1 Introduction
The osmotic stress is a powerful stimulus that has been associated to several peripheral and central manifestations in many species with alteration of numerous endocrine and neurotransmitter systems [1–4]. Circumventricular organs (CVO) has been meanly involved in the central response to osmotic stress such as the subfornical organ [5,6], area postrema (AP) [7], median preoptic nucleus (MnPO) [6], organum vasculosum of laminae terminalis (OVLT) [6] and the subcommissural organ (SCO) [2,8]. The latter is a derivative of the ependymal layer in the central nervous system of chordates [9,10] that releases doubly, in blood stream and cerebrospinal fluid (CSF), a glycoprotein with high molecular weight known after Reissner; Reissner's fiber (RF) [11] which has been involved in many physiological functions including its role in the clearance of monoamines [12], detoxification of the CSF [13,14], neuronal survival [15], the ontogenetic development of the spinal cord and the posterior commissure [16–19], the pathophysiology of lordosis [20], hydrocephalus [21] and its mean role in the control of water balance [2].
The SCO is a target of numerous innervations [22] namely GABAergic [23,24], noradrenergic and dopaminergic [25] and serotoninergic fibers [26,27]. One of the most abundant innervating systems within the SCO is of a serotoninergic nature [28,29]. Substantial evidences have suggested that the serotoninergic system, which originates from the dorsal raphe nucleus (DRN) [30] which influence sodium appetite, food and water intake behaviors [31,32], controls the SCO secretory activity of cat, frog, lizards, and meriones [1,29,33,34].
Otherwise, involvement of the SCO in water balance is supported by several experiments, indeed, destruction of the SCO results in lower water consumption and a decreased urine output [35,36]. Furthermore, SCO-extracts have an antidiuretic effect in cat [37,38] and influence the level of urinary sodium and sodium excretion in dog [39]. A functional relationship between SCO and adrenals has also been proposed [40–46].
The above observations suggest involvement of the SCO in the control of body homeostasis, however, little is known about its response especially the SCO secretory activity in rat submitted to prolonged dehydration.
For that we aimed by the present investigation to assess changes of SCO secretory activity of rat under prolonged water deprivation concomitantly with its serotoninergic innervation. This study was performed via an immunohistochemical analysis using antiserums against RF and serotonin (5HT) respectively.
2 Material and methods
2.1 Animals
Our animals were housed at a constant room temperature (25 °C), with a 12 h dark-light cycle and free access to food to all studied groups. Animals were treated in compliance according to guidelines of the University of Cadi Ayyad, Marrakesh (Morocco). All procedures were conducted in conformity with approved institutional protocols and in accordance with the provisions for animal care and use in the Scientific Procedures on Living Animals Act 1986 (European Council Directive: 86/609 EEC). All efforts were made to minimize animal suffering. Concerning the experimental design, we used male Wistar rats divided into two groups: Group 1 of controls (n = 5, 283.33 ± 26.40 g) with free access to food and water.
Group 2: of dehydrated rats (n = 5, 268.33 ± 23.85 g) by water deprivation during 2 weeks. To prevent excessive dehydration of the experimental animals, relative air moisture was kept at 90%.
2.2 Immunohistochemistry
At the 14th day of treatment, all animals of each group were anaesthetized with sodium pentobarbital (40 mg/kg i.p.) and perfused transcardiacally with chilled physiological saline and paraformaldehyde (4%) (Janssen Chimicas) in phosphate buffered saline (PBS, 0.1 M, pH 7.4). Brains were removed and fixed in the same fixative for 12 h at 4 °C, then dehydrated through a graded ethanol series (70–100%), passed through serial polyethylene glycol (PEG) solutions and embedded in pure PEG. 20 μm thick coronal sections were cut with a microtome according to the Bregma atlas and collected in phosphate buffered saline (PBS). Sections were taken from throughout the SCO and DRN. Free-floating sections were incubated in rabbit polyclonal anti-5HT antibody (Dakos), diluted 1/500, or in rabbit polyclonal anti-RF antibody [47], diluted 1/1000, for 48 h. Sections were then incubated for 2 h in biotinyled goat anti-rabbit immunoglobulin (Jackson Immunoresearch), diluted 1/2000, and then in avidin-biotin complex (ABC) (Vectastain ABC kit, Vecor laboratories), diluted 1/2000, for 2 h. All dilutions were performed in PBS, thorough washes between stages were performed in PBS containing 0.1% Triton X-100 and all stages occurred at room temperature unless otherwise stated. After washing with Tris buffer (Sigma) 0.5 M, pH 7.5, the peroxidase activity was revealed by incubating sections in 0.03% DAB (3,3′ diaminobenzidine, Sigma) and 0.02% H2O2 in 0.05 M Tris buffer, pH 7.5. Sections were then collected, dehydrated through a graded ethanol series and mounted in Eukit (Sigma) for light microscopy. Controls were carried out by omitting the primary antibodies during the first incubation and then incubating the SCO sections with all secondary immunoreagents. Observations were made in the same level of the SCO to prevent possible variations of the immunoreactivity between the different parts of the studied structures.
