1 Introduction
The rhizosphere is a region surrounding a plant's root system in the soil, with high microbial activity affected by the excretions of the plant roots. Microorganisms carry out fundamental processes that contribute to nutrient cycling, plant growth, and root health [1,2]. Soil microorganisms that can stimulate the growth of plants operate through a wide variety of mechanisms, including N2 fixation, enhanced phosphate solubilization, phytohormone production and polysaccharide production [3–5]. Among these bacteria, Pseudomonas spp., ubiquitous inhabitants of soil, water and plants, are in a unique position in the rhizosphere where complex trophic interactions arise. These beneficial or harmful bacteria effects on plant productivity [6,7] are the root group colonizing the most effective of soil microorganisms [8,9]. PGPR effects of Pseudomonas allowed the solubilization of phosphate and maintain the soil's health and quality [10,11]. Indeed, the phosphate-solubilizing bacteria were correlated with the ability of microbes to produce selected organic acids and/or extracellular polysaccharides [12,13].
These bacteria are known for their capacity to produce a wide variety of metabolites [14], nutritional diversity, and rhizosphere competition. The nutritional diversity of Pseudomonas and the control of its membrane fluidity confer a phenotypic plasticity allowing a better adaptation to the hostile environmental conditions [15–17]. Members of genus Pseudomonas have frequently been reported to produce exo-polymers and are considered to be primary colonizers of surfaces in aqueous environments [18]. These complex carbohydrates are widely varied in structure, with interesting physicochemical and rheological properties allowing specific functionality, namely water retention ability and film-forming capacity. Polysaccharides are used in a large number of industrial applications in the food, textile, painting, cosmetic, paper, wastewater treatment and pharmaceuticals as emulsifiers, stabilizers, or thickening agents [19–21]. The composition and biosynthesis of EPS are functions of a variety of environmental factors [22,23] such as climate, soil, topography, decomposers, and seasons [24]. These factors and specific culture conditions can dramatically impact exopolysaccharide production in terms of EPS yield, as well as the size and chemical composition of the polysaccharides being formed [25,26]. The physiological role of these exopolysaccharides is diverse and may be dependent on the specific natural environment of the organism [27,28].
The aim of the present work was the characterization of EPS synthesized by strain TF7 recovered from rhizospheric soil samples from an arid region of the southern Algerian desert (Hassi R’mel), which was subject to the constraints of erratic rainfall and large temperature variations causing high evapotranspiration and the evaluation of its capacity to solubilize phosphate in order to determine the possible synergistic effects of its EPS and organic acid on tricalcium phosphate (TCP) solubilization.
2 Materials and methods
2.1 Isolation and purification of strain TF7
Rhizospheric soil samples of wheat (Triticum durum L.) were collected in Hassi R’mel in Algeria (32°55′0″ N, 03°16′0″) E. This region is characterized by arid climate and a lack in the agricultural sector because of the scarcity of fertile soil. The soil sample was air dried and passed through a 2-mm sieve and then put into glass. Isolation of fluorescent Pseudomonas bacteria from rhizospheric soil was carried out after 4 weeks and soil suspensions were obtained by shaking 10 g of soil sample having roots with tightly adhering soil in 90 mL of 0.1 M MgSO4- 7 H2O buffer for 10 min at 180 rpm. The resulting suspensions were serially diluted and a 0.1-mL aliquot of each dilution was spread onto King's medium (KB) agar. After incubation at 28 °C for 2 days, fluorescent Pseudomonas colonies were located under UV light (366 nm). Single colonies were further streaked onto KB agar to obtain pure cultures.
2.2 Physiological characterization of strain TF7
To identify the strain, an isolate showing obvious similarities in colony morphology and pigmentation was picked from King’B agar plates, incubated at 28 °C for 48 h and subjected to the following tests for the preliminary selection of Gram-negative: Gram reaction was performed as described by Gerhardt et al. [29]. Oxidase and catalase tests were performed using the method of McFaddin [30]; for these tests, a 24-h old bacterial culture was used and results were noted after 18 h at 30 °C.
