1 Introduction
Arid and semi-arid areas are characterized by a poor soil quality generally (limited nutrient availability), low precipitation, high evapo-transpiration caused by high temperatures. These parameters negatively impact growth, diversity and function of plants [1]. Among these factors, drought stress is one of the most important environmental factors affecting plant growth and yield worldwide [2,3].
In Morocco, the Cupressus atlantica G. is an endemic conifer tree whose geographical distribution is limited in the Western High Atlas Mountains. This region is characterized by repeated episodic drought periods. Together with human overexploitation of natural resources, they negatively impact the regeneration of C. atlantica, thus many revegetation programs have been undertaken using drought-tolerant native plant species in arid and semi-arid regions with a view to reestablishing functional shrublands [4]. Many research investigations using beneficial soil microorganisms, such as mycorrhizal fungi, have been tested to alleviate drought stress. Some of these studies have shown that inoculation with arbuscular mycorrhizal (AM) fungi improved water uptake in many plant species that were subjected to drought stress [5,6]. AM fungi are plant-roots symbionts that enhance plant growth even in harsh environment, such as those submitted to drought stress [7–9]. AM fungal mycelia exploit a larger soil volume than roots, enhancing therefore water uptake and its transport to roots [5,10,11]. This improves physiological, cellular effects and osmotic adjustments in plants [12–14].
Plants submitted to drought stress are seriously affected by secondary damages caused by oxidative stresses. These oxidative damages are consequences of reactive oxygen species (ROS) that are able to rapidly react with a wide range of biomolecules causing therefore irreversible damages leading to cell necrosis and cell death [15,16].
This study aimed to investigate the role of AM fungi on the drought tolerance of C. atlantica, which has been previously shown to respond positively to mycorrhizal inoculation [17]. Thus, we hypothesized that AM fungal inoculation could have a beneficial impact on drought tolerance of C. atlantica. This could potentially lead to develop an efficient conservation strategy of this endemic plant. We proposed to investigate the role of AM fungi under drought and its effect on physiological, mineral nutrition, water relations and antioxidant responses in leaves of C. atlantica. We measured enzyme activities, such as catalase, superoxide dismutase, peroxidase as well as proline and sugar soluble accumulation, as a parameter to assess the effect on AM fungi on C. atlantica under water deficiency in greenhouse trials.
2 Materials and methods
2.1 Experimental design and growing conditions
Soil samples were collected in close proximity to Tetraclinis articulate trees at a depth between 10 and 30 cm. Four samples of 1 kg each were collected around the tree trunk a distance of 1 m. This has been done for ten T. articulate trees totalizing 40 kg of soil. Soil samples were collected in an area located in the N’fis valley (Haut Atlas, Morocco; 8°17′02′′W, 31°54′34′′, 1700 m above sea level) in November 2012.
The experimental setup consisted of two sets. The first set was designed for water stress using four different water regimes as described below, while the second set consisted of AM fungal inoculation as follow:
- • plants inoculated with AM fungi grown in sterilized soil (CA + AMF);
- • non-inoculated plants grown in sterilized soil (CAs);
- • non-inoculated plants grown in non-sterilized soil (CA).
The experiment was performed in pot culture of 1500 mL (20 cm diameter, 20 cm height) containing 1 kg of dried soil, and it has been conducted for ten months. Forty replicates per treatment were set up, resulting in a total of 480 plants (each plant per pot culture).
Pot cultures containing C. atlantica seedlings were watered regularly using distilled water at the following water regimes: 25%, 50%, and 75% of field capacity. The watering control consisted of 90% of field capacity. To determine these stress levels (75%, 50% and 25%) of the field capacity, we weighed pots containing 1 kg of dry soil (P1 = 1 kg dry soil) and these pots were then watered until soil saturation (flow of excess water under gravity). Watered pots were covered with black plastic bag to avoid the evaporation and they were soaked for 48 h in greenhouse. Pots were weighed again in order to determine P2, which was considered as saturated soils. The difference between P2 and P1 (P2–P1) provided the amount of water that was required to saturate the soil, which represents 100% of field capacity. In order to determine the watering volumes for four humidity levels of field capacity (90%, 75%, 50% and 25%), we added, to the series of pots containing dry soil, water volumes corresponding to 0.90 × (P2–P1); 0.75 × (P2–P1); 0.50 × (P2–P1) and 0.25 × (P2–P1) respectively. P3, P4, P5 and P6 weight of the pots at 90%, 75%, 50% and 25% of field capacity, respectively, were then calculated. The experiment was conducted in a greenhouse under natural light. The average day/night temperature was 33/25 °C; the relative humidity (RH) was 55/86%.