2.3 Immunolabeling quantification and statistical analysis
The quantification of immunolabeling was performed according to the protocol published by Vilaplana and Lavialle [48]. Briefly, the digitization and storage of images were performed using a Zeiss-Axioskop 40 microscope equipped by a digital Canons camera. Images were digitized into 512/512 pixels with eight bits of grey resolution and were stored in TIFF format. Image processing and quantification were performed using Adobe Photoshop v.6.0. After transformation of each image to the binary mode, the percentages of black pixels were obtained using the image histogram option of Adobe Photoshops. This percentage corresponds to the 5HT or RF immunopositive areas. For controls and dehydrated animals, five sections were randomly chosen for the quantification of each immunoassay treatment. Results are expressed as mean ± SEM. The Student's t-test was used to analyze differences between groups. Differences were considered significant for P < 0.05.
3 Results
3.1 Water deprivation reduces secretory activity of the rat SCO
After application of antiserum against the glycoprotein RF in a frontal sections through the SCO, our data show, in control rats (Fig. 1A), an heterogeneous immunostaining in all components of SCO; namely the apical and basal parts. Ependymocytes as well as the hypendymocytes of the SCO appeared strongly immunoreactive indicating an increase of the RF material ready for release doubly in blood and CSF circulations (Fig. 1A a1–a2), while in the rest of the organ, the immunostaining is weak. Dehydration for 2 weeks caused a significant overall decrease of RF immunoreactivity in both of the apical (Fig. 1Bb1) and basal (Fig. 1Bb2) parts of the SCO. Such decrease of RF immunoreactivity was confirmed by the statistical analysis (Fig. 1E).
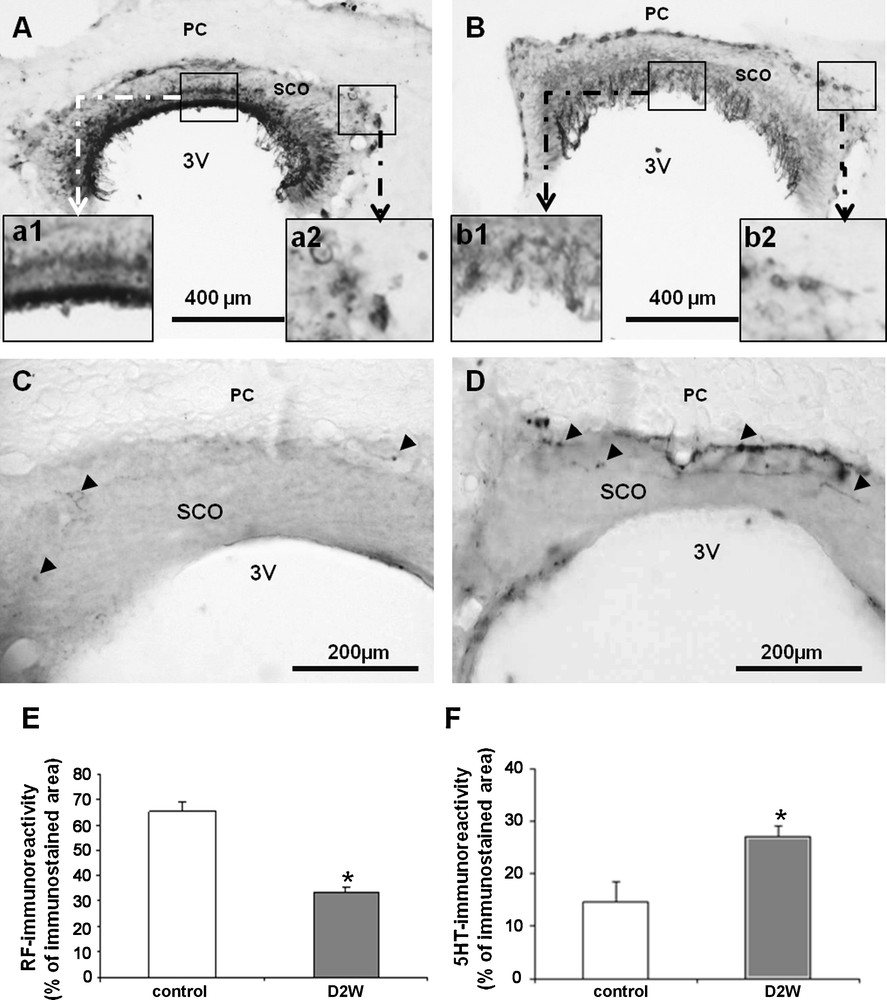
