosys) (diluted 1:400, 2 hr, at room temperature) or FITC-conjugated anti-mouse IgM (diluted 1:100) were used. For immunoperoxidase, after incubation with anti-rabbit or anti-mouse Igs (diluted 1:200 or 1:400 respectively), sections were incubated with rabbit peroxidase-antiperoxidase (PAP, Dako) complexes (diluted 1:100) or with anti-mouse IgM conjugated to horseradish peroxidase (HRP, 1:50). Peroxidase reaction product was revealed either with 3, 3′-diaminobenzidine (DAB) and 0.01% H2O2 or with the more sensitive glucose oxidase-nickel-DAB method.
In some cases, double immunofluorescence was performed on free-floating sections incubated for 48 hr at 4 °C in mixtures of primary antibodies containing the polyclonal rabbit IgG anti-VP (diluted 1:5000) and monoclonal mouse antibodies specific for OT-linked neurophysin (OT-Np; diluted 1:500) [17] (gift of H. Gainer). After careful rinsing, the sections were incubated for 2 hr at room temperature in a mixture of fluorescent conjugates. Rabbit FITC-conjugated anti-rabbit Igs (diluted 1:400) were used to identify VP immunoreactivity, whereas anti-mouse Igs conjugated with Texas Red (TR) (Biosys, diluted 1:500) were used to visualize OT-Np immunoreactivities. The preparations were examined with light microscopy (Leica DMR) using bright field optics for HRP-containing sections and epifluorescence with appropriate filters for FITC- or TR-treated sections.
Controls: controls included omission of primary antibodies and incubation in normal rabbit serum or with irrelevant secondary antibodies. These sections did not show any labeling.
2.4 Electron microscopy
