1 Introduction
A sex-specific pattern of individual growth, resulting in sexual size dimorphism (SSD), is a life history aspect of adaptive importance [1–4]. Our understanding of the evolutionary mechanisms that were responsible for the development of SSD in the ontogeny has come through the study of individual growth patterns [5,6]. An optimal growth pattern is achieved by the trade-off between the benefits of fast development and the costs of high growth rate [7].
Solitary aculeate Hymenoptera serve as an appropriate model group to study growth patterns and SSD formation, as their females store food for their offspring in individual brood cells; the amount of cell provision is sex-specific and predetermines the body size of adults of each sex [8–14].
The actual amount of food stored for each individual offspring and the parental provisioning effort can be precisely measured, thus aculeate Hymenoptera are used in studies on parental investment, sex allocation and sex ratios, prey handling effort and other evolutionary trade-offs [1,15–22].
Female-biased SSD, i.e. females being larger than males, is typical of solitary wasps [23,24]. There are three non-exclusive ways to achieve SSD in the ontogeny [25]. The simplest way is protandry, i.e. earlier male emergence due to shorter development, ensuring a mating advantage at the cost of a smaller size [26–29].
The second possibility is through a smaller size of eggs and hatching larvae which will develop into the smaller sex. This could be explained as a trade-off between the quality and quantity of offspring: e.g. if they are still large enough for successful mating, a wasp will have an advantage producing a higher number of smaller males. This explanation is plausible for organisms which are able to determine the brood sex, e.g. Hymenoptera with their single-locus complementary sex determination [30].
The third possible means for the SSD formation is a higher growth rate of the larger sex [25,31]. In the mass-provisioning solitary wasps, the adaptive benefit of a faster growth and shorter feeding period may be associated with the minimizing of losses of the provision, this occurring due to some of the paralyzed prey specimens eventually dying and drying out or becoming infected by fungi and accumulating toxic compounds, as has been supposed for the solitary wasp Philanthus triangulum [11]. In the case of larval prey, they may also develop into pupa or even imago, thus becoming inedible for the wasp larva [22]. Thus, loss of quality in the provision, affecting the larval development as a temporal constraint, may provide a higher selection pressure on the larger sex, which is supplied with a greater amount of prey, resulting in a higher rate of food consumption and growth of the female larva.
Blanckenhorn et al. [25] found that in four out of seven studied arthropod taxa with female-biased SSD, the development time of males is equal to or even longer than that of females, implying a higher growth rate of females. However, in the cases where protandry has a selective advantage, as in some Araneae, Lepidoptera and Hymenoptera, the development time of males may be shorter than that of females. The authors concluded that the difference between the growth rates in sexes is more important in SSD formation than the difference in development time. They did not consider the third possibility of SSD formation, i.e. size differences betweeen the hatching larva of differing sexes, because there was no evidence of size differences in the eggs developing into different sexes among the arthropods.
The goal of our study was an assessment of the relative importance of the three ways of SSD formation in the ontogeny of solitary wasps. We focused on the development of the cavity-nesting solitary wasp Symmorphus allobrogus Saussure (Hymenoptera: Vespidae). The species is protandrous and has a well-expressed SSD: the adult male/female average body weight ratio is 0.62 [32]. We tested three hypotheses on the origin of female-biased SSD in the ontogeny:
- • the female larva develops from a larger egg than the male larva does, thus the female has a larger size from the very start of its development and maintains the size difference during the whole ontogeny;
- • since the female larva is supplied with a larger quantity of provision, all other conditions being equal, it finishes consumption of food and growth later than the male larva, thus reaching a larger size due to a longer development;
- • the female larva becomes larger because it has a higher growth rate than the male larva due to faster and/or more efficient food assimilation.
2 Material and methods
2.1 Study organism
S. allobrogus is a univoltine gregarious cavity-nesting (xylicolous) solitary predatory wasp species, which is common in synanthropic environments in Lithuania. After emergence and mating during late May–early June, females start building their nests in pre-existing tubular cavities, e.g. xylophagous beetle exit holes, in wooden parts of buildings. A nest of S. allobrogus consists of a row of brood cells, built starting with the deepest one and separated by mud diaphragms [33]. The egg is attached to the upper internal surface of the brood cell and, after the oviposition, the mother wasp supplies the cell with 3–16 specimens of paralyzed Chrysomelinae leaf beetle larvae [20,34]. Usually the wasp oviposits the fertilized eggs developing into females in the innermost brood cells of the nest and the unfertilized male eggs in the cells closer to the entrance of the nesting cavity.
