1 Introduction
Medicinal plants are the major source of life-saving drugs for the majority of the world's population. Extracts of a large number of these plants have beneficial therapeutic effects [1]. For example, the volatile oil from seeds of Nigella sativa contains biologically active compounds that have been used in the Middle East as a natural remedy for various diseases for more than 2000 years [2].
A major constituent of N. sativa seed oil is thymoquinone (TQ). This molecule is reported to have anti-oxidative, anti-inflammatory and anti-neoplastic effects [3]. TQ also has anti-bacterial [4,5] and anti-fungal [6,7] activities. Recently, TQ was found to induce anabolic effects on the mouse (Mus musculus) MC3T3-E1 cell line and an increased expression of bone morphogenetic protein (BMP)-2 [8]. TQ belongs to a family of quinones that undergoes enzymatic and non-enzymatic redox cycling with the respective semiquinone radicals to generate superoxide anion radicals [9]. Other quinones (e.g., furanonaphthoquinones, 2-acylamine-1,4-napthoquinone and 1,4-napthoquinone) are known to induce apoptosis in cancerous cells by generating reactive oxygen species (ROS) [10–12].
In a variety of cancerous cell types, TQ has growth inhibitory effects through the inhibition of DNA synthesis and the induction of cell cycle arrest [13,14]. TQ shows significant toxicity against pancreatic, lung, colon, uterine and leukemic carcinomas, but has no effect on the growth of non-cancerous cell lines [15,16]. TQ also modulates the Bax/Bcl2 ratio by upregulation of pro-apoptotic Bax and downregulation of anti-apoptotic Bcl2 proteins in p53-null human myeloblastic leukemia HL-60 cells during apoptosis [17]. Several studies on animal cells demonstrated that TQ induces growth inhibition through the suppression or activation of various signaling pathways [8,14,17,18]. Potential action of TQ in inhibiting the proliferation of human cancer cells is based on its ability to increase the phosphorylation states of the mitogen-activated protein kinases (MAPK) JNK and ERK [8,19]. However, the precise mechanism by which TQ alters these cellular pathways and induces growth inhibition is yet to be elucidated.
The Bcl2-associated athanogene (or BAG) family was originally detected in mammals. The proteins encoded by BAG genes interact with the anti-apoptotic protein, Bcl2, and promote cell survival [20]. A gene family encoding BAG-like proteins (BAG-1-7) was characterized in Arabidopsis [21]. Members of the BAG protein family have a conserved 50 amino acid domain referred to as the BAG domain (or BD) that interacts with heat shock protein 70 (HSP70/HSC70) [22]. This family of co-chaperones functionally regulates diverse cellular pathways, including programmed cell death (PCD) and stress responses [23].
The present work sought to examine the possible effects of TQ on dividing plant cells with the intention of developing an experimentally tractable system to investigate the molecular basis of TQ on eukaryotic cells. In particular, the possible anti-mitotic and cytotoxic activities or cell death-inducing effects of TQ on plant dividing cells were investigated. We also report the identification of BAG-like genes from soybean (Glycine max), tomato (Solanum lycopersicum), Nicotiana benthamiana, and other plant species that are homologous to the AtBAG6 gene in Arabidopsis and we examined the expression of the soybean gene in roots in response to TQ treatment. In the present work, we studied the effects of TQ in inducing growth inhibition and on triggering cell death in dividing plant cells.
2 Materials and methods
2.1 Plant material
Wheat seeds (cv. Giza 164) and onion bulbs (cv. Beheri) were obtained from Field Crops Research Institute and Horticultural Crops Research Institute, respectively of the Agricultural Research Center (ARC), Giza, Egypt. Soybean (G. max) seeds (cv. Jack) were obtained from Department of Biological Sciences, Faculty of Science, King Abdulaziz University, Jeddah, Saudi Arabia.
2.2 Organic synthesis of TQ
TQ was synthesized from thymol by the method described by Kremers et al. [24]. The purity and chemical structure of the synthesized TQ were analyzed in Plant Pathogen Interaction Lab., Agricultural Genetic Engineering Research Institute (AGERI), ARC, Giza, Egypt, by GC–MS (HP-5890 GC equipped with HP-5972 mass spectrometer, HP-innowax column 30 m × 0.25 mm id × 0.25 μm film thickness) with helium in the mobile phase and 1 mL/min flow rate. Oven temperature was first set at 50 °C for 2 min, then gradually increased at a rate of 15 °C/min up to 200 °C. The mass detector temperature was set at 300 °C and the obtained mass spectrum was automatically compared to that in Wiley 7 N mass library.
2.3 Effects of TQ on wheat seed germination rate
The effects of TQ at 0.1 and 0.2 mg/mL on the germination rate of wheat seeds were tested in MS [25] medium (Duchefa Biochemie, The Netherlands) solidified by adding 1% agar. Ten seeds/replicate/treatment were surface sterilized, placed in Petri dishes containing MS medium. Experiments were conducted in four replicates and analyzed as a randomized complete block design. The plates were kept at 21 ± 2 °C during the day, and then shifted to 4 °C during the night. Germination was recorded starting from day 3 up to day 6. Emergence of the radicle from the seed coat was considered as completion of germination. Statistical analysis was performed following the procedure outlined by Gomez and Gomez [26].
2.4 Effects of TQ on growth of onion hairy root tips
The onion bulbs were cultured in beakers (50 mL) containing 25 mL of Hoagland solution [27] and left in a growth chamber at 25 °C for four days. The roots were placed for 1 h in TQ at a concentration of 0.2 mg/mL. The dividing cells in onion root tips were examined by light microscope and transmission electron microscope JEOL (JEM-1400 TEM, Japan) at the candidate magnification. Images were captured using CCD camera model AMT (Advanced Microscopy Techniques, Corp., Woburn, MA 01801, USA).