The presence of arginine dihydrolase and levan sucrase was tested on Thornley's medium and NA supplemented with arginine and sucrose (20 g·L−1), respectively [29]. In order to identify the fluorescence of the strain, overnight-grown cells on KB agar medium were visualized under UV light (366 nm). Briefly, a visible amount of overnight grown cells from agar plate was smeared (1–2 cm2 area) onto a glass slide containing a loop full (3 mm) of a 3% aqueous KOH solution. A Gram-negative strain was identified as a viscous gel that strings out along with the loop.
2.3 Phosphate-solubilizing activity
Phosphate-solubilizing activity was determined on Pikovskaya [31] which contain (per liter): 0.5 g of yeast extract, 10 g of dextrose, 5 g of Ca3(PO4)2, 0.5 g of (NH4)2SO4, 0.2 g of KCl, 0.1 g of MgSO4·7H2O, 0.0001 g of MnSO4·H2O, 0.0001 g of FeSO4·7H2O and 15 g of agar. After 3 days at 27 °C, a strain-induced halo around the colonies was considered as the indicator of phosphate-solubilizing activity. The solubilization index indicates the ability to solubilize the insoluble phosphate and it is calculated by the formula by measuring the halo diameter (HD) and the colony diameter (CD), to obtain the relation of HD/CD*100 [32]. After 3–4 days, solubilization Index (SI) was measured using the formula [33].
For the purpose of assessing the possible synergistic effects of polysaccharide production and organic acid on TCP solubilization, one gram of purified EPS of TF7 strain was added to 100 mL of NBRIP medium containing 19.2 mg of citric acid, as described by Goenadi et al. [34].
2.4 Genotypic characterization (16S rRNA gene sequencing)
After extraction of the total DNA of the bacteria as described by Sunish Kumar et al. [35], we amplified by polymerase chain reaction (PCR) 16S RNA using specific primers. The reagent mixture was prepared with the GC 518R, 1U of Taq polymerase enzyme and 1X reagent buffer (Promega®) and incubated in a Hybaid PCR Express thermocycler (Thermohybaid, California, USA). The amplification conditions of the PCR were as indicated by Weisburg et al. [36]. PCR products were observed by electrophoresis in agarose gels. The bands of almost 1500 bp were excised from the gels and purified using JET QUICK gel extraction spin kit (GENOMED). The purified fragments were sequenced using CEQ DTCS kit (Beckman Coulter, USA) on Beckman Coulter sequencer (CEQ-8800, Beckman Coulter Inc., Fullerton, USA). Sequence comparisons were performed using BLAST algorithm at the National Centre for Biotechnology Information (http://blast.ncbi.nlm.nih.gov/). The obtained sequences were registered in NCBI Genbank (www.ncbi.nlm.nih.gov/Genbank/submit.html) under accession number KC906191.
2.5 Production and purification of EPS
The strain TF7 forms mucoid and brilliant colonies on solid Levan medium, which contain: yeast extract (2 g·L−1); bactopeptone (5 g·L−1); sucrose (20 g·L−1); sodium chloride (5 g·L−1); and agar (15 g·L−1). Mucoid colonies were scraped with a sterilized spatula, and suspended in distilled water. After homogenization, the bacterial mash was taken out for a night and centrifuged (6000 g for 15 min at 4 °C). The bacteria-free supernatant containing the EPS fraction was filtered under vacuum sequentially through membranes of 8.0, 3.0, 1.2 and 0.80 μm pore size to eliminate cells and large cellular fragments. The high-molecular-weight fraction of EPS was precipitated by addition of three volumes of ethanol. The EPS precipitate was ultrafiltrated through a membrane of 100 kDa and then lyophilized.
2.6 Structural analysis of EPS
The samples were dissolved in D2O. Nuclear Magnetic Resonance (NMR) spectra were recorded with an Avance III 400 MHz Bruker spectrometer. 1H NMR spectra were obtained at 298 K with a spectral width of 2000 Hz and a pulse length of 12.7 μs (90°). The scans were accumulated with an acquisition time of 2 s. Data were zero filled to 32 K. 13C NMR spectra were obtained at 358 K, in order to decrease the viscosity of the exopolysaccharide solution, with a spectral width of 15,000 Hz and a recycle delay of 0.8 s. The data were collected in 16 K data sets and before Fourier transformation, exponential multiplication was applied. 1H NMR chemical shifts are expressed in ppm by reference to the D2O solvent peak, calibrated at 4.8 ppm for the analysis performed at 298 K and at 4.20 ppm for those operated at 358 K.