2.2 Collection and rearing
Wasp nests for the study were obtained using bundles of internode fragments of dry reed (Phagmites australis) stems as trap-nests. The latter were placed on the walls of old wooden or wattle and daub buildings with natural colonies of solitary aculeates in the localities of Varnupys (55°24′N 25°17′E), Bilšiai (55°08′N 25°16′E) and Papiškiai (55°56′N 24°16′E), Lithuania. Reed internodes with newly finished wasp nests were removed from the trap-nests every 7 days and taken to the laboratory. The nests were immediately opened and studied. Most of the studied wasp nests were collected in 1999–2006, with some additional data obtained in 2009–2011.
We used data from brood cells which contained an egg or a larva of the first or the second instar at the moment of nest dissection. Further observation of the brood cells containing an egg at the time of nest dissection demonstrated that the development of the first and the second larval instars always took less than one day. The larvae observed at the moment of hatching usually reached late second or sometimes early third instar in one day. Thus, for the brood cells containing a larva of the first or the second instar at the moment of nest dissection, the time of hatching was considered to be approximately one day before the dissection.
After the study, the larvae were placed back into the nest cells together with their provision, where they continued feeding and growth and developed to diapausing prepupae. The latter were placed individually into the wells of 96-well plastic plates. To stimulate the termination of diapause, they were kept in a refrigerator at 4 °C for 3–5 months. After the termination of diapause, the development to an adult at an ambient temperature (22–26 °C) continued for 13 to 20 days. The adult insects were kept in plastic containers with provided honey solution and pure water for 2–4 weeks.
2.3 Data on development
In the nests with brood cells where the wasp larvae had not yet hatched, we measured the length and width of the eggs. The egg volume was calculated from length and width, considering the approximate form of it as a cylinder with hemispherical ends. The provision in the brood cells containing an egg or a larva of the first or the second instar not older than approximately one day was weighed, the weight of larva being added to the weight of provision in the latter cases. Afterwards, the body weight and head width of the developing larva were measured daily, or in some cases, due to technical reasons, at intervals of two days, until the start of the spinning of the cocoon. As the larvae were temporarily removed from their prey, we suppose that daily measurements could have a weak negative effect on the growth rate and the duration of larval development. However, we consider this negative effect as unavoidable, relatively weak (the larvae were disturbed for 10–15 minutes per day) and approximately equal/random in relation to the studied larvae and the sexes. Therefore, we consider our results on larval growth as fully comparable within the obtained dataset of this study.
The prepupae (fifth instar larva after cocoon spinning and defecation) were weighed before and after the diapause. We also measured the body weight of pupae 1–2 days after emergence from the prepupa, the body weight of active adults after defecation and regurgitation of liquid from the gut or crop (in S. allobrogus, these phenomena occurred after 2–3 days of passive phase following the eclosion from the pupa) and the body weight of the adults again after 5–7 days of keeping them with available food (honey solution) and water.
2.4 Statistical analysis
Statistical analysis was performed using the computer program Statsoft STATISTICA, release 8. The differences between measurements of male and female were assessed using one-way Anova. The dependences on body weight and on the weight of provision were evaluated using Pearson linear correlation.
To estimate the growth rate, we grouped the weight measurements and weighing times in subsequent pairs, correspondingly W1 and W2 (in mg, to a precision of 0.1 mg), and t1 and t2 (in days, to an accuracy of the nearest minute). The relative growth rate (RGR) was calculated according to the formula RGR = (lnW2–lnW1)/(t2–t1) [35]. To approximately estimate the growth parameters r and b, the exponential growth function W(t) = W0ert was log-transformed to ln(W) = ln(W0) + rt; the simplified allometric growth function dW/dt = kWb [35] was log-transformed to ln((W2–W1)/(t2–t1)) = ln(k) + b ln(W1); the equations were solved using linear regression and pooled empirical data of each sex.