2.5 Measurement of cell death by Evans blue staining
Cell death was determined and quantified in G. max root tips treated with TQ (0.2 mg/mL) for 1 and 2 h by using Evans blue as described by Kim et al. [28]. Untreated roots were used as a control. Ten seeds/replicate/treatment were surface sterilized, sown in pots with soil:vermiculate mixture (1:1). Roots (1 mL in length) were submerged in 0.25% (w/v) Evans blue solution (MP Biomedicals, Santa Anna, CA, USA) for 20 min. Excess and unbound dye was removed with deionized water and root death was scored visually. Then, the roots were placed in liquid nitrogen, homogenized, placed in 1% (w/v) sodium dodecyl sulfate (SDS) solution, and incubated for 10 min at 37 °C. Solutions were centrifuged at 13,000 × g for 5 min. The quantity of remaining dye was spectrophotometrically measured at 600 nm. Measurements were expressed as relative values, with 1 corresponding to a maximum of a sample tested and the experiments were conducted in four replicates. Multiple comparisons were performed for value means as outlined by Duncan's New Multiple Range test [29].
2.6 BLAST analysis of BAG-like proteins in G. max and homologs in tomato and N. benthamiana
Six AtBAG protein family members (AtBAG1-6, Table 1) in the NCBI Arabidopsis database (taxid: 3702) were used to search via BLAST the NCBI G. max database (taxid: 3847). The criterion to accept an uncharacterized protein of G. max as a putative BAG-like protein was based on the conservation of critical amino acids in the BD domain. Four protein homologs (with accession numbers shown in Table 2) were detected and utilized for further molecular analysis. From the mRNA sequences corresponding to these four predicted proteins, DNA sequences were identified and primers specific to the four uncharacterized putative BAG-like genes were designed to recover amplicons with 300–350 bp (Table 3). Additionally, primer pairs for a number of G. max metacaspase genes to recover 300–400 bp, and a primer pair to recover 265 bp of the G. max actin mRNA (positive control) were also designed. Homologs of BAG genes were identified in the tomato and in N. benthamiana draft genome sequences using the resources at the Sol Genomics Network [30] (http://solgenomics.net/).
Accession numbers of BAG-like proteins of Arabidopsis utilized in blasting G. max NCBI protein database based on the conservation of the critical amino acids in the BD domain.
Size (aa) | Name | Protein accession no. |
342 | Bcl2-associated athanogene 1 (BAG-1) | NP_200019 |
296 | Bcl2-associated athanogene 2 (BAG-2) | NP_851246 |
303 | Bcl2-associated athanogene 3 (BAG-3) | NP_196339 |
269 | Bcl2-associated athanogene 4 (BAG-4) | NP_190746 |
215 | Bcl2-associated athanogene 5 (BAG-5) | NP_172670 |
1043 | Bcl2-associated athanogene 6 (BAG-6) | NP_182147 |
Putative AtBAG protein family (AtBAG1–6), Table 1 found by BLAST analysis of the NCBI G. max database (taxid: 3847) based on the conservation of the critical amino acids in the BD domain.
Size (aa) | Max identity (%) | E value | Query coverage (%) | Name | Protein accession no. |
179 | 30 | 1 × 10−06 | 9 | Uncharacterized protein LOC100814935 | NP_001240099 |
182 | 29 | 3 × 10−07 | 9 | Uncharacterized protein LOC100820130 | XP_003534820 |
254 | 33 | 1 × 10−04 | 8 | Uncharacterized protein LOC100789587 | XP_003529169 |
1253 | 27 | 2 × 10−52 | 67 | Uncharacterized protein LOC100805117 | XP_003529909 |
Primer sequences and expected amplicon sizes of four G. max BAG-like genes as well as the six G. max metacaspase-like mRNAs corresponding to the four predicted uncharacterized proteins with the BAG domain. The actin mRNA of G. max was used as the positive control.
Amplicon Size (bp) | Primer sequence (5′-3′) | Primer name | mRNA Accession no. | Protein Accession no. |
BAG-like | ||||
315 | GCGTCCTCTACCGGAAAATC | GmBAG Pr-1-F | NM_001253170 | NP_001240099 |
CACCGTTCTCCAGACAAATAC | GmBAG Pr-1-R | |||
315 | AAGCTGCGAATGAACGAGG | GmBAG Pr-2-F | XM_003534772 | XP_003534820 |
AAGGGGGAGTGGTGAATCAG | GmBAG Pr-2-R | |||
334 | GGAGGATGCTTCTAGTGAAGAG | GmBAG Pr-3-F | XM_003529121 | XP_003529169 |
CTTTACCAATGGGGGTAAAAGG | GmBAG Pr-3-R | |||