Following glutaraldehyde fixation and rinsing, tissues were postfixed in 1% OSO4 in Millonig's buffer (1 hr at room temp), dehydrated in ascending series of ethanol and flat embedded in Epon resin. During dehydration, they were treated with 1.5% uranyl acetate (in 50% ethanol) for 30 min en bloc to enhance contrast. After identification on semithin sections, silver-gray (about 60-80 nm) ultrathin sections were obtained on 300-mesh grids and contrasted further with lead citrate before examination on Philips CM10 electron microscope (SERCOMI Electron Microcopy Center, University of Bordeaux).
3 Results
3.1 The hypothalamic magnocellular nuclei of desert rodents
In comparison with laboratory rodents and other mammals [18–21], the HNS system of the two desert rodents, P. obesus and G. tarabuli, appeared highly developed. Whatever the immunocytochemical method that we applied, we observed many neurons immunoreactive for either of the HNS peptides in sections from both species (Figs. 1 to 3). Their neurohypophyses were also strongly immunoreactive for VP or OT.
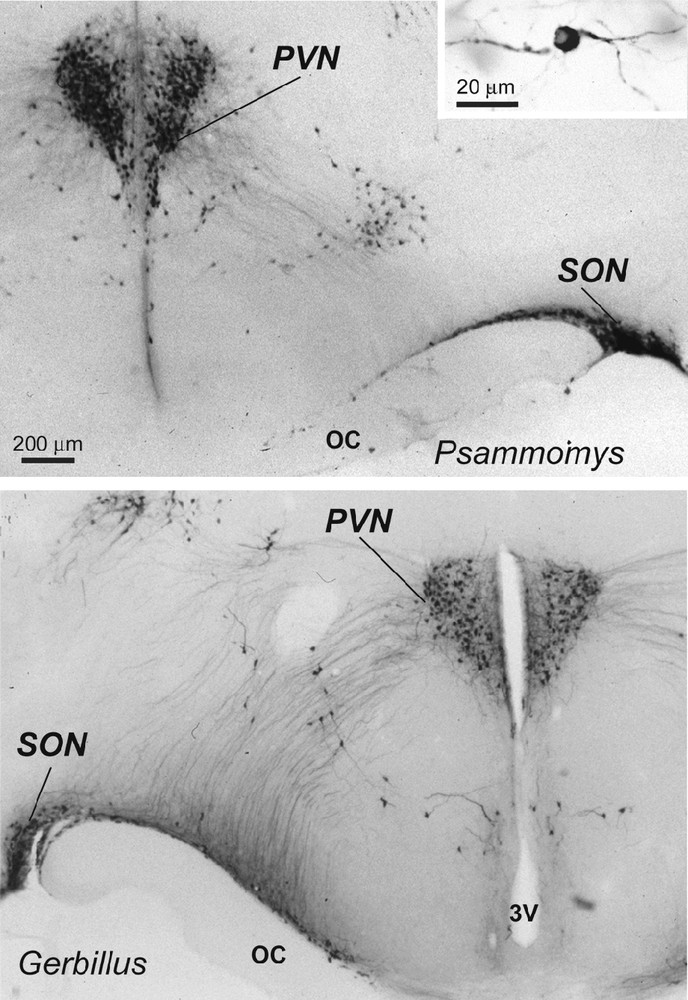
Light micrographs of frontal sections through the hypothalamus of two desert rodents (Psammomys obesus and Gerbillus tarabuli), showing magnocellular vasopressin (VP) neurons (and at higher magnification in the inset) in the supraoptic nucleus (SON), paraventricular nucleus (PVN) and in accessory groups between the two nuclei. Immunoperoxidase reaction with a polyclonal serum specific for VP; bright field optics. OC: optic chiasma; 3V: third ventricle.
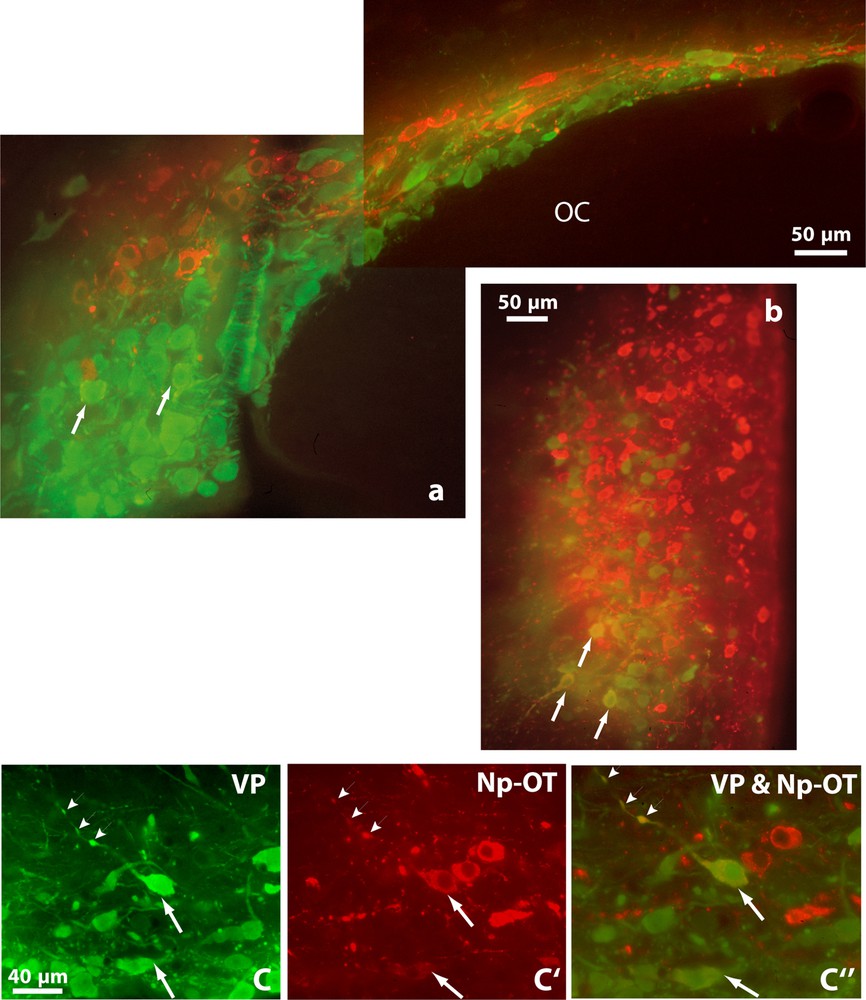
Double immunofluorescence shows vasopressinergic (VP, green) and oxytocinergic (OT-Np, red) neurons in the SON (a), PVN (b) and in the retrochiasmatic portion of the SON (c, c’ and c”) of Psammomys obesus. It also reveals many neurons showing immunofluorescence for both peptides (orange), in their somata (arrows) and axonal varicosities (arrowheads). OC: optic chiasma. Epifluorescence with appropriate filters.