2.2 Soil analyses
The soil samples were mixed manually and divided in two subsamples. One subsample was autoclaved at 104 °C for 24 h and the other was kept at room temperature for further analyses.
Chemical and physical analyses of mixed soil samples were performed and they provided the following characteristics: pH (H2O) 6; clay (%) 4.8; fine silt (%) 0.0; coarse silt (%) 1.6; fine sand (%) 28.9; coarse sand (%) 64.7; carbon (%) 3.1; total nitrogen (%) 0.09; Olsen phosphorus 5.2 mg/kg; total phosphorus 121 mg/kg.
2.3 Seedlings preparation and inoculation with AM fungi
Seeds of C. atlantica were immersed in distilled water for 24 h, and kept for germination on wet filter paper in Petri dishes at 20 °C. After one week, the germinated seeds were individually transplanted into pots that were filled with 1 kg of soil (sterilized or not).
The arbuscular mycorrhizal fungal inoculum used in this study was previously isolated and described by Ouahmane et al. [18]. This inoculum consisted of a mixture of Rhizophagus manihotis, R. aggregatus, R. fasciculatus, and Acaulospora sp. Seedlings of C. atlantica were inoculated using 3 g of rhizospheric mycorhized roots of maize. This inoculum provided 100% of mycorrhizal colonization.
After ten months of culture under normal conditions of irrigation, plants of C. atlantica were subjected to four water regimes for a four-month period. T0, T1, T2, T3 and T4 correspond to timelines of 1, 2, 3 and 4 months, respectively, where water regimes were applied.
2.4 Measured parameters
After 10 months of the experimental period, samples from ten C. atlantica plants per treatment were used to assess the effect of water stress on growth and water features of mycorhized plants and non-mycorhized ones. The remaining plants were used for mineral analysis and for the determination of other biochemical parameters.
The response of C. atlantica plants to mycorrhizal inoculation was estimated by determining the root collar diameter, stem diameter, elongation of the aerial part of the plant and biomass production. Dry mass (DM) was measured after drying in an oven at 105 °C for 24 h.
The effect of mycorrhizal inoculation on the mineral content in the C. atlantica was estimated by measurement of P, N, K, Ca2+ and Mg2+ in the aerial parts of the plant.
Total nitrogen was measured by the Kjeldhal method [19]. The effect of mycorrhization on the mineral content in the C. atlantica G. plant was estimated by measurement of P, K, Ca2+ and Mg2+ in the aerial parts of the plant [20]. Total P contents are determined by the colorimetric method and measured by spectrophotometry at 820 nm. The contents of K, Ca2+ and Mg2+ were measured by flame emission spectroscopy.
The water content of shoots (WC) was determined by the difference between the mass of fresh material (FM) and the dry matter (DM), in grams of water per gram of DM (g/g DM). The surface area of Cupressus needles was determined on the shoots for all treatments. The relative water content (RWC) was also measured on the shoots for all treatments using the following equation:
The needles’ water potential (ψh) was measured by the method of the pressure chamber developed by [21].
Proline was measured according to the method of Scholander et al. [22]. Five hundred milligrams of previously grounded needle material were homogenized with 5 mL of 3% (w/v) sulfo-salicylic acid. After centrifugation at 4000 rpm for 10 min, the supernatant was mixed with 2 mL of acid ninhydrin and 2 mL of glacial acetic acid in a test tube for 1 h at 100 °C, and the reaction was stopped by placing the tubes in an ice bath. Four millilitres of toluene were added to the supernatant in order to determine free proline content at 520 nm. The values are given in mg/g FM.