320 | ATGCGGGTCGAAAAGATGG | GmBAG Pr-4-F | XM_003529861 | XP_003529909 |
TCCCTAGCCAAGGATTTTCTG | GmBAG Pr-4-R | |||
Metacaspase-like | ||||
400 | CTCCTCATCAACAAGTTCAGC | Gm-MTC1-F | XM_003516742 | XP_003516790 |
CATATTGCCCACTCCTGTTC | Gm-MTC1-R | |||
398 | GGAATTTGTTGATGGGGTGC | Gm-MTC4-F | XM_003531762 | XP_003531810 |
CATCAAAGAGTGTAGGTCTCAG | Gm-MTC4-R | |||
392 | ACGTGTCCTCACAGAAGAAG | Gm-MTC3-F | XM_003547587 | XP_003547635 |
TTTTACTATGGGGTGGTTTGC | Gm-MTC3-R | |||
369 | CGTGTCCTTACAGAAGAACAG | Gm-MTC2-F | NM_001255418 | NP_001242347 |
ATCCTTCCATTTCCAACTGC | Gm-MTC2-R | |||
366 | GTTTCAGATTGTTGCCATAGTG | Gm-MTC5-F | XM_003532855 | XP_003532903 |
CAGAATTGTGTGTCCTCCTC | Gm-MTC5-R | |||
392 | GATCCCTCTAACATTGAGCTTC | Gm-MTC9-F | XM_003554030 | XP_003554078 |
GTGCTGCAACTTGGTTTTG | Gm-MTC9-R | |||
Actin | ||||
265 | GCTGCTGGAGAAGGATGGTT | Gm-actin-F | XM_003522781 | XP_003522829 |
TGGACACGTAGGGAGGAAGT | Gm-actin-R |
2.7 RT–PCR to analyze expression of putative GmBAG-like genes
Root tips of G. max were submerged in TQ (0.2 mg/mL) for 20 min, 40 min, 60 min, 80 min, 100 min or 120 min. Then, the roots were frozen in liquid nitrogen to extract RNAs. Untreated root tips were used as a negative control. Total RNAs were extracted from three similar-sized (1 mL) root tips per treatment using Trizol (Invitrogen) and treated with RNase-free DNase (Promega). First-strand cDNA was synthesized using 2.5 μg of the total RNA, 0.5 μg oligo (dT) primer and Superscript II reverse transcriptase (Invitrogen) to a final volume of 20 μL. PCR was performed in 20 μL reactions using 1 μL cDNA, 1 × PCR buffer (with 1.5 mM MgCl2), 200 μM dNTPs, 200 nM of each gene-specific primer and 0.2 U of GoTaq DNA polymerase (Promega). To ensure that no DNA contamination was present, PCR using two out of the six metacaspase as well as actin specific primer pairs (Table 3) was performed on the original mRNA samples and the results were negative (Fig. 1). To ensure that similar amounts of cDNAs were used for different treatments, parallel reactions with actin specific primers were run as a positive control. Primary denaturation of cDNA was done at 94 °C/3 min. Then, each PCR cycle (30 cycles) included denaturation at 94 °C for 30 s, annealing at 55 °C for 30 s, and extension at 68 °C for 30 s. Final extension step was done at 68 °C for 10 min. Amplicons were analyzed on a 1.2% agarose gel stained with ethidium bromide and visualized using the Gel Doc XR from Bio-Rad. RT–PCR was performed twice with similar results.
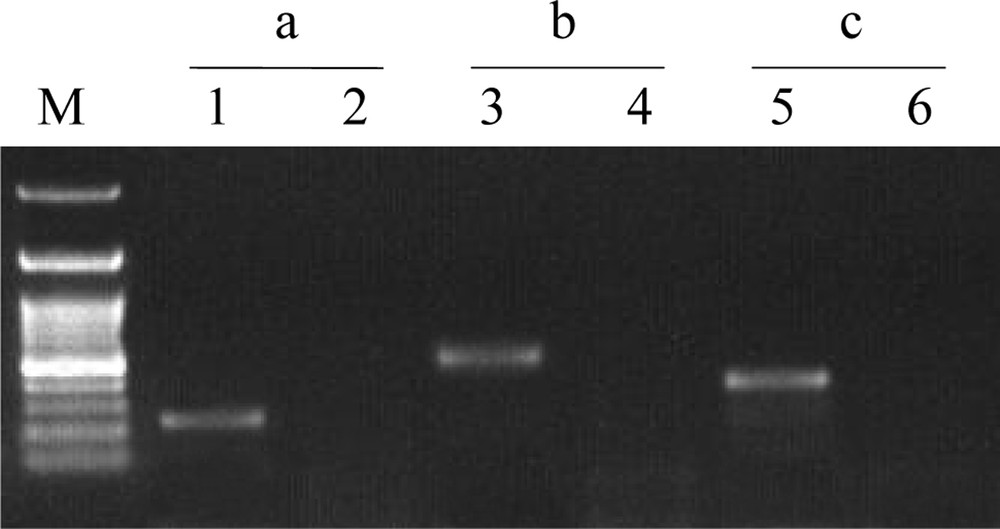
RT–PCR (lanes 1, 3 and 5) versus PCR (lanes 2, 4 and 6) analysis of mRNAs [accession nos. XM_003516742 (a) and XM_003531762 (b)] corresponding to two metacaspase-like proteins of G. max as well as the actin mRNA [accession no. XM_003522781 (c)] extracted from untreated roots. Primer sequences of the two metacaspase-like mRNAs as well as actin mRNA and amplicon sizes are shown in Table 3.
2.8 Detection of BAG-like protein type in G. max
One predicted BAG-like protein in G. max (with accession no. XP_003529909) was further examined as its corresponding mRNA showed a differential response across time of TQ treatment. This protein was compared with the BAG domains of the six AtBAG protein family members (AtBAG1-6) and a dendrogram was generated. Alignment of this predicted protein with BAG6-like protein in Arabidopsis was done to detect existence of the other BAG6-specific domain, namely IQ CaM-binding (or IQ) motif. Another uncharacterized, but conserved domain with unknown function, was also detected upstream IQ motif. This domain was used to BLAST against the whole NCBI database and homologs identified.
3 Results and discussion
Dividing cells of three plant systems were treated with TQ and observed for possible anti-mitotic activity, cytotoxic activity or ability to cause cell death. The results in Figs. 2 and 3 indicate that TQ effectively inhibited the seed germination rate of wheat (Giza cv. 164) at 0.1 mg/mL, and completely blocked germination at 0.2 mg/mL. The embryos of seeds treated with TQ at 0.2 mg/mL turned black in two days. It was also noticed that microbial contamination was completely prevented at 0.2 mg/mL TQ in the non-sterilized medium.
