1 Introduction
The spatial distribution of some animals representing the same taxa can be influenced by their developmental stage [1] or results from their choice where to deliver offspring [2,3]. In both cases, predation avoidance is one of the drivers of heterogeneous spatial distribution [4]. Predation influences spatial distribution of animals by direct lethal effect, resulting in the extinction of population by elevated predation pressure in some habitats, and indirect non-lethal effect resulting in constrain preys to seek habitats of lowered predatory pressure. Predation, competition, resource limitation and unfavourable abiotic conditions can influence offspring survival [5]. Limnetic species of Cladocera are of obvious interest, because their representatives play a leading role in zooplankton composition, serving as important food objects for planktivorous fish and planktonic invertebrates, including cladoceran L. kindtii (Focke 1844) [6] and predacious cyclopoid copepods [7,8], and thus playing a major role in planktonic food web. The reproductive cycle of cladocerans consists in parthenogenesis (asexual reproduction) and gametogenesis (sexual reproduction). In lakes of temperate climate zone, they usually begin gametogenesis during late autumn, and reproduce this way for a short period of time in preparation for unfavourable environmental conditions associated with winter. For the most part of the year, cladocerans reproduce parthenogenetically. Parthenogenetic cladocerans are more vulnerable to the predation pressure of planktivorous fish that forage large and highly visible individuals [9] than that of non-ovigerous ones, because of bearing eggs or embryos marked by a visible yolk sac and dark pigmentation inside the brood chambers [10]. To minimize the risk of meeting a predator, cladocerans have evolved a variety of morphological, behavioural and life-history defences [11]. One such defines is dwelling in refuges, i.e. habitats that are non-typical places to live, but their structure provides the cladocerans with shelters protecting them against predatory pressure. Research studies on the impact of fish predation on the distribution of Cladocera in lakes have attributed to patches of vascular macrophytes and macroalgae growing in the littoral zone a special refuge role for limnetic cladocerans [12,13], thus stating the hypothesis that, in more dense and structurally complex habitats, predators can capture fewer prey [14]. However, evidence for the macrophytes’ positive refuge role in protecting cladocerans against fish predation overlaps with the antithetic shore-avoidance theory, which states that the limnetic species of Cladocera, when under predation pressure, avoid a lake's shore parts and aggregate in its central, deeper parts [15,16]. That results in their specific horizontal distribution. Vertically, distinct individuals segregate at different depths in the water column, corresponding to different combinations of biotic and abiotic characteristics [17,18]. The problem of spatial complexity on the relations zooplankton–planktivorous fish have been profoundly studied in shallow lakes. However, the results of these studies cannot be directly transferred to predation–prey interactions in deep lakes, because of different environmental conditions [19,20]. They cannot also be transferred to relations between invertebrate predators and their prey, as foraging of planktivorous fish is mainly directed by vision [21], whereas planktonic invertebrate predators detect their prey with mechanoreceptors or tactile direct contact [22,23].
Some studies have reported the role of biotic and abiotic factors in the parthenogenetic reproduction of cladocerans [24,25]. Many cladocerans are active swimmers that can cover large distances and can recognize a wide variety of visual and chemical cues [26,27]; thus they can actively search a habitat where they can maximize their fitness. That means that besides minimizing the predation risk they will search areas with favourable conditions like good temperature, sufficient oxygen, high food concentrations, and reduced competition. Evidences concerning density pattern of egg-bearing cladocerans and their response to selected environmental factors are scarce, and their spatial distribution has not been reported yet. The general hypotheses have been:
- • distribution of brood-bearing cladocerans is related to predation pressure and predatory type; the abundance of cladocerans vulnerable to visually hunting fish is higher in the littoral zone than the abundance of species vulnerable to mechanoreceptive cyclopoid or tactile L. kindtii;
- • some environmental variables, especially macrophyte biomass in the littoral zone and abiotic factors in the pelagic zone, co-determine with predation pressure, the horizontal and vertical distribution of brood-bearing cladocerans.
The object of this study were five species of limnetic Cladocera coexisting in Lake Piaseczno: Daphnia cucullata Sars, 1862, Daphnia longispina O. F. Müller, 1785, Bosmina longirostris (O. F. Müller, 1785), Bosmina coregoni Baird, 1857, and Diaphanosoma brachyurum (Liévin, 1848). Observations on the distribution of ovigerous females and their refuge choice in relation to some environmental factors can extend our knowledge on the nature of the behavioural mechanisms that have evolved in cladocerans.