To extract soluble sugars, samples of 100 mg FW were grounded with a mortar and pestle using 4 mL of 80% ethanol in water and centrifuged at 5000 rpm for 10 min. The content of soluble sugars was determined according to the method of Dubois et al. [23]. To 1 mL of supernatant, we added 1 mL of 5% phenol and 5 mL of concentrated sulfuric acid. After stirring in a vortex, the samples were left to cool for 30 min and then the optical density was measured at 485 nm. The levels of soluble sugars were determined by reference to a standard range established with reference glucose solutions.
For enzyme extraction, frozen (–70 °C) fresh needles (0.5 g) were grounded to fine powder in liquid nitrogen with mortar and pestle and then homogenized in 6 mL of 0.1 mol/L potassium phosphate buffer (pH 6.8) containing 100 mg insoluble PVP and 0.1 mM EDTA. The homogenate was centrifuged for 5 min at 6000 g and 4 °C, and the supernatant was immediately used for the following antioxidant enzymes analysis.
Superoxide dismutase activity was determined by measuring its ability to inhibit the photochemical reduction of nitroblue tetrazolium (NBT) according to Stewart and Bewley [24]. The reaction mixture (3 mL) contained 13 mM of methionine, 75 mM of NBT, 100 mM of EDTA, 50 mL of enzyme extract within a 50 mM phosphate buffer (pH 7.8). The reaction was started with 2 mM of riboflavin by exposing the cuvette to a 15-W fluorescent tube for 10 min. The absorbance of the reaction mixture was recorded at 560 nm, and one unit of SOD activity (UA) was defined as the amount of enzyme required to cause 50% inhibition of the NBT photo reduction rate. The results are given as UA/min/mg of protein.
Catalase activity was determined by the H2O2 consumption monitored by spectrophotometry at 240 nm [25]. The decrease in H2O2 was monitored by reading the absorbance at 240 nm immediately when H2O2 was added. The difference in absorbance was divided by the H2O2 molar extinction coefficient (43.6 M/cm) and the enzyme activity was given as
Peroxidase activity was measured by following the change in absorption at 470 nm due to guaiacol oxidation. The activity was assayed for 1 min in a reaction solution (3 mL final volume) containing 20 mM of guaiacol, 10 mM of H2O2, and 0.35 mL of an enzyme extract in a 100 mM potassium phosphate buffer (pH 6.8) [26]. POD was analyzed according to Ros-Barcelo [27].
2.5 Statistical analysis
Data were analyzed with two-way ANOVA, with the SPSS statistical package version 18.0 software, to partition the variance into the main effects. Inoculation with AM fungal inuculum, different levels of drought stress, and duration of water stress, were used as the factors, respectively. Significant differences between factors were calculated at 5%, and significant differences were evaluated with post-hoc LSD test (P ≤ 0.05).
3 Results
3.1 Effect of inoculation and water stress on morphological parameters and nutrients uptake
Mycorrhizal inoculation promoted the growth of plants, independently of the water regime. We observed that under different water stress, mycorhized plants maintained higher growth rates than non-mycorhized plants in both sterile and non-sterile soils. Mycorrhizal inoculation had a significant effect on the height of the shoots of C. atlantica during a four-month period of water stress (Table 1). We note that non-mycorrhizal plants subjected to moderate water regime (50% FC) showed an aerial height changing in a manner similar to that of control plants against the regime of 90% FC. Mycorrhizal plants showed greater heights against the regime of 75% FC than the control T. The root collar diameter is higher in mycorrhizal plants than in control plants (Table 1).
Effect of the water deficit on the height and the collar diameter of seedlings of Cupressus atlantica G. aged ten months and subjected to different treatments (CA + AMF, CAs, CA). T0, T1, T2. T3 and T4 correspond to 0-, 1-, 2-, 3-, and 4-month periods, respectively.