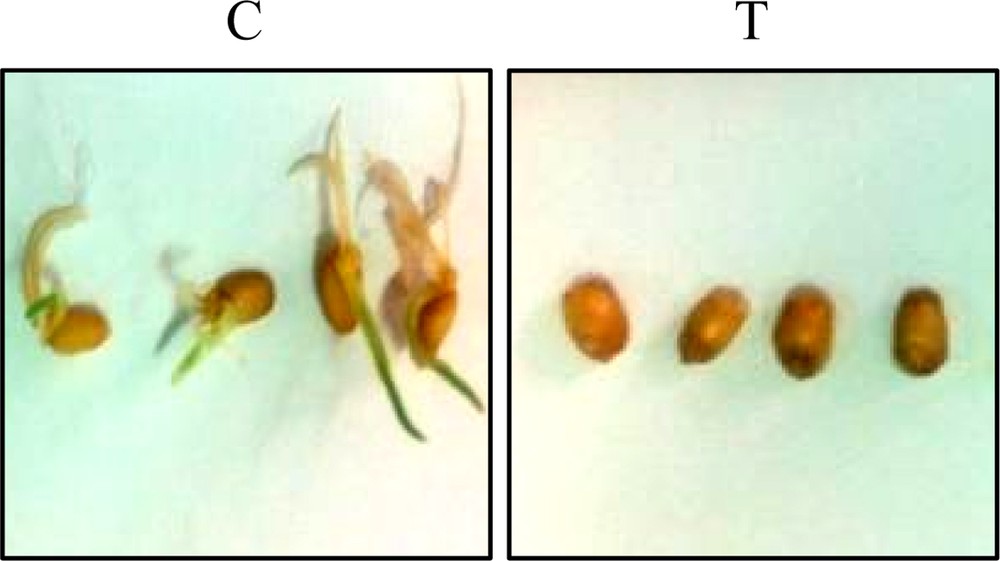
Effect of thymoquinone (0.2 mg/mL) treatment on germination of wheat seeds (T) as compared to the control untreated seeds (C). Color online.
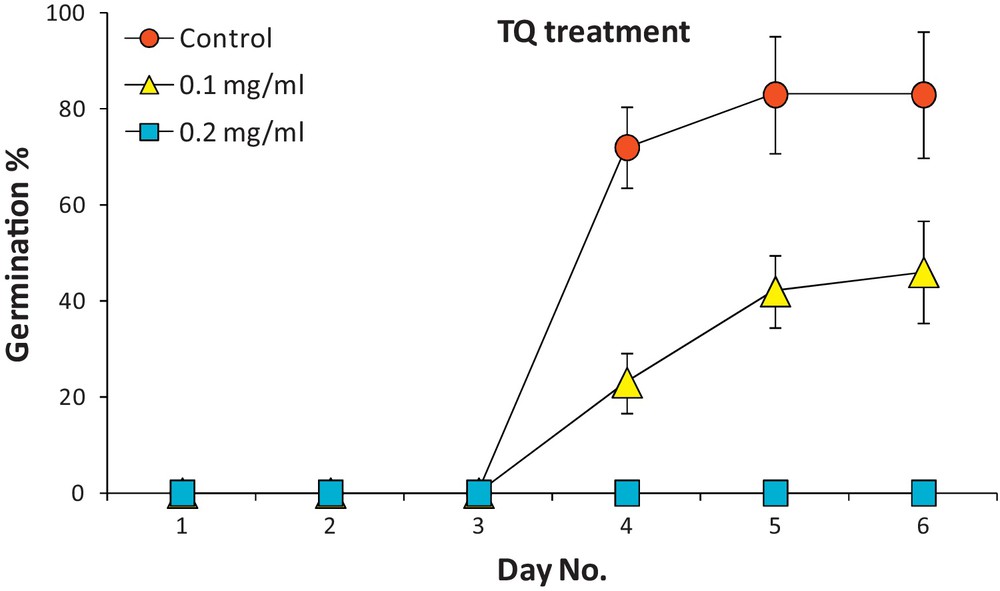
Influence of TQ at two concentrations (0.1 and 0.2 mg/mL) on germination of wheat seeds up to day 6.
Root tips of G. max treated with TQ at 0.2 mg/mL were observed to have moderate cell death after 1 h as indicated by an Evans blue assay (Fig. 4). It was evident that cell death took place exclusively in the meristematic zone of G. max roots. This region is known to contain actively dividing cells. Intensity of cell death was more pronounced after 2 h of TQ treatment (Fig. 4). Root tips of onion (Allium cepa L.) bulbs were also analyzed to gain knowledge about the cytotoxic effects of TQ. Histological examination was performed by light microscopy (Fig. 5) and transmission electron microscopy (Fig. 6) after soaking roots in 0.2 mg/mL TQ for 1 h. Several features indicative of cell death were observed in onion hairy root tip cells as compared to the untreated cells. For example, treated cells were visually enlarged and chromosomes showed fragmentation and condensed nuclei after 1 h of treatment (Fig. 5). Under the transmission electron microscope, we observed shrinkage of the plasma membrane, condensation of cytoplasm, leakage of cell lysate, degradation of the cell wall, and occurrence of large vacuole and condensed nuclei (Fig. 6).