2 Materials and methods
2.1 Study site
The study was conducted in mesotrophic Lake Piaseczno (51° 23′ 03′′ N, 23° 01′ 46′′ E), situated in eastern Poland. The lake area is 83.2 ha and its maximum depth reaches 38.8 m. The lake is dimictic, so in summer and winter, the temperature and oxygen stratification typical of the temperate zone occurs. The lake displays a low trophic status. The concentration of total nitrogen amounts to 0.3 mg·dm−3, whereas the concentration of total phosphorus does not exceed 0.1 mg·dm−3. The average primary production is about 11 mg chla·dm−3 [28] and Secchi disc visibility ranges between 4.5 m in summer and 6.5 in late autumn. The littoral zone reaches to 5–6 m of depth due to high water transparency.
Samples were collected in two transects similar in depth and vegetation structure, but different in distance from the shore to the deepest part of the lake. The transects were placed in the eastern (transect 1) and north-western (transect 2) parts of the lake, and samples were collected in sites corresponding to the near shore with common reed Phragmites australis (hereafter called NS); near the littoral (hereafter called NL); deep littoral with water milfoil Myriophyllum alterniflorum (hereafter called DL1); deep littoral with macroalgae Nitella flexilis in transect 1, Nitella flexilis and Chara fragilis in transect 2 (hereafter called DL2), sublittoral (hereafter called Su), near the pelagial (hereafter called NP) and the deep pelagic zone (hereafter called DP) (Fig. 1).
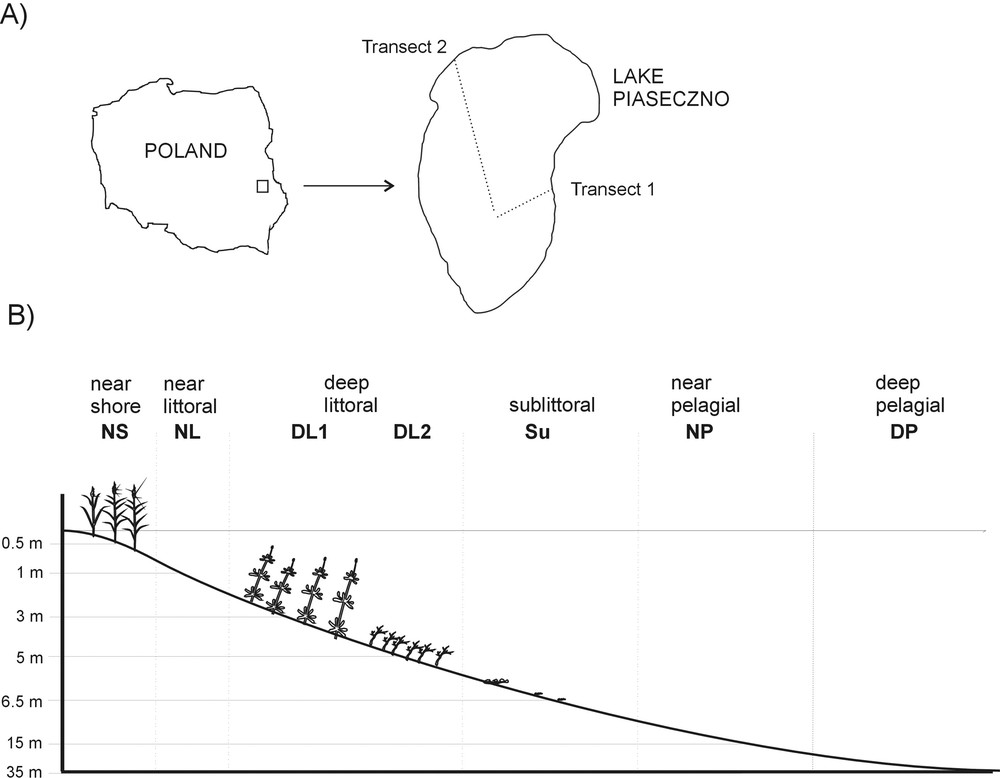
Location of the study area (A) and sampling sites (B).
2.2 Field and laboratory analyses
The densities of brood-bearing cladocerans were monitored monthly from April to November. Zooplankton was collected just about noon using a Plexiglas sampler. Samples 10 dm3 in volume were sieved through in a 40-μm mesh net and preserved with formalin treated with glycerine to prevent deformation of preserved organisms. In the laboratory, the cladocerans were counted (ind·m−3) under the microscope at 100× magnification with the use of a Sedgewick–Rafter counting cell. The proportion of adults in the population was estimated after determining the size at maturity [29] for each of the sampling times. Ovigerous females were counted to obtain the number (ind ·m−3) and proportion (%) of those egg-bearing to the total number of adult individuals. Simultaneously, predatory crustaceans potentially foraging on cladocerans (cyclopoid copepods M. leuckartii (Claus, 1857), T. oithonoides (Sars, 1863), T. crassus (Fischer, 1853) and cladoceran L. kindtii) were monitored in every sample. Fish for gut content analysis were collected by fyke nets in the littoral and gillnets in the pelagic zone. In the laboratory, the fish were analysed for their gut content. The Cladocera carapaces found in fish guts were classified to species level and counted.