2.7 Chemical analysis
2.7.1 Hydrolysis of EPS
The polysaccharide of TF7 strain was hydrolyzed in 2 M trifluoroacetic acid (TFA) at 100 °C for 30 minutes and 4 h. One fraction of the hydrolyzed sample was injected and analyzed by High-Performance Anion Exchange Chromatography (Dionex), and another part was analyzed by gas chromatography [37].
2.7.2 Gas-liquid chromatography
A part of the previously hydrolyzed sample was reduced and peracetylated, with myo-inositol as an internal standard. The prepared alditol acetates were separated on an Agilent glass-capillary column SP 2380 chromatograph equipped with a flame ionization detector and high-purity nitrogen as the carrier gas.
2.7.3 High-performance anion exchange chromatography with pulsed amperometric detection (HPAEC-PAD)
The hydrolysate was used for the quantification of the constituent monosaccharides. The separation of the constitutive monosaccharides of the TF7 samples was performed using a Dionex LC system (Dionex ICS 3000) equipped by a CarboPac PA1 column (4 × 250 mm) in combination with a CarboPac guard column (Dionex). Neutral monosaccharides were eluted isocratically using 18 mM sodium hydroxide at a flow rate of 0.7 mL/min. Carbohydrate components were detected by pulsed amperometry.
3 Results
On the basis of colony morphology, the strain TF7 was selected for its resemblance to Pseudomonas. The isolate strain was taxonomically described as Pseudomonas fluorescens on the basis of 16S rRNA gene sequencing and subsequent molecular phylogeny analysis and showed a similarity of 96% with that of P. fluorescens the sequence is registered in NCBI Genbank under accession number KC906191 (Fig. 1).

Phylogenetic analyses of strain TF7 of Pseudomonas fluorescens based on the nucleotide sequence of 16S rRNA. The phylogenetic tree was constructed by neighbor-joining (NJ).
Strain TF7 showed good growth on King's medium B (KB) agar after 48 h. The bacterial strain had proven to be a positive reaction resulting in the formation of a viscous filament after contacting the bacterial cream with a solution of potassium hydroxide (KOH 3%). The physiologic tests used confirm that the strain was Gram-negative, motile, and tested positive for cytochrome oxidase, catalase, and arginine dihydrolase (Table 1). The TF7 strain had a capacity to produce a variety of polymers, which was analyzed as levan. Thus, convex and whitish colonies (Fig. 2) were observed after 5 days with a mucoid slimy appearance, indicating polysaccharide production. This production was abundant after 7 days.
Physiological and biochemical characteristics of the bacterial isolate TF7.
Characteristics | TF7 strain |
KOH Test | Positive |
Catalase | Positive |
Oxidase | Positive |
Arginine dihydrolase | Positive |
Polysaccharide production | Positive |
Pigmentation | Green |
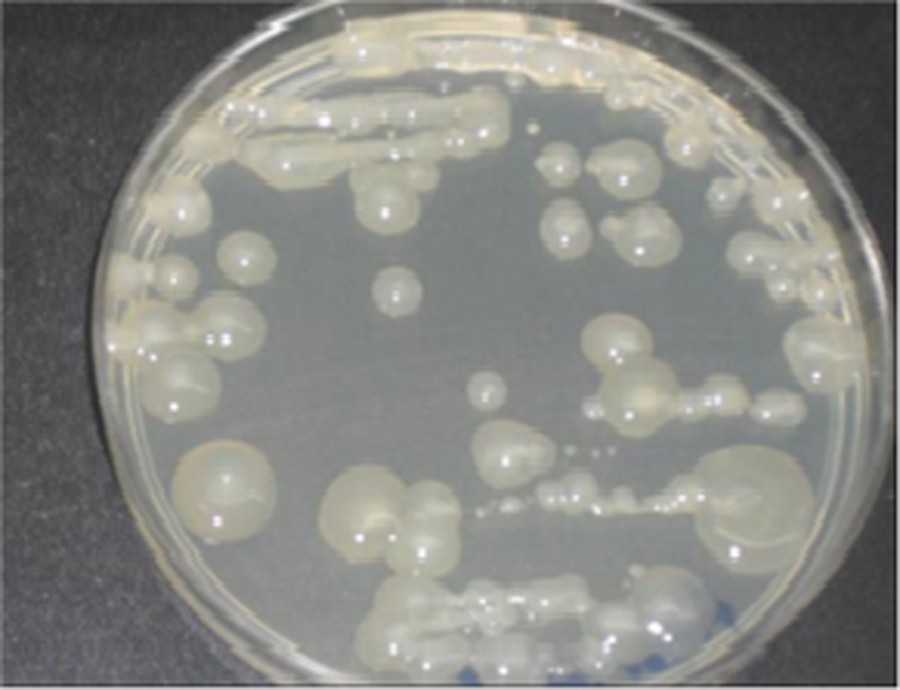
(Color online.) Polysaccharide production on King B medium by strain TF7.