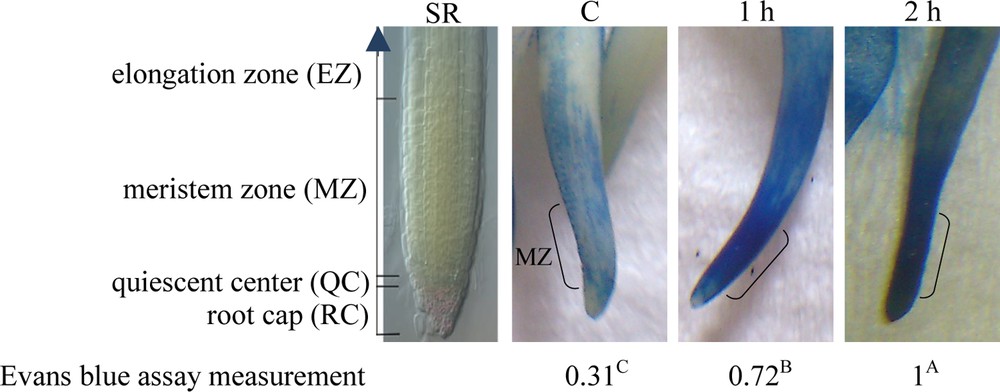
Visualization of Evans blue-stained meristematic zone of G. max roots treated with TQ (0.2 mg/mL) for 1 or 2 h as compared to the control untreated roots (C). SR refers to standard root structure [42,43]. Stained zone indicates dead cells. Dye released from dead root cells was measured at OD 600 nm. Measurements were expressed as relative values with one corresponding to the maximum of a sample. Multiple comparisons of value means indicate that means are significantly different as analysed by Duncan's New Multiple Range test [29]. Color online.
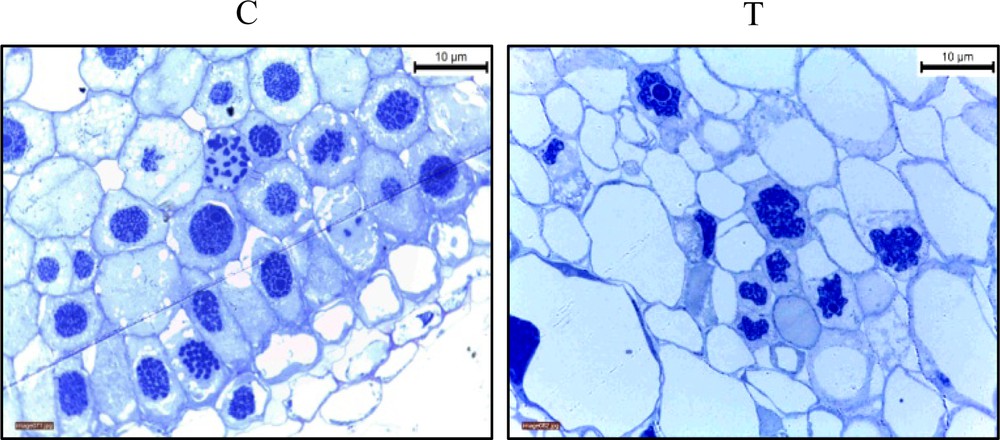
Light microscopic examination of cross sections of Giemsa-stained onion hairy root tips treated with TQ (0.2 mg/mL) (T) for 1 h as compared to the control untreated root tips (C). Treated cells were considerably enlarged and chromosomes showed fragmentation and condensed nuclei. Color online.

Transmission electron microscopic examination of cross sections of onion hairy root tips treated with TQ (0.2 mg/mL) (T) for 1 h as compared to the control untreated root tips (A). B–F = roots treated with TQ (0.2 mg/mL TQ) for 1 h. N = nucleus, PM = Shrinkage of the plasma membrane, CC = condensed cytoplasm, CL = leakage of cell lysate, CW = degraded cell wall, V = large vacuole, CN = condensed nucleus.
The present results suggested that TQ might be active exclusively in dividing cells of the three plant systems as it is in cancerous animal and human cells [1,8,12,14,17–19]. Previous reports demonstrated the mode of action of TQ as an inhibitor for the growth of various microbial species and various cancer cell lines [31], in addition to its inhibitory effects on some enzymes [32]. These effects of TQ were also investigated by Rasooli and Owlia [33], who reported that the main targets of thyme oils (containing TQ and other compounds) in Aspergillus parasiticus are the cell wall and membrane. The plasma membrane was associated with the formation of lomasomes, similar to the effects found in fungus cells treated with imidazole compounds. Quinones are known to form an irreversible complex with amino acids in nucleophilic proteins, which may lead to inactivation and loss of function of these proteins [34]. We hypothesize that TQ binds to the nucleophilic amino acids of the lysosome membrane in the plant cell causing membranes to lyse, thus, releasing all of the stored hydrolytic enzymes of the lysosomes in the cytosol. This may cause hydrolysis of all cell components after 1 h of treatment with TQ.
Several effects of thymol on Porphyromonas gingivalis, Selenomonas artemidis and Streptococcus sobrinus have also been examined by Shapiro and Guggenheim [35]. The extremely rapid efflux of intracellular constituents evoked by thymol is consistent with its postulated membranotropic effects. Shapiro and Guggenheim [35] suggested that membrane perforation might be the principal mode of action of thymol leading to cell death.
TQ has also been reported to induce apoptosis in human promyelocytic leukemia cells by its ability to increase the expression of pro-apoptotic genes, such as caspase-8 and p53 [17]. Accordingly, a number of six metacaspase-like genes (Table 3), e.g., metacaspases 1, 2, 3, 4, 5 and 9, were studied in G. max roots to detect their response to TQ treatment for 20–120 min. Metacaspase proteins are related to caspases and paracaspases [36], except that they are arginine/lysine-specific, while caspases are aspartate-specific [37]. Metacaspases are found in plants, fungi and protists, but not in animals, with one exception in the genome of Monosiga brevicollis [38]. In a similar manner to caspases, metacaspases induce cell death in plants and yeasts [39]. In the present study, we observed unchanged expression levels of the six tested metacaspase genes after TQ treatment over time, indicating no specific response of these genes to the treatment (Fig. 7).