Concurrently with the zooplankton sampling, temperature, conductivity, pH, concentration of dissolved oxygen, total organic carbon (TOC), and total suspended solids (TSS) were measured. These data were used in further processes to estimate the impact of physical and chemical factors on the distribution of brood-bearing females. They were also used to calculate the depth of the epilimnion (temperature 21–23 °C, oxygen concentration 7.42–7.78 mg·dm−3 in June), metalimnion (8–10 °C, 8.92–9.12 mg·dm−3) and hypolimnion (7.3–4.8 °C, 6.25–8.34 mg·dm−3), and samples gathered in the deep pelagic zone were attributed to the adequate layers. The investigations of macrophytes were carried out in April, May, June, August, and October. The biomass of common reed stands was estimated at five randomly chosen sites limited by a floristic fork (0.25 m2 of sampling area). Species composition and biomass of submerged macrophytes were obtained with the use of the fork-type sampler (0.16 m2 of sampling area). Samples were washed and dried at 60 °C to a constant weight. These data were used in further process to estimate the impact of macrophyte biomass on the distribution of brood-bearing cladocerans.
2.3 Statistical analyses
The average density of egg-bearing individuals for each of sampling sites was counted on the whole number of samples collected in distinct site for distinct month, according to the formula:
On the basis of these data, a relative horizontal distribution (RHD) of individuals bearing embryos was calculated using the equation:
On the basis of the data collected vertically in the DL1, DL2 and DP, a mean residence depth (MRD) was estimated for each species [30]:
Spearman correlation coefficients were used to estimate the relations between the density of Cladocera and potential invertebrate predators. The feeding selectivity of fish towards the studied species of Cladocera was evaluated according to Chesson's formula [31]:
ANOVA with post-hoc Bonferroni tests were run to determine the differences in the vertical and horizontal distribution of cladocerans. Canonical correspondence analysis was used to determine the variables that best predicted the distribution of brood-bearing cladocerans. As a final result of that analysis, a set of environmental variables was reduced to a few orthogonal axes as composite environmental gradients structuring species distribution patterns. Stepwise forward selection was used to include significant variables (P < 0.05) in the model. The significance of the first canonical axis and of all canonical axes together was tested by the distribution-free Monte Carlo simulation (499 permutations).
3 Results
3.1 Predatory impact on Cladocera
3.1.1 Planktivorous fish
Among the fish netted in Lake Piaseczno, planktivorous fish constituted 98.9 % of all fish collected and were represented mainly by roach Rutilus rutilus L. (57 %), perch Perca fluviatilis L. with body length < 15 cm (16.8 %), catfish Ictalurus nebulosus Lesueur, 1819 (15.5 %), white fish Coregonus albula L. (4 %), and bleak Alburnus alburnus L. (4 %). The analysis of the gut contents of these species of fish revealed that B. coregoni and D. longispina were the preferred food – the values of Chesson's coefficient were α = 0.93 ± 0.15 for B. coregoni and α = 0.71 ± 0.22 for D. longispina. Chesson's coefficient for D. cucullata reached α = 0.49 ± 0.31, suggesting an unselective forage of fish on that species. D. brachyurum (α = 0.015 ± 0.015) and B. longirostris (α = 0.21 ± 0.18) were avoided by fish.
3.1.2 Potential invertebrate predators
L. kindtii appeared from May to September, and their density computed for the whole period of research ranged from 100 ± 80 ind·m−3 in the DL2 in transect 1 and 50 ± 50 ind·m−3 in the NS in transect 2 to 340 ± 179 ind·m−3 in the DP. No individuals of L. kindtii were found in DL1 in both transects. Cyclopoid copepods appeared from April to November, their densities were similar in both transects and ranged from 1000 ± 534 ind·m−3 in the NS to 18 200 ± 9200 ind·m−3 in the DP. The values of Spearman correlation coefficients between the density of B. longirostris and the density of predatory cyclopoids and L. kindtii suggested a high vulnerability of B. longirostris to the predation pressure of invertebrates (r = –0.58, P = 0.021 for cyclopoid copepods; r = –0.32, P = 0.043 for L. kindtii). Of the two Daphnia, D. longispina seemed to be more vulnerable to Leptodora's predation, as their collapses in density corresponded with the occurrence of L. kindtii in densities higher than 500 ind·m−3 (r = –0.72, P = 0.040). The density of B. coregoni did not correlate significantly either with that of cyclopoids or with that of L. kindtii. Significant values of correlation coefficients indicated that an increasing population of Diaphanosoma coincided with a high occurrence of invertebrates (r = 0.36, P = 0.027 for cyclopoid copepods, r = 0.24, P = 0.013 for L. kindtii).