3 Results
3.1 Sex differences in egg size
Eggs developing into females were longer (2.25 ± 0.03 mm) and wider (0.73 ± 0.01 mm, n = 28) than eggs developing into males (correspondingly 2.15 ± 0.03 mm and 0.69 ± 0.01, n = 34; here and hereafter mean ± SE); the difference was significant (here and hereafter Anova: correspondingly F = 5.0, P = 0.03 and F = 6.3, P = 0.02). The estimated volume of male eggs had a wider range than that of female eggs, the mean volume of the latter being larger by 16.3% (F = 7.3, P = 0.009) (Fig. 1). Consequently, the freshly hatched female larvae were on average larger than the male larvae. The size difference between sexes at hatching thereafter continued to increase, the difference as measured by the width of head capsula (Table 1) and the body weight (Fig. 2) increasing constantly, albeit weakly, from the first to the final fifth instar of larva.
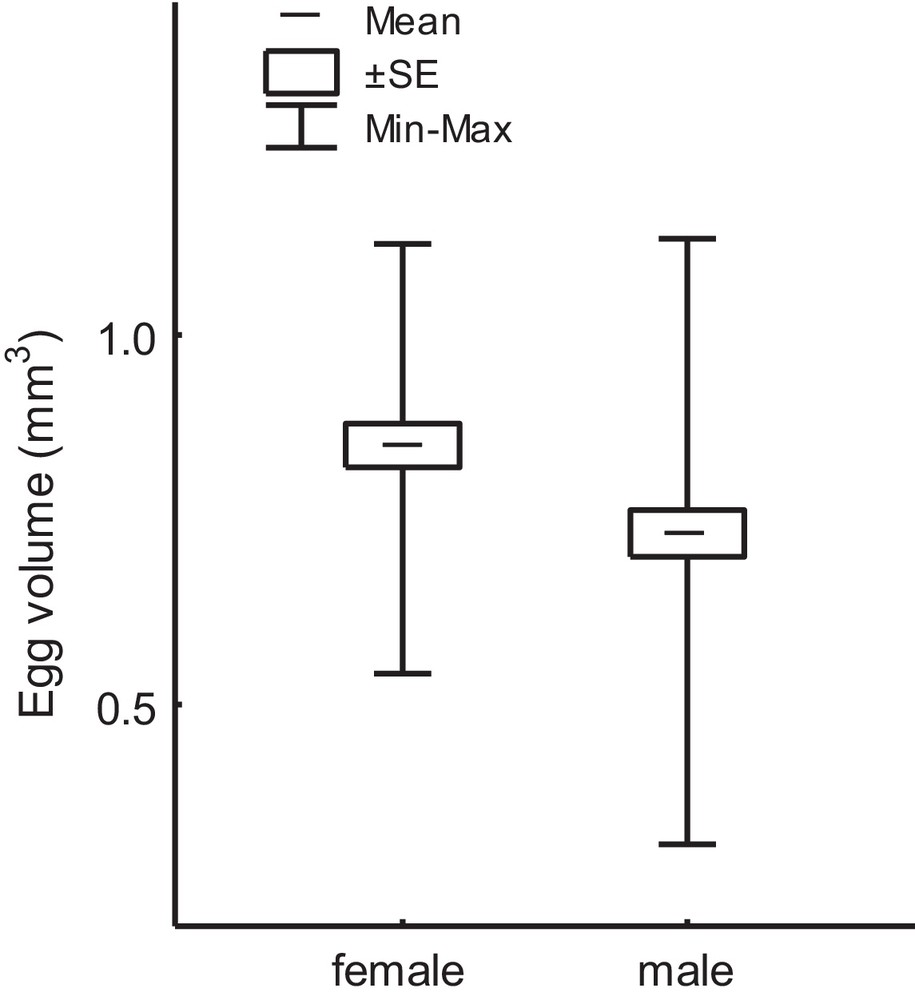
Volume of eggs of S. allobrogus, developing into females and males.
Width of the larval head, the SSD (male/female ratio of the average head width) and the significance of difference in the head width between sexes using Anova in S. allobrogus.
Instar | Head width, mm (mean ± SE) | SSD | Difference between sexes (Anova) | ||||
Female | n | Male | n | F | P | ||
1 | 0.573 ± 0.005 | 36 | 0.551 ± 0.006 | 26 | 0.962 | 8.6 | 0.005 |
2 | 0.683 ± 0.004 | 66 | 0.658 ± 0.006 | 37 | 0.963 | 14.6 | 0.0002 |
3 | 0.869 ± 0.003 | 107 | 0.819 ± 0.005 | 69 | 0.942 | 71.9 | 0.0000 |
4 | 1.114 ± 0.002 | 203 | 1.045 ± 0.005 | 114 | 0.938 | 206 | 0.0000 |
5 | 1.333 ± 0.002 | 228 | 1.237 ± 0.005 | 140 | 0.928 | 400 | 0.0000 |

Growth of larval body weight from hatching to cocoon spinning in the two sexes of S. allobrogus.