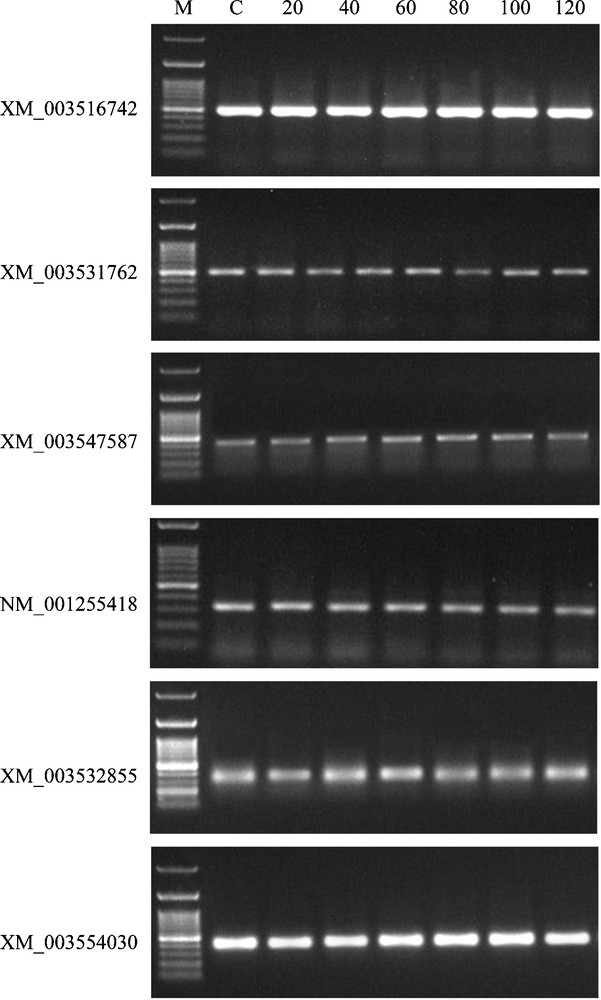
RT–PCR analysis of root mRNAs (XM_003516742, XM_003531762, XM_003547587, NM_001255418, XM_003532855 and XM_003554030) corresponding to the six metacaspase-like proteins of G. max as affected by treatment with TQ (0.2 mg/mL) for 0 (C), 20, 40, 60, 80, 100 or 120 min. The actin mRNA (accession no. XM_003522781) was used as a positive control. Primer sequences of the six metacaspase-like mRNAs as well as actin mRNA and amplicon sizes are shown in Table 3.
We next sought to identify BAG-like genes in G. max as these genes are evolutionarily conserved and have been associated with diverse physiological processes in animals, including apoptosis, stress responses, and the cell cycle [21]. A BAG protein family has to date only been reported in Arabidopsis and characterized by profile sequence (Pfam) and profile–profile (FFAS) algorithms [21]. Although BAG proteins are extensively studied in animals, very little is known about their function in plants or to what extent functional similarities might exist between the plant and animal systems [40].
By searching the Arabidopsis genome sequence using HMM-based protein search tools (Pfam and SMART), Doukhanina et al. [21] found seven putative AtBAG-like proteins with high statistical significance (E values in the interval 2.7 × 10−06/8.3 × 10−26). Amino acid sequences were annotated as “unknown” or “hypothetical” proteins. The criterion to accept the putative AtBAG-like members was based on the conservation of the critical amino acids in the BAG domain (or BD). We used this same criterion to identify four putative BAG-like protein sequences in soybean (Table 2). These four proteins ranged in length from 179 to 1253 amino acids. When comparing the lengths of these four BAG-like proteins with those of Arabidopsis, it is likely that the longest G. max BAG-like protein is a BAG6-like. Doukhanina et al. [21] also indicated that the domain structure correlates with the predicted cellular localization of Arabidopsis BAG family proteins. AtBAG1, AtBAG2, AtBAG3 and AtBAG4 share very similar domain organizations with a ubiquitin-like domain in the N terminus and are predicted to be cytosolic proteins. In contrast, AtBAG5 and AtBAG6 have very similar domain organization, with the calmodulin-binding (or IQ) motif found upstream the BD.
RT–PCR was performed to examine the expression of the four putative GmBAG-like genes in G. max root tip cells treated with TQ for 20, 40, 60, 80, 100 or 120 min. The expression of only one of these genes, e.g. accession No. XM_003529861 was induced over this time course (Fig. 8). RT–PCR products of the other three mRNAs were recovered for TQ-treated as well as untreated G. max root tip cells and the results indicated constitutive expression of the corresponding genes.