Light micrographs of frontal sections through the subcommissural organ (SCO) of adult rat, immunolabelled with antiserum against Reissner's Fiber (RF) (A and B); and against serotonin (C and D) in controls (A and C) and after 2 weeks of water deprivation (B and D). High magnification shows detail of immunoreactive ependymal (a1 and b1) and hypendymal (a2 and b2) cells. Histograms (E and F) showing the percentage of immunoreactive area to Reissner's fibers (E) and serotonin (F) in the two studied groups. Data are reported as mean ± SEM, and were subjected to Student t-test. A value of P < 0.05 was considered to indicate statistical significance between controls and dehydrated groups. PC: posterior commissure, 3V: third ventricle.
3.2 Water deprivation affects the serotoninergic innervation of the rat SCO
Substantial evidences support a serotoninergic control upon the secretory activity of the SCO in rat. Thus, to assess whether changes of RF material release by the SCO ependymocytes occurring in dehydrated rats is due to alterations of the serotoninergic outputs achieving the SCO, we undertook an immunohistochemical study of 5HT fibers within the SCO. Effectively, our findings demonstrated in control rats (Fig. 1C), many 5HT-immunoreactive fibers surrounding the ependymocytes in the basal and lateral parts and even within ependymocytes of the SCO. In dehydrated rats, an obvious enhancement in the density of 5HT-immunoreactive fibers is observed. These fibers form a dense and continuous plexus surrounding the basal and lateral parts of the SCO (Fig. 1D). Some immunopositive fibers appeared deeper within the ependymocytes of this organ. The statistical analysis supports such increase (Fig. 1F).
3.3 Water deprivation affects the 5HT immunoreactivity within the ventricular borders (VB)
To ascertain whether changes of serotoninergic innervation observed in the SCO occurring in dehydration are generalized to other brain regions, we examined in the VB of the third ventricle via immunohistochemistry the 5HT immunoreactivity, thus, in control animals; many 5HT immunopositive fibers innervated the ventricular borders and formed a highly reactive plexus (Fig. 2A). After dehydration, an obvious rise of the immunoreactivity was seen in the ependymal region with an increased density of IR fibers reaching the ependymal cells of the ventricular borders (Fig. 2B). Such increase was confirmed by the statistical analysis (Fig. 2F).