The TF7 strain produces 4.5 g per liter of the polysaccharide dry weight when it was cultivated on sucrose medium against 3.7 for the strain isolated from sub-humid regions.
The isolated TF7 is capable of solubilizing tricalcium phosphate present in Pikovskaya medium using glucose as the sole carbon source and forming large halos of solubilization after 48 h of incubation, to be cleared after 72 h. The composition of the medium affects the polysaccharide production and phosphate solubilizing activity [38] as well as the type of soil [39], and the location from which the bacteria were isolated [40].
The performance of strain P. fluorescens to solubilize phosphate (phosphate-solubilizing index 2.33) was better than those of P. putida [41] and P. stutzeri [42], known as the best known phosphate solubilizers. Jeon et al. [43] reported phosphorous solubilization by three strains of P. fluorescens.
The relationship between phosphate solubilization and pH indicates that acidic conditions observed by the decrease in pH would play a role in the solubilization of phosphate (data not shown) [44,45] (Fig. 3).
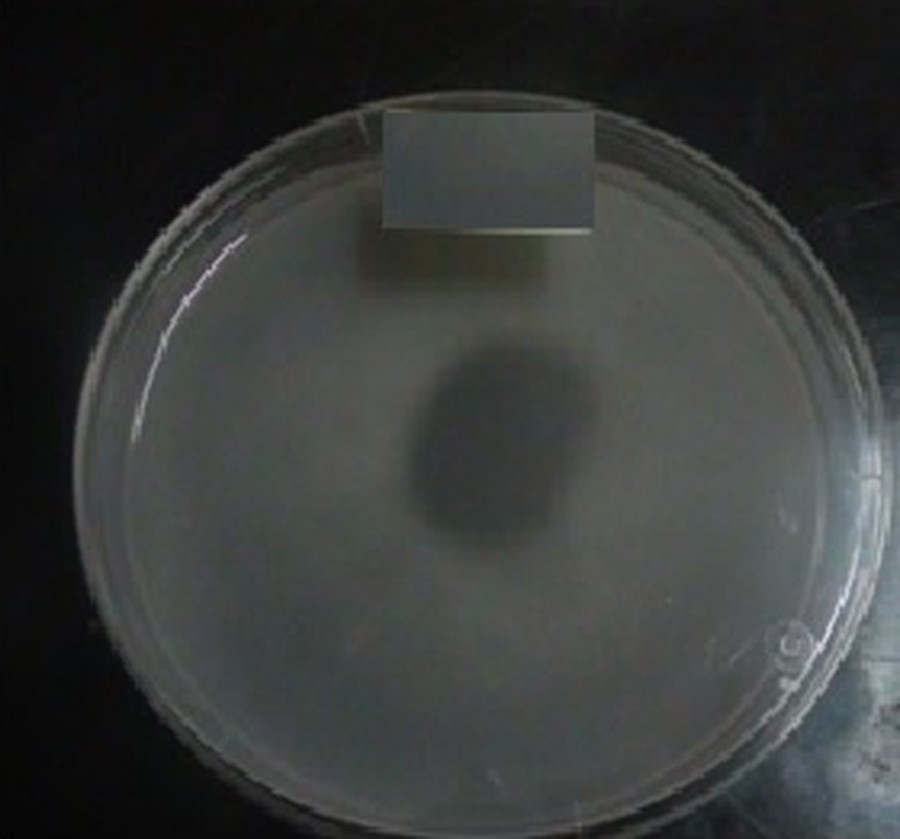
(Color online.) Phosphate solubilization on Pikovskaya's agar medium by strain TF7.