Plant treatment | Time | 25% FC | 50% FC | 75% FC | T |
Elongation of the aerial part (cm) | |||||
CA + AMF | T0 | 39.33 ± 0.55 | 39.60 ± 1.25 | 40.83 ± 0.61 | 38.60 ± 0.75 |
T1 | 39.63 ± 0.73 | 41.03 ± 1.72 | 41.80 ± 0.95 | 39.73 ± 1.25 | |
T2 | 40.07 ± 0.66 | 41.97 ± 0.94 | 42.87 ± 0.28 | 40.83± 1.3 | |
T3 | 40.77 ± 0.30 | 42.47 ± 1.11 | 44.10 ± 0.45 | 42.23 ± 1.27 | |
T4 | 41.33 ± 0.38 | 43.13 ± 1.16 | 45.10 ± 0.43 | 42.90 ± 1.34 | |
CAs | T0 | 16.97 ± 0.51 | 21.37 ± 2.78 | 17.23 ± 0.15 | 22.07 ± 1.12 |
T1 | 17 ± 0.53 | 22.30 ± 2.61 | 17.97 ± 0.46 | 23.03 ± 1.31 | |
T2 | 17.30 ± 0.44 | 23.47 ± 2.15 | 19 ± 0.62 | 23.97 ± 1.44 | |
T3 | 17.40 ± 0.36 | 24.13 ± 1.6 | 19.87 ± 0.9 | 24.43 ± 1.62 | |
T4 | 17.70 ± 0.36 | 24.63 ± 1.3 | 20.73 ± 0.8 | 25.20 ± 1.61 | |
CA | T0 | 22.77 ± 0.6 | 24.87 ± 1.06 | 29 ± 0.46 | 32.20 ± 2.05 |
T1 | 27.87 ± 0.66 | 25.07 ± 1.06 | 29.5 ± 0.8 | 32.97 ± 3.04 | |
T2 | 28.37 ± 0.85 | 25.57 ± 1.8 | 29.87 ± 0.82 | 33.9 ± 2.09 | |
T3 | 28.60 ± 0.82 | 26.13 ± 1.79 | 30.97 ± 0.3 | 35.43 ± 2.02 | |
T4 | 28.93 ± 0.85 | 26.63 ± 1.95 | 31.20 ± 0.5 | 36.30 ± 1.39 | |
Collar diameter (mm) | |||||
CA + AMF | T0 | 5.72 ± 0.45 | 6.72 ± 0.36 | 7.30 ± 0.6 | 7.19 ± 0.77 |
T1 | 5.62 ± 0.27 | 6.46 ± 0.29 | 7.48 ± 0.54 | 7.58 ± 0.59 | |
T2 | 5.54 ± 0.44 | 6.6 ± 0.27 | 7.67 ± 0.59 | 7.58 ± 0.57 | |
T3 | 5.59 ± 0.86 | 6.70 ± 0.30 | 7.78 ± 0.55 | 7.93 ± 0.59 | |
T4 | 5.87 ± 0.72 | 6.97 ± 0.05 | 7.99 ± 0.64 | 8.10 ± 0.59 | |
CAs | T0 | 2.27 ± 0.15 | 4.38 ± 0.31 | 3.74 ± 0.35 | 4.24 ± 0.08 |
T1 | 1.98 ± 0.17 | 4.19 ± 0.24 | 3.85 ± 0.38 | 4.37 ± 0.10 | |
T2 | 1.90 ± 0.16 | 4.29 ± 0.34 | 4.08 ± 0.38 | 4.44 ± 0.11 | |
T3 | 1.88 ± 0.17 | 4.42 ± 0.26 | 4.19 ± 0.40 | 4.61 ± 0.05 | |
T4 | 2.13 ± 0.05 | 4.59 ± 0.18 | 4.39 ± 0.37 | 4.73 ± 0.10 | |
CA | T0 | 3.23 ± 0.20 | 3.83 ± 0.28 | 4.27 ± 0.26 | 4.44 ± 0.07 |
T1 | 3.10 ± 0.32 | 3.73 ± 0.33 | 4.33 ± 0.25 | 4.48 ± 0.07 | |
T2 | 2.85 ± 0.10 | 3.92 ± 0.32 | 4.36 ± 0.24 | 4.50 ± 0.06 | |
T3 | 2.89 ± 0.11 | 4.01 ± 0.31 | 4.42 ± 0.25 | 4.53 ± 0.08 | |
T4 | 3.05 ± 0.10 | 4.13 ± 0.27 | 4.42 ± 0.23 | 4.62 ± 0.11 |
The level of water stress at 25% and 50% FC induced a significant decrease in the collar diameter of inoculated and non-inoculated seedlings of C. atlantica grown in both sterile and non-sterile soils. The highest collar diameters were observed in mycorhized plants.