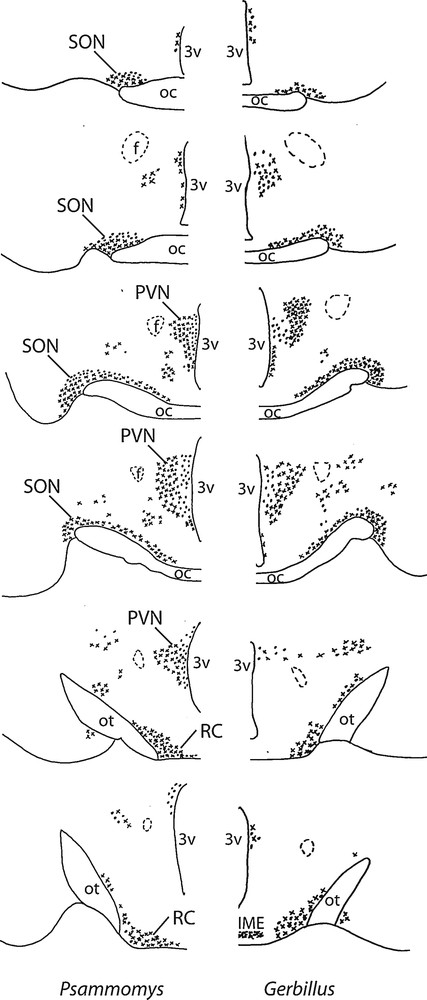
Diagrammatic representation of the distribution of vasopressinergic and oxytocinergic neurons in coronal sections of the SON (about 150 μm far from each other), including its retrochiasmatic portion (RC), PVN and accessory nuclei in two desert rodents (Psammomys and Gerbillus). The neurons were visualized with the immunoperoxidase method using antibodies against VP or OT. OC: optic chiasma; ot, optic tract; 3V: third ventricle; IME: internal median eminence; f: fornix.
In the hypothalamus of Psammomys and Gerbillus, magnocellular VP- and OT-positive neurons were visible in a number of areas (Figs. 1 to 3). Strong immunolabeling characterized their somata that were round to ovoid (20–30 μm in diameter) and possessed two to three primary dendrites and an axon with varicosities (insert, Fig. 1; Fig. 2c). The fibers were also strongly immunoreactive. As the other species, the magnocellular neurons in desert rodents accumulated in the well-delineated paired PVN and SON (Figs. 1, 2), and in the latter, they were particularly striking in its retrochiasmatic portion (RC) (Figs. 2, 4). In addition, they were easily detectable in the hypothalamic region between the magnocellular nuclei, including in those areas referred to as accessory nuclei (anterior commissural, perifornical and circularis nuclei) (Figs. 1, 3). A semi-quantitative evaluation revealed that VP neurons dominated in the SON (about 2 times more in its principal part and four times in its retrochiasmatic part) and in accessory nuclei. In the PVN, the number of VP and OT neurons appeared equivalent. Bundles of vasopressinergic and oxytocinergic nerve fibers were visible in the internal portion of the median eminence.