1H and 13C nuclear magnetic resonance (NMR) spectroscopy were used to evaluate EPS structure. The 1H NMR spectrum contained signals between 3.5 and 4.3 ppm, so no signal in the anomeric proton was found (Fig. 4).

1H NMR spectra of the TF7 polysaccharides of Pseudomonas fluorescens.
The chemical shift values generated by the strain TF7 polysaccharide were quite similar to those of levan, with a signal at 104.70 ppm assigned to the C2 of a β-hexofuranose residue, at 80.8 ppm for the C5, 77.6 ppm for the C3, 76.2 ppm for the C4, 64 ppm for the C6, as already described by Han and Clarke [46].
Therefore, our results suggest that the state of the structure of the polysaccharide generated by strain TF7 would probably be that of the levan characterized by β (2→6) linkage (Fig. 5).
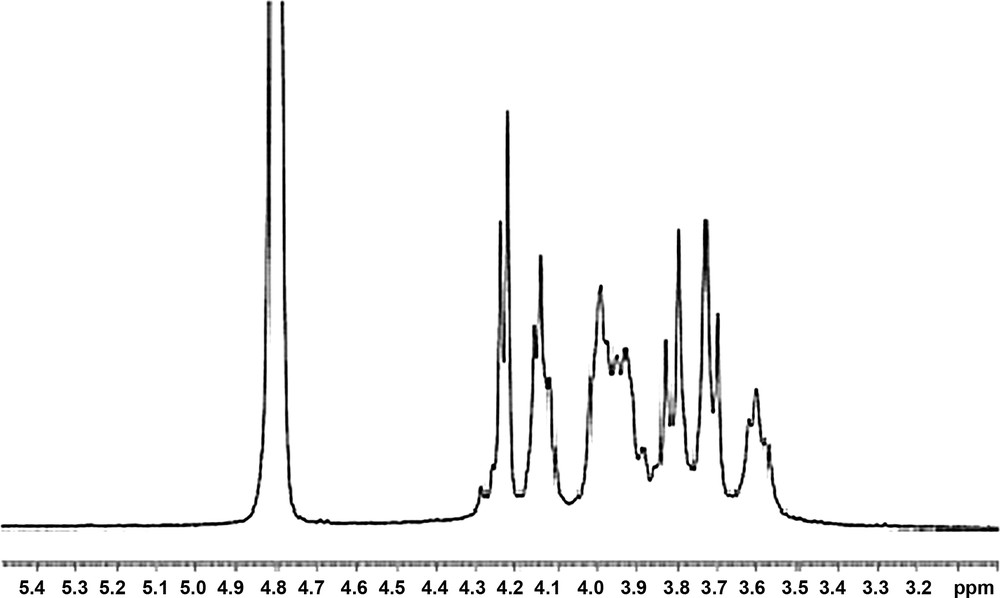
13C NMR spectrum of the TF7 polysaccharide of Pseudomonas fluorescens.
Chemical characterization of EPS produced by the strain TF7 from the sucrose carbon source was performed by gas chromatography and high-performance anion exchange chromatography (HPAEC–PAD).
After 30 min of acid hydrolysis, a chromatography analysis of the monosaccharide composition of EPS revealed that it was mainly constituted of fructose, with the presence of the two epimers of fructose, namely mannose and glucose in a molar ratio of 4:1:0.6, respectively (Fig. 6).