3.2 Density of brood-bearing cladocerans
The average density of brood-bearing B. longirostris computed for the whole period of research was 498 ± 265 ind·m−3 (1.7–8.6 % of total density of adults) in transect 1 and 471 ± 129 ind·m−3 (0.8–7.4 %) in transect 2. B. coregoni displayed 594 ± 274 ind·m−3 (1.8–16.5 % of the total density of adults) in transect 1 and 597 ± 271 ind·m−3 (3.1–11.1 %) in transect 2. Brood-bearing D. cucullata ranged between 524 ± 517 ind·m−3 (4.1–7.5 %) in transect 1 and 478 ± 295 ind·m−3 (2–11.9 %) in transect 2, whereas the density of D. longispina was 624 ± 519 ind·m−3 (6.1–34 %) in transect 1 and 509 ± 336 ind·m−3 (3–52.1 %) in transect 2. D. brachyurum ranged between 636 ± 226 ind·m−3 (3.3–8.2 %) in transect 1 and 729 ± 113 ind·m−3 (4.3–9.3 %) in transect 2.
3.3 Distribution of brood-bearing cladocerans – horizontal aspect
3.3.1 Ovigerous females
Two trends in the distribution of the brood-bearing females were apparent. In April–June (transect 1) or April–May, and then in October–November (transect 2), the ovigerous cladocerans existed both in the littoral and pelagic zones, whereas in the rest of the months, they avoided the littoral zone (Fig. 2). However, when taking into consideration the distribution of every species separately, each of them displayed a different pattern. ANOVAs showed that the distribution of B. coregoni (F = 0.13, P = 0.039), D. longispina (F = 0.21, P = 0.027), and D. cucullata (F = 0.29, P = 0.041) differed significantly between transects. In general, the cladocerans showed insignificant differences in the horizontal distribution in the pelagic zone (ANOVA F = 0.15–029, P > 0.05), and significant differences in the distribution in the littoral zone (F = 0.2–0.45, P < 0.05), except D. brachyurum, of which horizontal distribution in the littoral zone was statistically insignificant. D. brachyurum was the only species displaying an even horizontal distribution in both transects (Fig. 3a). B. coregoni rather gathered in the pelagic zone and occurred in the littoral zone only temporarily in May in transect 2, and then in October–November in both transects (Fig. 3b). B. longirostris displayed similar pattern of distribution to that of B. coregoni, with occurrences in the littoral zone in April–May and October–November and avoidance of that zone in June–September in both transects (Fig. 3c). D. longispina apparently avoided the NS, and only in transect 2 it occurred there in higher number in November (Fig. 3d), whereas D. cucullata existed mainly in the sublittoral and in the pelagic zone, and it occurred in the NS (transect 1) and the DP2 (transect 2) only in May (Fig. 3e).
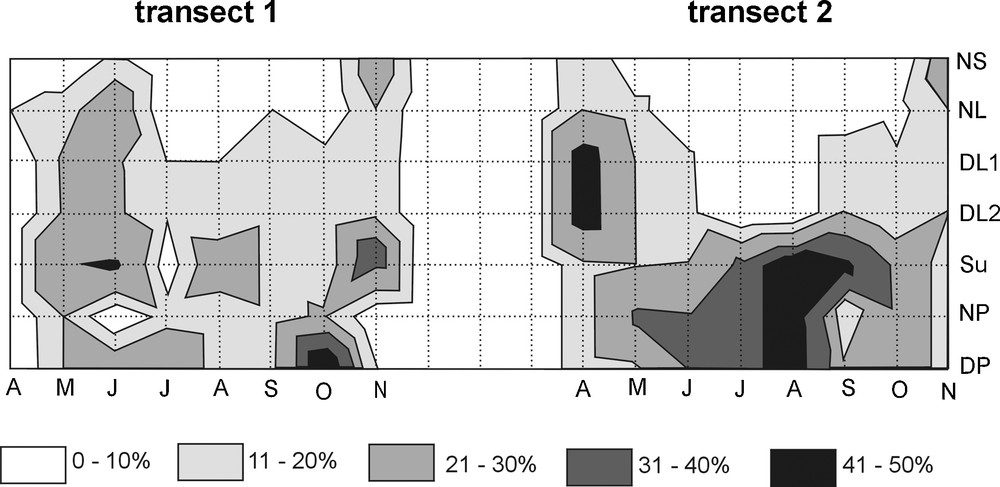
The horizontal relative density (%) of brood-bearing Cladocera in the horizontal gradient in Lake Piaseczno.