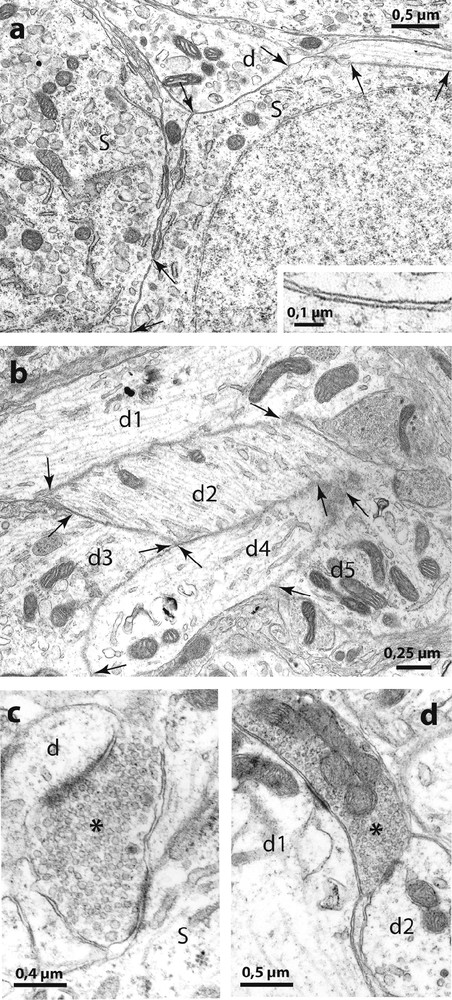
Electron micrographs of the SON of Psammomys. In (a) are shown two directly juxtaposed somata (S) and a dendrite (d) without interposition of astrocytic processes (at arrows). The higher magnification in the inset shows that the extracellular space appears normal between the juxtaposed neuronal membranes. (b): bundles of directly juxtaposed dendritic profiles (d1 d2, d3, d4 and d5) were also frequent. (c) and (d) illustrate ‘multiple’ synapses where axon terminals (asterisks) make synaptic contact simultaneously on somata (s) and dendrites (d).
3.2 Colocalization of neurohypophysial peptides
Double immunofluorescence revealed that many neurons displaying strong immunoreactivity for VP also showed reaction for OT (Fig. 2). Doubly labeled cells were detected in both magnocellular nuclei; they were particularly visible in the SON, mainly in its RC, (Fig. 2a and c). A semi-quantitative evaluation, on five to ten frontal sections of hypothalamus containing the SON in both species, revealed that immunoreactivities coexisted in about 20–30% of magnocellular neurons.
3.3 Neuro-glial configuration
Activity-dependent neuronal-glial and synaptic changes have been detected and analyzed with electron microscopy in the rat [8] and in the mouse [9]. In the present study, therefore, we examined the SON of desert rodents with electron microscopy and found that the magnocellular nuclei in these species displayed ultrastructural features characteristic of the activated HNS in laboratory animals. As in other species [8], the SON of desert rodents is relatively homogeneous and is composed essentially of magnocellular somata, dendrites, astrocytes and their processes as well as other neuropile elements. As in chronically dehydrated rats [8] and mice [9], the SON of the two desert rodents displayed many directly juxtaposed neuronal profiles, not separated by the fine astrocytic processes that normally separate neuronal elements (Fig. 4). The neuronal juxtapositions were visible between adjacent magnocellular somata and between dendrites (Fig. 4a) that often appeared as bundles (Fig. 4b). In addition, as in laboratory rodents in which HNS secretion is strongly stimulated [1], we found many axonal buttons making synaptic contact with two or more somatic and/or dendritic profiles in the same plane of section (‘multiple’ synapses, Fig. 4c).
3.4 PSA-NCAM is detected in the hypothalamic nuclei of desert rodents
Using an antibody that specifically recognizes PSA on NCAM [16], and immunoperoxidase and immunofluorescence techniques, we detected high levels of PSA-NCAM immunoreactivity in the hypothalamus of the two desert rodents.
In hypothalamic sections, a striking immunoreactivity was noted in both the PVN (Fig. 5a) and SON (Fig. 5c). The immunoreactive signal was particularly strong in the ventral portions of the SON and in its ventral glial lamina (Fig. 5c), where neurosecretory dendrites and astrocytic processes accumulate. In the PVN and SON, it filled the neuropile surrounding the magnocellular somata, which were immunonegative (Fig. 5b and d). As in the stimulated rat SON [10,12], there was no PSA immunoreactivity around clusters of closely juxtaposed neuronal somata (Fig. 5b and d).