Overall, water stress reduced significantly shoots, leaves, and collar diameter. However, mycorhized plants were less affected by water stress compared to non-mycorhized plants.
For water regimes 75% and 90%, mycorrhizal plants showed an increase of the root collar diameter compared to non-mycorhized plants. During the four-month water-stress period, plants grown in sterile soils showed reduced length of areal parts and collar diameter compared to mycorhized plants and non-sterile soils.
Interestingly, highly significant effects of mycorrhizal inoculation were observed on shoots and roots dry weights in all treatments (Figs. 1 and 2). The production of shoots dry weight was significantly doubled in mycorhized plants in all water-treatment regimes.
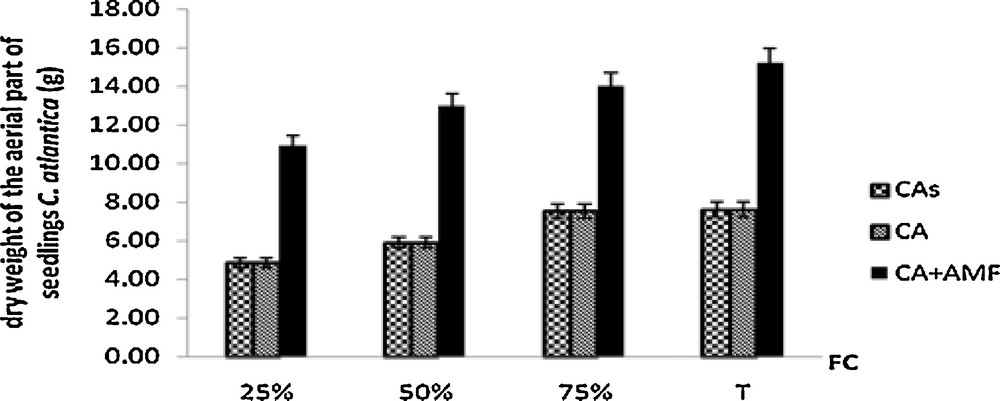
Shoots dry weight of Cupressus atlantica seedlings aged ten months and subjected to different treatments after a 4-month period of water stress.
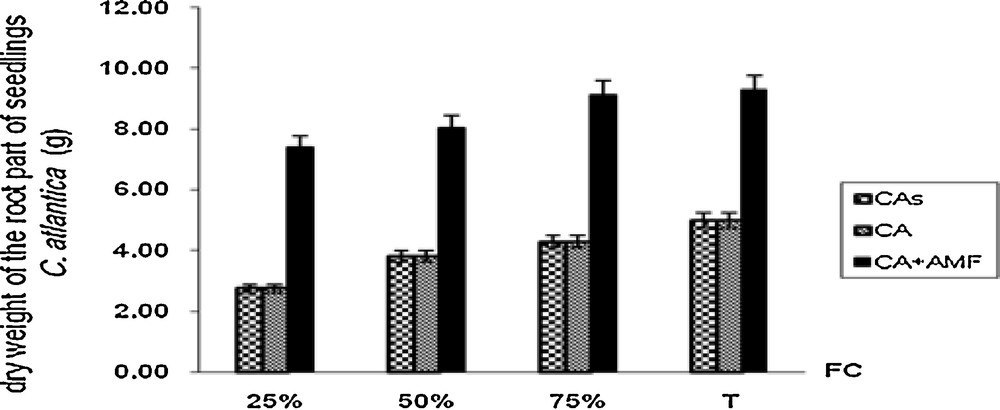
Roots dry weight of Cupressus atlantica seedlings aged ten months and subjected to different treatments after a 4-month period of water stress.