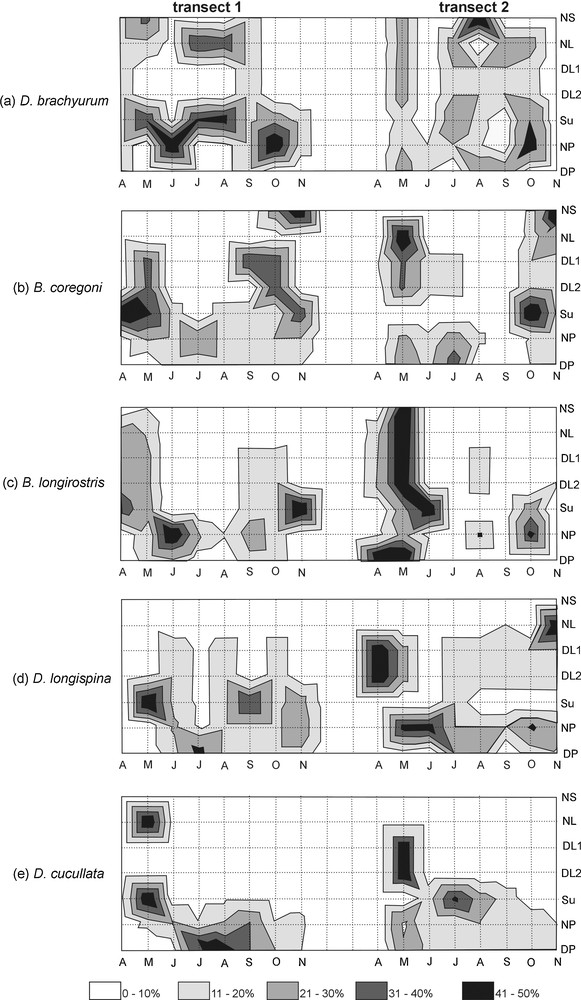
The spatial relative density (%) of brood-bearing D. brachyurum (a), B. coregoni (b), B. longirostris (c), D. longispina (d), and D. cucullata (e) along the horizontal gradient in Lake Piaseczno.
3.3.2 Environmental background
Based on forward model selection, conductivity (λa = 0.069, F = 2.73, P = 0.017), TOC (λa = 0.051, F = 2.21, P = 0.013), and macrophyte biomass (λa = 0.066, F = 2.034, P = 0.037) were identified as the variables best explaining horizontal distribution of Cladocera. CCA was performed using only these variables (Fig. 4). The correlation coefficients showed that axis 1 of the CCA was a gradient of increasing conductivity, whereas axis 2 was largely defined by macrophyte biomass. Ordination scores indicated a positive association between the conductivity and the density of the two Bosmina species and D. cucullata (r = 0.31, P = 0.04 for B. longirostris; r = 0.21, P = 0.019 for B. coregoni; r = 0.29, P = 0.03 for D. cucullata). D. longispina and D. brachyurum showed weak but significant negative correlations with TOC (r = –0.13, P = 0.017 for D. longispina, r = –0.09, P = 0.035 for D. brachyrum), whereas the remaining species correlated positively with that parameter (r = 0.21–0.36, P = 0.004–0.027). D. longispina displayed weak but significant positive correlation with the biomass of macrophytes (r = 0.18, P = 0.032), whereas Bosmina species correlated negatively with them (r = –0.21, P = 0.046 for B. coregoni, r = –0.36, P = 0.012 for B. longirostris). However, eigenvalues of CCA axes 1 (λ = 0.22) and 2 (λ = 0.17) were relatively weak and the canonical eigenvalues accounted together for only 21.7 % of the total variance.
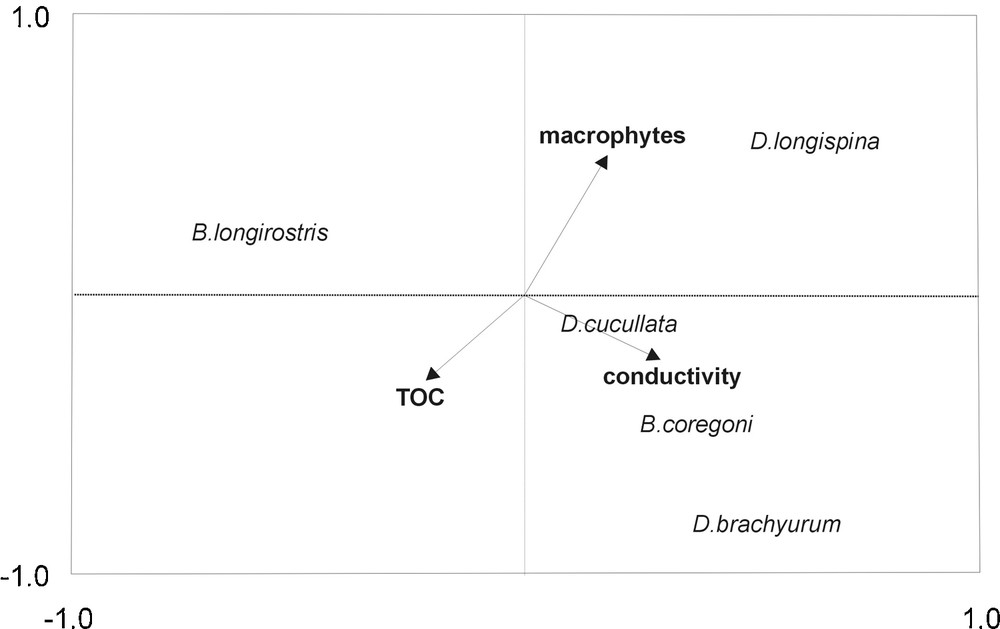
Canonical Correspondence Analysis (CCA) biplot for egg-bearing cladocerans showing species and environmental variables in the horizontal aspect.