Light microscopic localization of polysialylated Neural Cell Adhesion Molecule (PSA-NCAM) in the hypothalamic magnocellular nuclei of desert rodents. Both immunoperoxidase (a, b) and immunofluorescence (c, d) reactions reveals a striking immunoreactivity for polysialic acid (PSA) in the PVN (a and b), and SON (c and d). Note that in the PVN (a and b) and SON (c and d), PSA immunoreactivity is particularly strong in the neuropile around immunonegative magnocellular somata. There is less reaction where there are clusters of closely apposed magnocellular somata (S1–S5) (b and d). Adjacent hypothalamic structures, including the optic chiasma, are not labeled above background levels (c).
4 Discussion
The present observations reveal that the hypothalamic magnocellular nuclei of desert rodents like Psammomys and Gerbillus are in a hyperactive state and present several characteristics favoring neurosecretion.
From our immunocytochemical observations, it is obvious that the hypothalamic magnocellular nuclei in both species were very developed. In comparison with the rat [18,21], the nuclei were composed of a greater number of magnocellular neurons that displayed a high degree of immunostaining for either OT or VP, as shown by immunocytochemistry. Another feature of heightened activity was the numerous neurons that displayed colocalization of the peptides. Indeed, as shown by earlier studies in the rat [22–24], under normal basal levels of neurosecretion, few HNS neurons display colocalization of OT and VP while under conditions like lactation and dehydration [22,24], their incidence is significantly enhanced (up to 16% in the second day of lactation) [22]. In the present study, we found over 20% in either species in which both peptides were clearly colocalized.
Using electron microscopy, we were able to detect additional properties of heightened neurosecretion in these animals. Thus, the cytological characteristics of the somata and dendrites of these neurons in the SON reflect heightened proteosynthesis and neurosecretion. In addition, the neuronal-glial and synaptic organization of the nucleus was that characterizing the nuclei of dehydrated rat [8,25,26] and mouse [9] and many neuronal juxtapositions without glial interposition and “multiple” synapses were apparent.
Finally, as in other rodents (rat and mouse) [9,12,13], the HNS of desert species shows the presence of ‘embryonic’ molecular features now considered as markers for neuronal, glial and synaptic morphological plasticity. In particular, using immunocytochemistry, we detected high level of PSA-NCAM, which is known to reduce cell adhesion and to promote dynamic cell interactions and which has been shown to be essential as a ‘permissive molecular factor’ of such plasticity in the rat and mouse HNS [9,11,27].
4.1 Presence of HNS peptides in desert rodents in need of water retention
In comparison with the rat and other mammalian species [18,19,21,28], the hypothalamic magnocellular system of the two desert rodents, P. obesus and G. tarabuli, which is constituted by the neurons secreting VP and OT is very developed. These neurons, which appear far more numerous than those in the laboratory rat, for example, accumulate in well defined areas, the paired supraoptic, PVN nuclei and a number of accessory nuclei. As in the other rodents, VP and OT neurons in the desert species display loose homotypic segregation in the SON, PVN and accessory nuclei but there is no strict separation of the two populations and the two types of neurons intermingle. In our models, the vasopressinergic neurons clearly predominated in the SON whereas in the rat, within the SON, neurons producing VP are found in approximately equal number with OT neurons [18,19]. The retrochiasmatic part of the SON is also much extended largely, composed of VP producing neurons. All these observations reveal the importance of VP in these desert species. That, the vasopressinergic system is well developed in these desert animals is not surprising when one considers that the neurohormone serves to increase the permeability of the renal ducts and thus to concentrate urine, a function of obvious value for survival of a desert rodents that do not drink free water and that must conserve all water obtained from its food and cell metabolism.