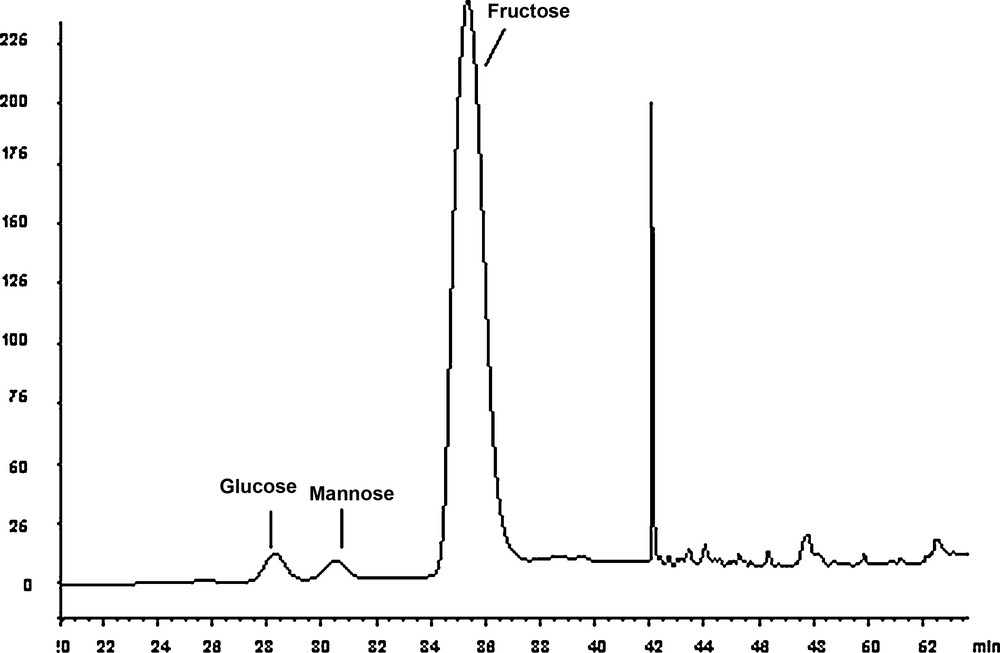
Chromatogram of the degradation of the EPS produced by the strain TF7 after 30 min of acid hydrolysis. The released monosaccharides were separated on a CarboPac PA1 column with 18 mM NaOH as an eluent. Fructose was the main monosaccharide observed, with the formation of its two epimers: glucose and mannose.
The result was confirmed by gas chromatography that the alditol acetates showed the total fructose epimerization in mannose and glucose after 4 hours of hydrolysis (Fig. 7).
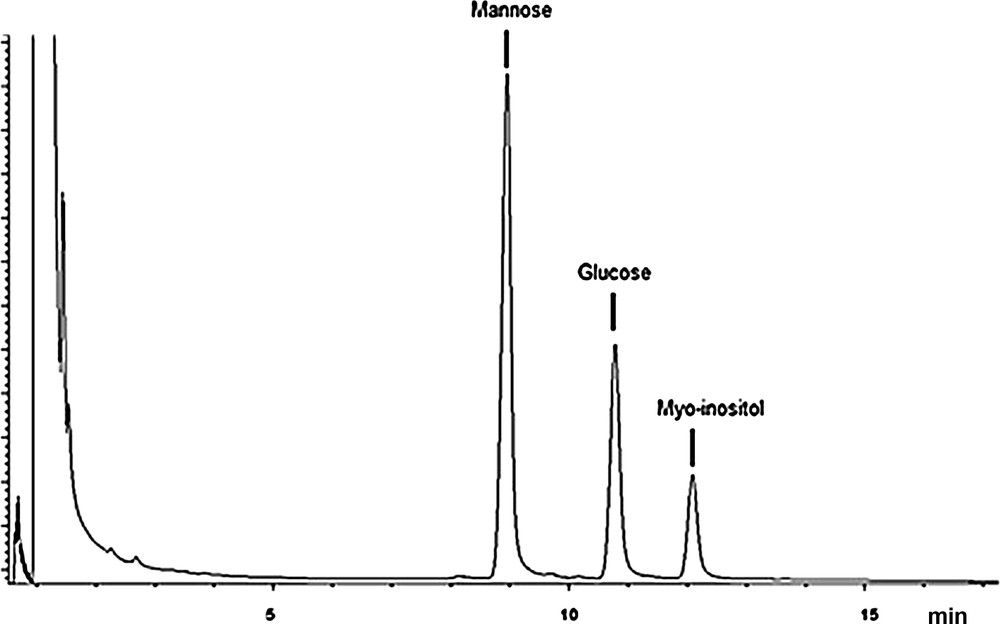
Chromatogram corresponding to the TF7 EPS, after 4 h of hydrolysis, reduction and peracetylation: the corresponding alditol acetates were separated on a SP2380 column with nitrogen as the carrier gas and were detected with a flame ionization detector.