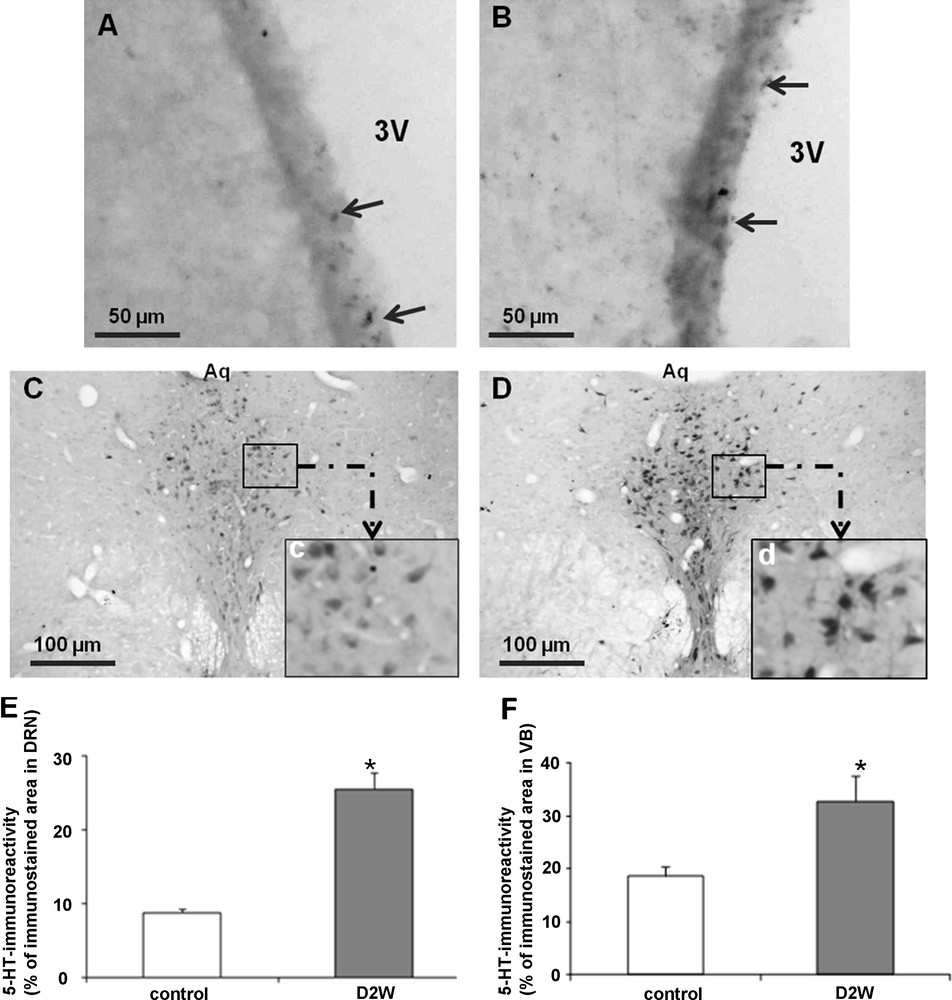
Light micrographs of frontal sections through borders of the third ventricle (VB) (A and B) and the dorsal raphe nucleus (DRN) (C and D) of adult rat, immunolabelled with antiserum against serotonin in controls (A and C) and after 2 weeks of water deprivation (B and D). High magnification shows detail of immunoreactive neurons in the DRN (c and d). Histograms (E and F) represent the percentage areas of immunoreactivity of serotonin in the DRN (E) and the VB (F) in different groups. Data are reported as mean ± SEM, and were subjected to Student t-test. A value of P < 0.05 was considered to indicate statistical significance between controls and dehydrated groups. Aq: Aqueduc de Sylvius, PC: posterior commissure, 3V: third ventricle.
3.4 Water deprivation affects serotoninergic system within the nucleus of origin: dorsal raphe nucleus (DRN)
To assess whether the increased 5HT innervation of the SCO is due to an enhancement of 5HT expression in the neurons of origin, we examined immunohistochemically the expression of 5HT in the nucleus of origin: DRN. Indeed, our finding demonstrates in dehydrated animals a significant enhancement of the immunoreactivity in all neurons forming the DRN nucleus (Fig. 2D) as compared to controls (Fig. 2C), high magnification shows details of immunoreactive neurons (Fig. 2Cc, 2Dd). The statistical analysis supports such observation (Fig. 2E). The prolonged water deprivation does not affect 5HT neurons number within the DRN between treated groups (Fig. 3).