3.2 Time scale and sex differences in the duration of larval development
Observation of brood cells in a number of fresh nests collected on the day of their completion revealed that the duration of egg development of S. allobrogus was approximately three days. The larvae passed the first and reached the second or sometimes even the third instar within approximately one day. Therefore, as growth measurements of larva were only taken once a day, we often missed the first larval instar and thus did not consider it separately from the second larval instar. On average, the duration of larval development of S. allobrogus from the first encounter of the first or second instar till the first encounter of the last fifth instar was approximately equal to three days (Table 2). The growth of the fifth instar until the start of cocoon spinning lasted another three days. Thus, the larvae finished consuming their provision, stopped growing and started to spin their cocoons typically at the age of seven days (Fig. 2). On the sixth day after the start of spinning, the larvae finished the cocoon formation and then entered a one-day period of final defecation. Afterwards they entered the phase of diapausing prepupa. Consequently, the duration of all phases of the postembryonic development from hatching to diapausing prepupa was approximately equal to 13 days.
The time scale of larval development of S. allobrogus and the significance of differences between sexes using Anova.
Instar | Period from hatching to the first encounter of the instar, days (mean ± SE) | Difference between sexes (Anova) | ||||
Female | n | Male | n | F | P | |
2 | 1.03 ± 0.05 | 13 | 0.94 ± 0.07 | 6 | 1.1 | 0.31 |
3 | 1.50 ± 0.09 | 30 | 1.24 ± 0.10 | 19 | 3.6 | 0.06 |
4 | 2.45 ± 0.10 | 34 | 2.32 ± 0.12 | 20 | 0.7 | 0.41 |
5, feeding | 3.94 ± 0.13 | 35 | 3.87 ± 0.17 | 20 | 0.1 | 0.76 |
5, cocoon spinning | 6.88 ± 0.22 | 24 | 6.90 ± 0.35 | 12 | 0.01 | 0.95 |
5, final defecation | 12.31 ± 0.36 | 43 | 12.30 ± 0.53 | 24 | 0.01 | 0.91 |
Brood cells of S. allobrogus were supplied with sex-specific amount of provision: the cells with female larvae contained 139.4 ± 1.9 mg (n = 102), the cells with male larvae contained 101.4 ± 2.0 mg (n = 46) of paralyzed prey (F = 100, P = 0.0000). With this difference, one would expect the consumption and development time of female and male larvae to be different. In fact, the time period between hatching and the first encounter of larva of the second, the third, the fourth and the fifth instar was on average longer in females than in males, implying that the instar development duration of female larvae of the penultimate 2nd–4th instars was longer than that of male larvae. However, with the available number of observations, the time difference was statistically not significant, particularly at the stages of development of the final instar of larva (Table 2). It is apparent, despite the substantially differing quantities of the consumed provision, the duration of larval development of the two sexes from hatching until diapausing prepupa was approximately equal.
3.3 Sex differences in larval growth rate
During the period of feeding, the S. allobrogus larvae grew nearly exponentially: the estimated exponent b of the allometric growth function dW/dt = kWb was 0.90 ± 0.03 in females and 0.91 ± 0.05 in males. The estimated growth constant r of the exponential growth function W(t) = W0ert was 0.64 ± 0.02 in females and 0.63 ± 0.03 in males (Fig. 2), corresponding to an increase in weight of approximately 1.9 times a day.
Female larvae had a significantly higher average relative growth rate (RGR) (0.853 ± 0.012, n = 390) than male larvae (0.765 ± 0.016, n = 239; F = 19.1, P = 0.00001). The RGR of the larvae had a weak sigmoid change during their development, approximately described by cubic polynomial functions (Fig. 3). It appeared that the young larvae grew faster while feeding on their first prey specimen. Afterwards, the efforts of still relatively small larvae to switch to other prey specimens of the provision negatively affected their growth rate. For instance, the mean RGR of female larvae with W1 < 7 mg was 0.91 ± 0.02 (n = 152), while the mean RGR of female larvae with W1 ≥ 7 mg was 0.82 ± 0.01 (n = 238; F = 13.4, P = 0.0003); the mean RGR of male larvae of these weight groups was correspondingly 0.80 ± 0.02 (n = 118) and 0.73 ± 0.02 (n = 121; F = 5.6, P = 0.02). The larvae of the last instar appeared to increase their growth rate: e.g. female larvae with W1 ≥ 18 mg had mean RGR 0.93 ± 0.03 (n = 32); male larvae with W1 ≥ 14 mg had mean RGR 0.84 ± 0.04 (n = 27). At the end of the feeding period, the relative growth rate of the larvae seemed to decrease again (Fig. 3).