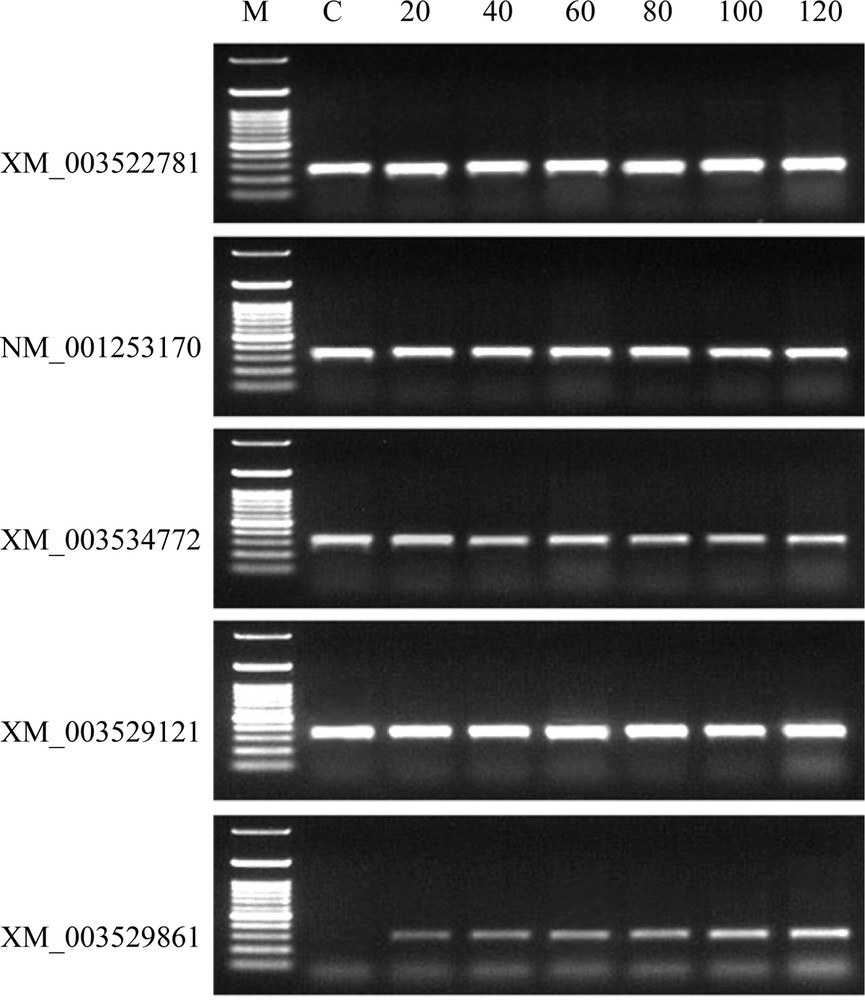
RT–PCR analysis of root mRNAs (accession nos. NM_001253170, XM_003534772, XM_003529121 and XM_003529861) corresponding to the four predicted BAG-like proteins of G. max as affected by treatment with TQ (0.2 mg/mL) for 0 (C), 20, 40, 60, 80, 100 or 120 min. The actin mRNA (accession no. XM_003522781) was used as a positive control. Primer sequences of the four putative BAG-like mRNAs as well as actin mRNA and amplicon sizes are shown in Table 3.
A comparison of the amino acid sequence of the induced GmBAG-like mRNA with the six BAG-like proteins (BAG-1-6) of Arabidopsis indicated that it is most similar to AtBAG6 followed by the AtBAG5 protein (Fig. 9). The sequence of the AtBAG5-like protein (215 aa, Fig. 9) is shorter than that of the soybean BAG-like protein (1253 aa). The length of AtBAG6 is 1043 aa (Fig. 9) and sequence alignment with the soybean BAG-like protein indicated the presence of the two main domains: the IQ CaM-binding motif and the BAG domain (BD) (Fig. 10). Interestingly, the AtBAG6 gene causes cell death in yeast and Arabidopsis plant cells when overexpressed [41]. We observed the AtBAG6 protein shares a previously uncharacterized highly conserved domain of 14 aa (at 451–464) with the soybean BAG6-like protein (at 400–413) (Fig. 10). We, therefore, refer to this gene as GmBAG6.

Multisequence amino acid alignment (a) and dendrogram (b) of the predicted uncharacterized protein corresponding to the putative GmBAG-like mRNA and the six putative BAG-like proteins (BAG-1–6) of Arabidopsis; c: schematic of domains of AtBAG5-like and AtBAG6-like proteins. The dendrogram indicates that GmBAG6 protein is closely related to the AtBAG6 protein. Color online.
Doukhanina et al. [21]
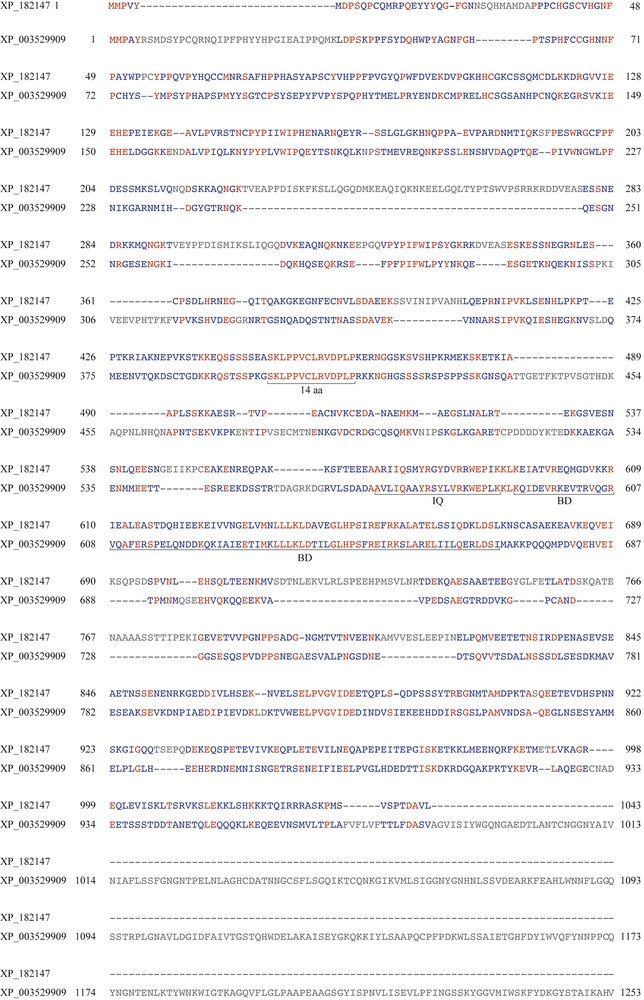
Alignment of the GmBAG6 protein (XP_003529909) with the AtBAG6 protein (NP_182147). BD: BAG domain; IQ: IQ CaM-binding motif; 14 aa, uncharacterized domain with unknown function. Color online.