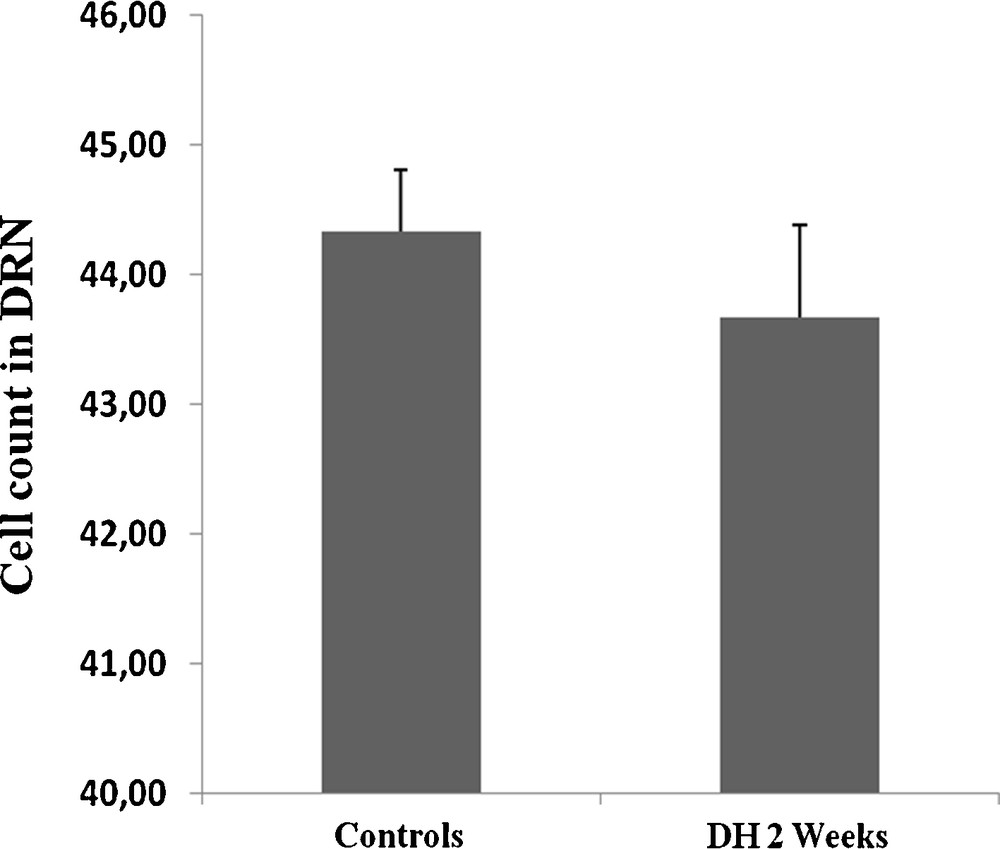
Histograms displaying the cell count in DRN of both control and dehydrated rat. Note that the prolonged water deprivation does not affect 5HT neurons number between treated groups (DH 2 weeks: dehydrated for 2 weeks).
4 Discussion
4.1 Effect of water deprivation on the secretory activity of the rat SCO
The SCO is a particular CVO that releases doubly in the CSF and the circulation [49] a glycoprotein with high molecular weight known after Reissner; Reissner's fiber [11]. This glycoprotein has been involved in many functions including neuronal survival [15], detoxification [13,14], transport of monoamines and clearance of the CSF [50], the pathophysiology of lordosis [20], hydrocephalus [21] and control of water and salt balance [2,8].
In our knowledge, to date no study has been performed concerning the effect of osmotic stress by prolonged water deprivation on the secretory activity of the SCO in the rat. The present study was aimed on the assessment of changes in the secretory activity and serotoninergic innervation of the SCO occurring during water deprivation in the rat. Indeed, our findings demonstrate after 2 weeks of total water restriction, a significant reduction of RF immunoreactivity within both of the hypendymal and the ependymal cells of the SCO. Substantial evidences have associated the changes of the SCO secretory activity with alteration of body homeostasis [1,2,8]. In the semi-desert rodent Meriones shawi, the secretory material of the SCO is significantly reduced following 1 and 3 months of total water restriction [2], whereas, in the frog Rana temporaria, submitted to osmotic stress, the growth rate of the RF and the secretory activity of the SCO have been increased [1].
According to our finding, the reduction of the RF immunoreactivity in the SCO may result from either a decreased RF biosynthesis and/or increased RF release by the SCO.