The change in the relative growth rate (RGR) of larvae of S. allobrogus in relation to their weight.
The difference of growth rate between the sexes was higher if comparing young larvae: the mean RGR of larvae with W1 < 10 mg was 0.87 ± 0.02 (n = 221) in females, but 0.76 ± 0.02 (n = 177) in males (F = 18.9, P = 0.00002). The RGR of the larger larvae (W1 > 10 mg) had a lesser difference: 0.83 ± 0.02 (n = 169) in females and 0.78 ± 0.03 (n = 62) in males (F = 1.8, P = 0.18).
The weight of a full-grown female larva comprised 68.4 ± 0.9% of the weight of provision (n = 102), while the weight of a full-grown male larva was 62.4 ± 1.5% of the weight of provision (n = 46). The weight of the diapausing prepupa after cocoon spinning and final defecation comprised 35.8 ± 0.5% of the provision weight in females (n = 88) and 30.9 ± 0.8% in males (n = 34). In both cases the difference between sexes was significant (correspondingly, F = 13.1, P = 0.0004, and F = 26.5, P = 0.0000). Thus, the female larva assimilated food more efficiently. This efficiency was not related to a larger body size or a larger amount of provision of the female larva per ce: the ratio of weight of female prepupa to the weight of the consumed provision negatively correlated with the weight of the provision itself (r = −0.24, P = 0.02; this correlation was not significant in males), i.e. the assimilation of a too large amount of provision was relatively less efficient.
3.4 Sex differences in weight loss from full-grown larva to imago
After consumption of the provision and before the start of cocoon spinning, the fifth instar larvae reached their greatest weight, 94 ± 1 mg in females (n = 144) and 63 ± 2 mg in males (n = 63; F = 170, P = 0.0000). The subsequent ontogenetic stages demonstrated weight loss until they emerged as active imago (Fig. 4).
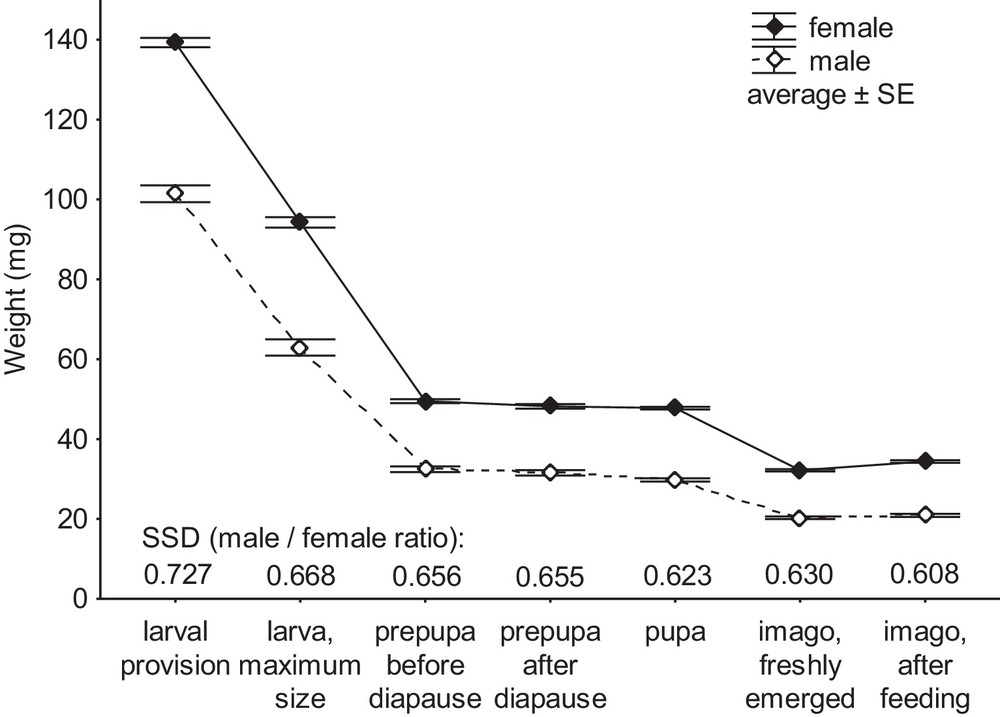
Weight of larval provision and the main developmental stages of the two sexes and the SSD (male/female weight ratio) of S. allobrogus.