3.4 Distribution of brood-bearing cladocerans – vertical aspect
3.4.1 Ovigerous females
The mean residence depth (MRD) of the studied cladocerans in the littoral zone ranged between 1.1 and 3.7 m in transect 1 and between 0.7 and 3.5 m in transect 2. No significant differences in the MRD values of distinct species were found either between each site or between the DL1 and DL2 (ANOVA F = 1.19–3.27, P > 0.05). Mean residence depths of B. longirostris and B. coregoni differed significantly between transects (F = 0.5, P = 0.027 for B. longirostris, F = 2.14, P = 0.044 for B. coregoni), because those species existed at lower depths from July to September (MRD = 2.9–3.7 for B. longirostris and 2.6–3.5 for B. coregoni), and ascended in the remain months in transect 1, whereas in transect 2 they did not show such changes in the distribution pattern occurring at the depth between 1.5 and 3.2 m.
In the pelagic zone, the bulk of egg-bearing D. cucullata preferred the epilimnion and metalimnion throughout the year (MRD = 2.1–5.6 m). D. longispina also existed in the epilimnion and metalimnion (MRD = 1.9–6.3 m). In the pelagic zone, B. longirostris occurred mainly in the metalimnion and hypolimnion (MRD = 8.9–15.4 m), whereas D. brachyurum and B. coregoni were mostly found in the epilimnion and metalimnion (MRD = 2.5–5.7 m for D. brachyurum, MRD = 1.6–4.9 m for B. coregoni).
3.4.2 Environmental background
All the environmental variables used to build the CCA orthogonal axes of gradients best structuring the vertical distribution pattern of egg-bearing cladocerans in the littoral zone proved to be insignificant (λ = 0.006–0.022, P > 0.05), whereas the environmental variables included by the CCA forward selection as best differentiating the vertical distribution in the pelagic zone were temperature (λa = 0.08, F = 3.17, P = 0.002), dissolved oxygen (λa = 0.12, F = 4.12, P = 0.014), and conductivity (λa = 0.08, F = 3.21, P = 0.034). CCA was performed using only these variables (Fig. 5). Correlation coefficients showed that axis 1 of the CCA was a gradient of increasing temperature and oxygen content, whereas axis 2 was largely defined by conductivity. Individual ordination scores for cladocerans indicated that D. cucullata selected the habitats with regard to dissolved oxygen (r = 0.17, P = 0.044). Temperature correlated with the density of D. brachyurum (r = 0.43, P = 0.009) and B. longirostris (r = –0.27, P = 0.031), whereas conductivity correlated with the density of B. coregoni (r = 0.31, P = 0.039). The interia in the species after fitting the variables was 1.863. Of this, the first axis explained 17 %, and the second axis 9.2 %. The canonical eigenvalues accounted together for 42.9 %.
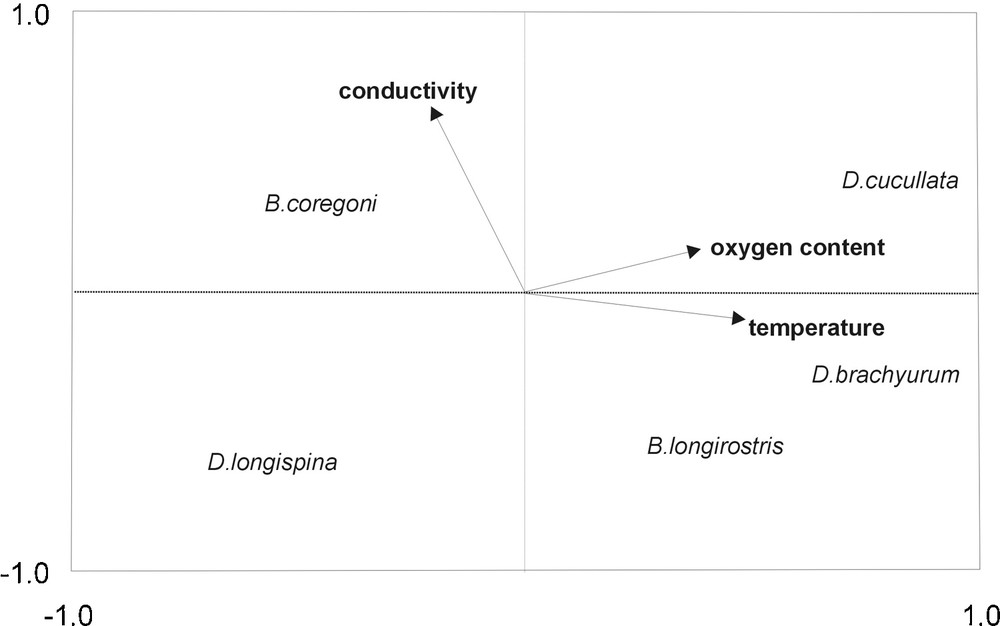
Canonical Correspondence Analysis (CCA) biplot for egg-bearing cladocerans showing species and environmental variables in the vertical aspect.