A BLAST analysis using the GmBAG6 amino acid sequence with the NCBI database as well as databases in the Sol Genomics Network (SGN, http://solgenomics.net/) available for tomato (Solanum lycopersicum) and a wild species of tobacco (Nicotiana benthamiana) against the 14 aa unknown domain revealed substantial sequence identity with 10 other uncharacterized hypothetical plant proteins (Table 4). Interestingly, all of these proteins possess both the IQ CaM-binding and BD domains (Fig. 11). The analysis also revealed that the 14 aa domain is a core sequence in a larger domain consisting of 33 amino acids. These 10 uncharacterized hypothetical proteins belong to Zea mays, Oryza sativa, Medicago truncatula, Ricinus communis, Populus trichocarpa, Solanum lycopersicum, and Nicotiana benthamiana (Fig. 11 and Table 4). These findings further support the notion that the soybean protein we identified is a GmBAG6-like protein as it shares all three conserved domains, i.e. 33 aa conserved region, IQ and BD (Fig. 12). Future research will be directed at understanding whether this 33 aa conserved sequence plays a role in the activity of this protein. Dendrogram of amino acid sequences of these BAG6-like proteins (Fig. 13) indicated high sequence identity among sequences within Zea mays (three accessions) or within Oryza sativa (two accessions). These results indicate that two accessions of Zea mays share the same protein. The same observation applies to two other accessions of Oryza sativa. The results also indicate high sequence identity between BAG6-like proteins of tomato and N. benthamiana. The BAG6-like protein of G. max showed the highest sequence identity with those of Medicago truncatula, Ricinus communis and Populus trichocarpa. Arabidopsis BAG6-like protein showed the lowest sequence identity with those of the other plant species.
BLAST results of the 14 aa domain with unknown function against the NCBI database as well as well as the Sol Genomics Network databases (http://solgenomics.net/) available for tomato (Solanum lycopersicum) and a wild species of tobacco (Nicotiana benthamiana).
Accession no. | Name | Query coverage (%) | E value | Max identity (%) | Size (aa) |
AFW87615 | Hypothetical protein ZEAMMB73_678428 [Zea mays] | 100 | 9 × 10−05 | 100 | 643 |
AFW87616 | Hypothetical protein ZEAMMB73_678428 [Zea mays] | 100 | 9 × 10−05 | 100 | 643 |
AFW71015 | Hypothetical protein ZEAMMB73_290783 [Zea mays] | 100 | 9 × 10−05 | 100 | 1304 |
EEE56689 | Hypothetical protein OsJ_06145 [Oryza sativa Japonica Group] | 100 | 9 × 10−05 | 100 | 881 |
EEC72871 | hypothetical protein OsI_06641 [Oryza sativa Indica Group] | 100 | 9 × 10−05 | 100 | 881 |
XP_003637212 | Hypothetical protein MTR_077s0025 [Medicago truncatula] | 100 | 9 × 10−05 | 100 | 1081 |
XP_002511942 | Hypothetical protein RCOM_1617200 [Ricinus communis] | 100 | 9 × 10−05 | 100 | 1170 |
XP_002301387 | Predicted protein EEE80660 [Populus trichocarpa] | 100 | 9 × 10–05 | 100 | 1227 |
Solyc01g095320 | Hypothetical protein [Solanum lycopersicum] | 100 | 9 × 10−05 | 100 | 972 |
NbS00033349g0010 | Hypothetical protein [N. benthamiana] | 100 | 9 × 10−05 | 100 | 1200 |
XP_182147 | BCL2-associated athanogene 6 [Arabidopsis lyrata subsp. lyrata] | 100 | 9 × 10−05 | 100 | 1043 |
XP_003529909 | PREDICTED: uncharacterized protein LOC100805117 [Glycine max] | 100 | 9 × 10−05 | 100 | 1253 |

Multisequence amino acid alignment of the two BAG6 proteins of Arabidopsis (NP_182147) and G. max (XP_003529909) with proteins identified from a BLAST search of the NCBI database as well as the Sol Genomics Network databases (http://solgenomics.net/) available for tomato (Solanum lycopersicum) and a wild species of tobacco (Nicotiana benthamiana) using the 14 aa domain with unknown function. The highly conserved 33 aa region is shown along with the known IQ and BD regions. Accession Nos. refer to BAG6-like proteins of plant species shown in Table 4. Color online.

Schematic of domains of putative BAG6-like proteins in Arabidopsis (NP_182147) and G. max (XP_003529909). 33 aa, the conserved 33 amino acid region; IQ: CaM-binding domain; BD: BAG domain. Color online.

Dendrogram of the two BAG6 proteins of Arabidopsis (NP_182147) and G. max (XP_003529909) with proteins identified from a BLAST search of the NCBI database as well as the Sol Genomics Network databases (http://solgenomics.net/) available for tomato (Solanum lycopersicum) and a wild species of tobacco (Nicotiana benthamiana). Accession nos. refer to BAG6-like proteins of plant species shown in Table 4.
We conclude that TQ has anti-mitotic activity, cytotoxic activity and the ability to cause apoptotic-like changes on dividing cells of three plant species. This influence was also evident at the gene expression level. The latter analysis demonstrated the association of changes in GmBAG6 gene expression in the response to TQ treatment. G. max was chosen in this work as its roots are large enough to visualize the effect of TQ more easily. To our knowledge, this is the first report of the influence of TQ on dividing cells in plants and it sets the stage for using experimentally tractable plant systems, such as tomato and N. benthamiana, to understand the molecular basis of TQ activity on such cells.
Disclosure of interest
The authors declare that they have no conflicts of interest concerning this article.
Acknowledgments
This project was funded by the Deanship of Scientific Research (DSR), King Abdulaziz University, Jeddah, under Grant no. (4-3-1432/HiCi). The authors, therefore, acknowledge with thanks DSR technical and financial support. The authors also thank authorities at AGERI, Egypt and at KAU for providing technical facilities and support.