The weight of prepupa comprised 52.1 ± 0.5% of the weight of a full-grown larva in females (n = 122) and 48.8 ± 0.8% in males (n = 46; F = 12.9, P = 0.0004). In females, the ratio of the prepupal weight to the weight of the full-grown larva significantly negatively correlated with the weight of that full-grown larva (r = −0.30, P = 0.0007), i.e. the largest larvae lost relatively more weight during cocoon spinning and excretion of the meconium than smaller larvae. This correlation was not significant in males.
The loss of body weight during the prepupal diapause and the subsequent development of pupa was relatively low (Fig. 4). On average, the weight of a female prepupa after hibernation comprised 97.0 ± 0.2% (n = 210) of weight of the prepupa before hibernation. In males the loss was slightly lower: after hibernation the weight of prepupa was 97.6 ± 0.2% of the weight before it (n = 117; F = 5.0, P = 0.025). This weight loss did not correlate with the body size in any sex.
The weight of fresh (white) pupa comprised 95.1 ± 0.2% of the weight of the prepupa after hibernation in females and 95.0 ± 0.3% in males; the difference in weight loss between the sexes during the turning to pupa was not significant and it was not affected by the body size.
Emerging adult wasps were passive for 2–3 days. Afterwards they excreted white faeces, presumably rich in uric acid, and a relatively high amount of liquid from their gut or crop, which was regurgitated and used to soften the mud of the diaphragms and plug of their nest in order to exit. The weight of a freshly emerged active adult after the defecation and the regurgitation was 67.9 ± 0.3% of the pupal weight in females (n = 446) and 68.4 ± 0.5% in males (n = 228; F = 1.1, P = 0.3). The ratio of the weight of the active adult to the weight of the fresh pupa positively correlated with the pupal body weight in females (r = 0.19, P = 0.00004), i.e. the relative loss of weight at the eclosion was lower in the larger females. There was no such correlation in males.
Additional feeding in captivity did not significantly change the body weight of males during the next 5–7 days: the latter comprised 99.8 ± 1.0% of the weight at the eclosion (n = 170). In contrast, the body weight of females increased after the feeding, comprising 103.1 ± 0.6% of the weight at the eclosion (n = 330); the difference between sexes was significant (F = 9.1, P = 0.003). The relative increase in weight negatively correlated with absolute body size at the eclosion (r = −0.39, p = 0.0000 in females; r = −0.21, P = 0.006 in males), i.e. smaller wasps gained relatively more weight after feeding than the larger ones.
4 Discussion
4.1 Egg size
To our knowledge, no studies of insect eggs have found size differences in eggs that develop into the different sexes [25,31,36]. Similar male and female egg size has been reported in several studies of protandrous insect species, including solitary Hymenoptera [11,37–39].
In S. allobrogus, the maximum size of eggs developing into males and females was approximately equal, but the minimum size of eggs developing into males was remarkably smaller. These data imply that the females of S. allobrogus tend to oviposit the smaller eggs without fertilization. As a result, hatching larvae of males are on average smaller than hatching larvae of females. Thus, our results support the tested hypothesis (1) that the SSD may be caused at least in part by a difference in the initial body size of larval males and females.
4.2 Duration of larval development
Despite the larger amount of provision of female larvae than of male larvae, the duration of larval feeding of the two sexes of S. allobrogus did not demonstrate significant differences (Table 2). Thus, the hypothesis (2) presuming a longer growth period of the larger sex due to a larger amount of consumed provision is not supported, at least for the available set of observations. Consequently, the data on larval development of S. allobrogus corroborate the statement of Blanckenhorn et al. [25] that the SSD of Hymenoptera is only weakly mediated by the difference between sexes in the duration of larval development, and an earlier eclosion of males in protandrous species occurs due to a shorter development of prepupa and pupa after the diapause. A weak effect of difference between sexes in the duration of development on the formation of SSD has also been found in some other protandrous insects, e.g. in Coccinellidae [28,39].