The mean role and the underlining mechanisms by which the SCO controls water and electrolytic homeostasis is not well established, some experiments are thought to involve this organ in a direct control of peripheral homeostasis. Indeed, destruction of the SCO induces a lower water consumption and a decreased urine output [35,36] and increases the affinity of the SCO-cells for Schiff's reagent (periodic acid-Schiff reaction) indicating either storage or excessive production of the secretory material [51]. Otherwise, dehydration also affects the ultrastructure of the SCO-cells; the dilated sacs and vacuoles of the endoplasmic reticulum, the membrane system of the Golgi complex and the increase in the number of ribosomes suggest a stimulation of the secretory process in the SCO of dehydrated animals [52]. Otherwise, SCO-extracts have an antidiuretic effect in cat [37,38] and influence the level of urinary sodium and sodium excretion in dog [39]. A functional relationship between SCO and adrenals has also been proposed [40,41,43–46]. The SCO may have a hormonal influence on the adrenal cortex by the production of an aldosteronotropic factor [43,53]. Vullings and Diederen in 1985 demonstrated that exposure of the SCO to aldosterone, known to be enhanced during dehydration [54], decreases the secretory activity of the SCO by means of a process of negative feedback regulation [1]. Also worth mentioning is the presence of angiotensin II (AAGII) receptors in the SCO-cells which display binding sites for angiotensin II [55] and, in some species, for atrial natriuretic peptide [56]. Indeed, some studies suggest a functional interrelation between AAGII and the secretory activity of the SCO of goat [57].
4.2 Effect of water deprivation on the serotoninergic innervation of the rat SCO
The SCO is a target of numerous innervations, namely GABAergic [23,24], noradrenergic and dopaminergic [25], and specially serotoninergic [26,27]. This latter innervation is well known to have a control on the SCO secretory activity of several species such as rat [28,58], Meriones [29], gerbil [58] and lizard [34]. Our results show a significant enhancement of the density of the 5HT-immunoreactive fibers achieving the SCO ependymocytes. Thus, the same tendency was observed in the DRN, the origin nucleus of this innervation, from which emanates the serotoninergic fibers innervating the SCO. Such increase of the 5HT innervations of the SCO seems supporting a possible decrease of RF biosynthesis rather than an increase of RF release by the SCO, since in rat, serotonin negatively controls the RF material release by the ependymocytes of the SCO [59]. In contrast to M. shawi, in which, serotonin stimulates the RF release by the SCO [29] that may explain in part, the specific differences of the SCO responsiveness to water deprivation between rat and M. shawi.
Involvement of serotonin in water intake behavior is well established [32,60]. In rat, deprivation of water for 5 days causes a selective decrease in the serotonin concentrations of the neural lobe and pars distalis [61]. In M. shawi, submitted to prolonged water deprivation, serotonin expression in the DRN, supraependymal plexus and the subcommissural organ decreased significantly [2]. While in adult soft-shelled turtles exposed to hyperosmotic stress either with chronic dehydration for 7 days or intramuscular injection of sodium chloride (3.6%, 0.5 mL daily for 7 days) or acute treatment for 0.5, 1 or 2 h with sodium chloride (3.6%, single intramuscular injection) reduced the pineal-paraphyseal serotonin [62]. Our data seem to be contradictory regarding the previous studies; such differences may result from the duration of water deprivation since the present work describes for the first time, in contrast to previous studies, the responsiveness to serotoninergic system to long period of dehydration in rat. Taken together, the above data demonstrate the implication of the serotoninergic system in water and electrolyte homeostasis. Indeed, further investigations have associated this system with the control of body homeostasis [63]. Some experiments of electrolytic or exitotoxic lesions of the DRN reduced the basal and stimulated plasma levels of the atrial natriuretic peptide [64], and increased the basal salt intake in several experimental models [32,65–67].
5 Conclusion
The present findings confirm the involvement of the SCO and the serotonin system in the response to water deprivation in the rat as demonstrated in the M. shawi [2]. The variation of the serotonin system after a prolonged dehydration induced both in the rat as in the Meriones, a decreased secretion of the RF. The mechanism for the involvement of the latter in the dehydration remains to be determined.
Disclosure of interest
The authors declare that they have no conflicts of interest concerning this article.
Acknowledgment
This work has been supported by the Moroccan CNRST (SC. Vie 05/10), le Centre national d’études et de recherches sur le Sahara (CNERS); OTAN (PDD [CP] - [LST.CLG979823]).