In addition, the strong immunostaining of VP and OT observed in SON and PVN of desert rodents was due to an accumulation of the immunoreactive material occupying all the cytoplasm and dendrites. Furthermore, as shown by electron microscopy in desert rodents, the cytological features of the magnocellular somata were clearly those of stimulated neurons (enlarged Golgi apparatus and rough endoplasmic reticulum) involved in hyperactive neurosecretion. In contrast to the rat, in which peptide stores are depleted under strong dehydrating stimuli [29,30], we found strong immunostaining for VP or OT in neurohypophysial axons. The axons were also filled with secretory granules, as seen with electron microscopy. Both observations indicate then that the storage of HNS peptides is great in the neural lobes of both Psammomys and Gerbillus, and are in accordance with previous results [5,7,31–33], demonstrating important stores of VP in the neural lobe of desert rodents, presumably necessary to allow rapid adaptation of such species to the strong dehydrating conditions of the desert environment. The AVP storage in the hypothalamus is also increased, making it possible to restore pituitary AVP pools, thereby replacing the AVP released into the blood during long-term water restriction.
Earlier studies showed that plasma VP concentrations were higher in desert rodents than in laboratory rats [6,33]. In our study, we detected high immunoreactivity not only for VP but for OT as well, in the hypothalamus of the two desert rodents examined. This is not too surprising in view of the fact that OT also contributes to hydromineral regulation, as earlier described under osmotic stimulation or chronic dehydration [34,35]. Previous reports suggested that OT can bind to the V2 receptor in the kidney, thereby stimulating levels of aquaporin 2 and inducing antidiuresis [36]. Such activation of V2 receptors by OT then could contribute to water conservation in these species.
Another novel finding of this study was that we detected numerous HNS neurons that displayed both VP and OT immunoreactivities. As in the dehydrated rat [24], immunocytochemical colocalization of the two peptides was observed in SON and mainly in its retrochiasmatic part and in PVN of Psammomys and Gerbillus. Colocalization was noted not only in neuronal somata but in axons as well, indicating simultaneous transport of both peptides to the neurohypophysis. Whether OT neurons are recruited into VP expression upon prolonged dehydration challenge to compensate the deficit of VP, or whether VP neurons begin to express OT under such strong conditions of stimulation remains to be determined.
4.2 The HNS of desert rodents is “permanently” reorganized
Since the HNS in the both desert rodents, P. obesus and G. tarabuli shows many features of increased neurosecretion, it was not too unexpected to find that its neuronal-glial interrelationships are those characterizing the HNS of rodents like the rat and mouse, under various conditions of heightened HNS secretion [1]. In particular, the SON of Psammomys and Gerbillus displayed a morphology similar to that of dehydrated rats [37] and mice [9]. With electron microscopy, we noted that in both desert rodents, the surfaces of many neurosecretory neurons were directly juxtaposed, without glial interposition, at the level of somata and dendrites. The reorganization of the system was also accompanied by synaptic changes, particularly well illustrated by the presence of numerous ‘multiple’ synapses coupling the neurosecretory neurons [1,38]. Such synapses were also a common feature of the magnocellular nuclei of the desert rodents.
5 Immunodetection of cell adhesion glycoproteins linked to structural plasticity
Observations from several laboratories clearly demonstrated that PSA-NCAM continues to be detected in systems like the HNS which undergo dynamic cellular changes [13,39]. As described in the rat [12], in both desert rodents we found high presence of PSA-NCAM throughout the HNS. Earlier studies using specific enzymatic removal of PSA from NCAM [10,11] established that it is the carbohydrate PSA which exists on the extracellular domain of NCAM that is essential to allow the neuronal and glial remodeling. In the desert species, the presence of the glycoprotein ensures that neuronal and glial changes can occur whenever necessary.
6 Conclusion
Our study has revealed that the HNS of desert rodents, P. obesus and G. tarabuli possesses many morphological properties linked to activated neurosecretion, including the presence of cell adhesion molecules necessary for neuronal and glial changes that accompany stimulated secretion. These species thus offer interesting models for further studies of neurosecretion under strenuous conditions of difficult habitats. They are also excellent models for further analysis of activity-dependent morphological plasticity since, even under natural conditions, they display the neuronal, glial and synaptic organization characteristic of the HNS under conditions of experimental and physiological stimulation.
Disclosure of interest
The authors declare that they have no conflicts of interest concerning this article.