4.3 Growth rate
Larval growth close to exponential, as that in S. allobrogus (Fig. 3), has been observed in other insects [40,41], although it has been found that the larvae of the last instar may have a slower growth [35]. Sexual differences in the growth rate are however sparcely documented [31].
Our data demonstrate that female larvae of the studied wasp species grow considerably faster than the male larvae, particularly if we compare the young larval instars feeding on their first prey specimen. A young larva has low mobility and weakly developed senses, thus the higher growth rate of female larvae cannot be explained by a wider possibility of best prey choice from the larger amount of provision. The most probable explanation of the higher growth rate is more efficient food assimilation and ability to gain more weight from a provision unit by female larvae. The results of our study support the hypothesis (3) presuming that a faster growth of the larger sex is the main reason of the SSD formation.
A higher food conversion to the body mass of female offspring is also known in other Hymenoptera [1,12,18,42–44]. Studies with resource manipulation are particularly convincing: provided with the same amount of food, females grow larger and heavier than males in Panorpa cognata [45], Brachinus explodens [46], Euodynerus foraminatus [47], P. triangulum [11,48].
Concerning the lower growth rate of male larvae, their growth may be determined by sex-specific resource allocation. For example, male larvae allocate more resources than female larvae to their fat reserves in P. triangulum [11] or to gonads in Adalia bipunctata [39]. According to another presumption, males may have a higher metabolic conversion rate per unit of weight due to a smaller size, as it has been found in ants [49,50] and solitary bee Osmia cornuta [12].
4.4 Weight loss
Male larvae of S. allobrogus lost more weight during the cocoon spinning and defecation than female larvae. In contrast, the female larvae lost slightly more weight during the diapause. The latter phenomenon may be explained by the suggestion of Fischer et al. [51] that a higher weight loss during the development of protandrous males may comprise a physiological cost of a faster growth. The male-biased weight loss during the cocoon preparation observed in our study could be caused not only by a higher metabolic rate of smaller males, but also by the investment of a greater proportion of their larval resources into the cocoon, as it has been reported for solitary bees O. cornuta [12] and O. ribifloris [44]. This investment may have an adaptive value in the xylicolous solitary Hymenoptera species building linear nests, such as Osmia and Symmorphus, due to a higher vulnerability of brood cells with male offspring. The latter are placed in the external part of nest, thus they are more susceptible to predators and parasitoids than the female offspring cells, which are placed in the internal, deeper part of the nest.
The weight loss of diapausing prepupae of S. allobrogus during the hibernation, as well as during the ecdysis to a pupa, was relatively low. In contrast, the diapausing adults of the cavity-nesting solitary bees O. cornuta and O. lignaria markedly lost body weight and depleted their fat [52,53].
The weight loss at the eclosion of an adult was approximately equal in both sexes of S. allobrogus, although other insects may demonstrate sexual differences at this stage of development. For example, females of Lepidoptera typically lose less weight upon eclosion than males [54].
Finally, a significant increase in body weight in adult female wasps during the first week after the eclosion implies a necessity of additional feeding for the formation of eggs, which apparently is not needed for male wasps.
Comparing the ratio of the estimated mean volume of the eggs developing to male and female (0.86), we may conclude that the SSD of S. allobrogus firstly occurs at the stage of egg fertilisation in the body of the mother wasp. Afterwards it increases during the entire postembryonic ontogeny, as is demonstrated by the ratio of the mean body weight of the male to that of the female at the last larval instar (0.67), the prepupa (0.66), the pupa (0.62), the freshly emerged adult (0.63) and the adult after the additional feeding (0.61). Early SSD formation is in line with the studies of several lepidopteran species, where the body size of the sexes diverges relatively early, and this difference increases during the larval development [36]. In the predatory wasp S. allobrogus, the SSD is mediated by a combination of a larger starting size and a higher growth rate of female larva, in comparison to male larva; the difference in duration of larval development has no or low significance. The generality of these findings needs to be tested across other species of solitary wasps.
Disclosure of interest
The authors have not supplied their declaration of conflict of interest.
Acknowledgements
This study was partly supported by a grant from the Research Council of Lithuania (contract No. MIP-033/2011) and by the EU Framework Programme 7 Project SCALES (Securing the Conservation of Biodiversity across Administrative Levels and Spatial, Temporal and Ecological Scales) (contract No. 226852). Authors are grateful to two anonymous reviewers for their criticism and useful suggestions, as well as to Jos Stratford for help in the drafting of the English language.