4 Discussion
Arid areas characterized by their extreme bioclimatic conditions and poverty of their soils allow the implantation of only the microflora able to adapt to the hostile conditions, with the example of Pseudomonas, whose synthesis of polysaccharides confers it a greater adaptability.
The strain TF7 isolated from the Algerian desert had oxidase, catalase, arginine dihydrolase activities, and sequencing analysis identified it as P. fluorescens.
On sucrose medium, whitish convex colonies observed after 5 days indicated that bacteria produced the levan sucrase enzyme [29]. The polysaccharide produced by TF7 is a homopolysaccharide consisting of fructose. After acid hydrolysis, the fructose epimers, i.e. mannose and glucose, were present in appreciable amounts. Our results indicated that the structure of the polysaccharide generated by our strain is primarily levan, characterized by β (2→6) linkage.
Pseudomonas is among a wide variety of microorganisms known for their ability to synthesize fructan [37]. It is commonly accepted that fructans produced by microorganisms are levans of high-molecular-weight compared with those produced by plants, and their role in microbial cells’ protection against abiotic and biotic stresses was demonstrated, such as desiccation, freezing, antibiotics or toxic compounds, and attacks of parasites and predators [47,48]. Microbial polysaccharides provide a potential new source of functional biopolymers for food, industrial, cosmetic or medical applications. Depending on the carbon source used, they can be homopolymers or heteropolymers [49].
A variety of EPS of different chemical compositions has been reported in P. fluorescens, so there is a specificity related to the strain. Read and Costerton [50] showed that the EPS of P. fluorescens was composed of glucose, galactose and pyruvate, while Kives et al. [18] showed that the P. fluorescens strain B52 produced polysaccharides containing rhamnose, glucose, and glucosamine. Unlike these results, Chin-Chang et al. [51] reported in P. fluorescens biovar II that 70% of polysaccharide consisted of uronic acid. This variability depends mainly on the type of soil and environment of strain, culture conditions and medium composition [52]. The production capacity of EPS is a direct and logical answer to the selective pressures of the environment [53]. Indeed, the production of EPS requires a lot of energy and thus it seems unlikely that a bacterium uses as much energy and substrate without any benefit. EPS producing bacteria appear to have a competitive advantage that allows them to survive in hostile conditions.
The result obtained shows the synergistic effect between EPS and citric acid on the solubilization of phosphate: it is being reaffirmed that phosphate solubilization is involved with the production of organic acids as described by Goldstein [44] and Rashid et al. [45]. However, P-solubilization is a complex phenomenon, which depends on many factors such as nutritional, physiological and growth conditions of the culture.
In relation to our results, it would be interesting to know to what extent and how the monosaccharide composition and the presence of complex sugar may be essential to the survival and metabolic activity of strain TF7 in arid soils. Moreover, it would be important to analyze how TF7 strain may act as a PGPR, which would interact with the wheat rhizosphere by producing secondary metabolites. These molecules present the highest capacity for carrying biological information since they have the greatest potential for structural variability and constitute a direct response to selective pressures and logic of the environment.
PGPRs are an important environmental factor in the development and maintenance of metabolic activities of plants and are able to promote and enhance plant growth through the compounds released into the rhizosphere. This interaction also depends on the plant, which, through its root exudates, changes the environment in the rhizosphere and thus promotes the development of specific microorganisms.
Acknowledgments
We thank A.-L. Pablo for her technical assistance with PCR and sequencing experiment.