4 Discussion
4.1 Predatory impact on Cladocera
The correlations between the density of the studied species of Cladocera and their abundances in guts of fish as well as the density of planktonic invertebrates (cyclopoid copepods and L. kindtii) suggest that the studied cladocerans displayed very distinct vulnerabilities to predation pressure. The density of D. longispina and B. coregoni were affected by fish predation pressure, D. cucullata were under the moderate influence of planktivorous fish, and planktonic invertebrate L. kindtii, B. longirostris were influenced by cyclopoid copepods and L. kindtii, whereas D. brachyurum did not show any apparent symptoms of being under predation pressure. The results confirm our previous work on the effect of predatory impact on life histories of limnetic species of Cladocera, which showed that vertebrate and invertebrate predators have a major but different impact on density and reproductive output of cladocerans [32,33]. The disproportions between the even horizontal distribution of non-predated D. brachyurum and the remaining species being under predation pressure, which apparently avoided the littoral habitats in May–August, i.e. the season of intense growth of macrophytes, suggest that the distribution of brood-bearing cladocerans was affected by predation pressure. However, although pelagic invertebrate and vertebrate predators detect their prey differently [21–23], the kind of predators that were dominant seemed to have no special influence on the distribution of particular species. Invertebrate-predated B. longirostris and fish-predated B. coregoni displayed a very similar horizontal distribution. Similarly, fish-impacted D. longispina and invertebrate-impacted D. cucullata showed similar preferences in avoiding the shallower parts of the lake.
4.2 Distribution of brood-bearing females – horizontal aspect
The studied species, although preferring to live in open water areas, explored the littoral zone, especially in early spring and late autumn, but their abundance was very low there from June/July to September. This suggests that none of the examined macrophyte patches in Lake Piaseczno constituted refuges for brood-bearing cladocerans. Daphnia species and Bosmina species with their broods avoided existing in the NS covered with emergent macrophytes, preferred to stay in the DL2 covered with morphologically simpler macroalgae patches, and avoided the DL1 covered with more dense (thus potentially better as a refuge) beds of water milfoil. The results are in accordance with the model of relations between the refuge role of submerged macrophytes and the lake's trophic state, as described by Jeppesen et al. [34], which predicts that macrophytes may be less efficient refuges for cladocerans against planktivorous fish in mesotrophic lakes because they do not provide an efficient shelter due to the high lucidity of water. That statement cannot be regarded concerning the interactions between either cyclopoid copepods or Leptodora and their prey, as foraging of planktivorous fish is mainly directed by vision [21], whereas cyclopoids detect their prey with mechanoreceptors sensitive to hydrodynamic disturbances resulting from swimming movements of a prey [22], and Leptodora needs tactile direct contact [23]. However, laboratory experiments conducted by Adamczuk [35] showed that the spatial complexity of habitats could hinder foraging activity of predatory invertebrates by impeding water disturbances created by swimming cladocerans and obstruct tactile contact of Leptodora with cladocerans. Notwithstanding, in Lake Piaseczno, the predatory type had no influence on the refuge role of macrophytes for brood-bearing individuals, since invertebrate-impacted cladocerans showed a pattern of vegetation avoidance similar to the distribution pattern of fish-impacted species.
4.3 Distribution of brood-bearing females – vertical aspect
Vertical distribution of cladocerans in the pelagic zone, particularly in the framework of diel migrations, is very well-documented [36,37]. The thermal layers (epilimnion, metalimnion and hypolimnion) in a pelagic zone of a deep lake are thought to play a refuge role because they show apparent vertical gradients in light, temperature, and oxygen capacity, thus creating natural boundaries that can reduce the mortality of cladocerans [38–40]. Viroux’ research [41] suggests that egg-bearing cladocerans are able to display a vertical distribution even in river ecosystems, where they gather in higher number in bottom layers. In the pelagic zone of Lake Piaseczno, egg-bearing cladocerans displayed a clear vertical distribution. The species existed in the epilimnion and metalimnion and their mean residence depth ranged between 1.6 m and 6.3 m, except B. longirostris, which preferred to stay in the metalimnion and the upper layer of hypolimnion. Vertical distribution of cladocerans in the littoral zone has not been studied yet. The present study showed that in the littoral zone of Lake Piaseczno, the cladocerans existed between 0.7 and 3.5 m. The seasonal changes in the mean residence depth of distinct species were statistically insignificant. Furthermore, none of the abiotic factors significantly influenced vertical distribution of cladocerans in the littoral zone, including submerged macrophytes that differed in the volume occupied, with water milfoil infesting almost the whole water column in the DL1, and macroalgae infesting a much lower capacity in the DL2. Insignificant differences in residence depth both in the DL1 and the DL2 and between these two sites confirmed that spatial architecture was not a decisive factor in their vertical distribution in the littoral zone.
4.4 The role of environmental variables in the distribution of brood-bearing cladocerans
The role of abiotic parameters in the distribution of cladocerans still remains an unsolved problem. The same species usually inhabit a wide range of lakes, although in different abundances, and the major environmental requirements for many species are almost the same [42], suggesting they are ecologically flexible. On the other hand, it has been shown in some studies that Cladocera are sensitive to many physical, chemical, and ecological factors [43,44]. Some studies have reported the influence of physical and biological factors on the distribution of zooplankton in lakes. Pinel-Alloul et al. [45] found that water temperature, oxygen concentration, wind direction and chlorophyll a were factors driving the spatial distribution of some taxa of macrozooplankton. Other studies suggest that light can influence the spatial distribution of species [17,18]. The present study showed that some environmental factors proved to be also important in the distribution of brood-bearing females. One of the hypotheses stated in the study was that some environmental variables and predatory type co-determine the distribution of ovigerous females, focusing on the special role of macrophytes in the littoral zone and of abiotic parameters in the pelagic zone. However, predatory type turned out to have no apparent influence on the distribution of cladocerans, but distinct environmental parameters had. In the horizontal aspect, the macrophyte biomass impacted the density of the Bosmina species and D. longispina, the conductivity impacted the density of Bosmina sp. and D. cucullata, and the TOC affected the density of all the studied species. However, in the vertical aspect of the same parameters, the temperature correlated significantly with the density of D. brachyurum and B. longirostris, the dissolved oxygen impacted the density of D. cucullata, and the conductivity correlated with the density of D. brachyurum. The mechanisms by which temperature, dissolved oxygen, and macrophytes influence the cladocerans are known. Conductivity, which turned out to be an important parameter, has been repeatedly reported as a decisive factor in the distribution of cladocerans, both in geographical and whole-lake scale [46,47], although the mechanism of its influence on cladocerans is not clear. Apparently, the distribution of ovigerous females could play some role in the competition between the studied cladocerans. The species play different roles in the food web, and some studies have reported strong competitive interactions between the limnetic species of Cladocera [48]. Daphnia are often ascribed the key role in water bodies [49], and due to the niche overlap, they are able to compete with D. brachyurum [50] as well as with Bosmina sp. [51,52]. That competition between the species often causes the dominant species to expel the weaker ones to worse habitats. Daphnia's avoidance of more complicated habitats is well known [53,54]. In Lake Piaseczno, ovigerous D. cucullata and D. longispina settled mainly in the pelagic zone and occurred rarely in the littoral zone. Littoral habitats have reported to be “worse” for limnetic cladocerans, which are obligatory filter-feeders, to exist by virtue of food availability, because the productivity of phytoplankton is frequently reduced there due to a weaker light climate and faster sedimentation than in the pelagic zone [55,56]. Therefore, when analysing the causes of species distribution in Lake Piaseczno, it could be possible that the distribution pattern of brood-bearing D. brachyurum could partly result from avoidance of Daphnia. Similarly, the temporal shifts of Bosmina between littoral and pelagic habitats could play a part in the trade-off to deliver offspring in the area of both lower predation pressure and free from Daphnia competition.
5 Conclusion
The studied species of Cladocera showed apparent and regular differences in horizontal distribution in the littoral zone, and lack of such differences in the pelagic zone. Their vertical distribution, in turn, showed a clear pattern in the pelagic zone and less regularities in the littoral zone. The differences in spatial distribution of non-predated and predated species suggest that predation pressure, but not predatory type, was an important factor structuring their distribution, particularly in the horizontal aspect. However, the temporal occurrence/absence of egg-bearing cladocerans in distinct habitats was only partly structured by predation avoidance, which turned out to be a crucial but not exclusive factor. Other factors that occurred to be significant in their distribution were macrophyte biomass, conductivity and TOC in the horizontal aspect, and temperature, dissolved oxygen and conductivity in the vertical aspect. However, most of those factors better explained the vertical distribution of brood-bearing cladocerans in the pelagic zone than their horizontal distribution in the littoral zone. The vertical and horizontal distributions of distinct species suggest that competitive interactions between the studies cladocerans may be a possible factor influencing their distribution pattern.
Disclosure of interest
The authors declare that they have no conflicts of interest concerning this article.