This aerial dry weight mass is two times higher in mycorrhizal plants than in control plants grown on sterilized soil.
Similarly, the root dry mass is clearly significant in mycorrhizal plants. Non-mycorrhizal plants showed low aerial and root dry weights and remained similar whether on soil sterilized or unsterilized. The lowest aerial dry weight was noted in the roots of non-mycorrhizal plants grown in sterilized soil and subject to favorable water regimes (90% and 75% FC).
We observed that the mineral contents of the shoots decreased with increasing soil water deficiency (Table 2). However, we observed that mycorhized plants accumulated higher contents of P, K, N, Ca2+, and Mg2+ than non-mycorhized plants in all water treatments. Overall, this experiment clearly demonstrated that inoculation with mycorrhizal inoculum significantly improved the nutritional status of C. atlantica in our trials.
Effect of the water deficit on the ionic content of shoots of Cupressus atlantica aged ten months and subjected to different treatments after a 4-month period of water stress.
Hydric treatment | Plant treatment | P (mg/plant) | N (mg/plant) | K (mg/plant) | Ca2+ (mg/plant) | Mg2+ (mg/plant) |
25% FC | CA + AMF | 3.45 ± 0.06 | 4.40 ± 0.51 | 5.42 ± 0.06 | 3.52 ± 0.04 | 2.65 ± 0.11 |
CAs | 0.77 ± 0.11 | 2.11 ± 0.54 | 2.09 ± 0.06 | 1.22 ± 0.13 | 1.10 ± 0.66 | |
CA | 1.02 ± 0.42 | 2.26 ± 0.12 | 2.18 ± 0.07 | 1.28 ± 0.08 | 2.23 ± 0.13 | |
50% FC | CA + AMF | 5.52 ± 0.41 | 6.65 ± 0.07 | 9.82 ± 0.07 | 5.12 ± 0.10 | 3.20 ± 0.04 |
CAs | 1.52 ± 0.10 | 2.55 ± 0.49 | 2.26 ± 0.16 | 2.07 ± 0.04 | 1.93 ± 0.05 | |
CA | 1.09 ± 0.34 | 3.40 ± 0.31 | 3.74 ± 0.13 | 2.23 ± 0.07 | 2.43 ± 0.26 | |
75% FC | CA + AMF | 10.99 ± 0.06 | 11.30 ± 0.17 | 12.40 ± 0.49 | 6.70 ± 0.05 | 6.21 ± 0.16 |
CAs | 2.82 ± 0.16 | 2.94 ± 0.07 | 3.64 ± 0.46 | 2.67 ± 0.23 | 1.98 ± 0.04 | |
CA | 1.85 ± 0.61 | 2.68 ± 0.52 | 5.68 ± 1.37 | 4.65 ± 0.27 | 3.45 ± 0.16 | |
T | CA + AMF | 15.07 ± 0.37 | 10.51 ± 0.39 | 14.78 ± 0.19 | 9.05 ± 0.24 | 7.39 ± 0.37 |
CAs | 1.59 ± 0.36 | 2.60 ± 0.17 | 4.70 ± 0.34 | 3.58 ± 0.44 | 2.31 ± 0.18 | |
CA | 1.06 ± 0.12 | 1.30 ± 0.37 | 5.28 ± 0.76 | 5.22 ± 0.06 | 2.18 ± 0.24 |
3.2 Effect of inoculation and water stress on physiological parameters
In general, the non-mycorhized C. atlantica plants were negatively impacted with high water stresses (25% and 50% FC) compared to mycorhized plants (Fig. 3). C. atlantica seedlings mycorhized maintained high relative water content in their shoots compared to control plants. The relative water content decreased when the water deficit increased in needles of non-inoculated plants growing in both sterile and non-sterile soils for 75%, 50% and 25% FC. Fig. 3 shows a significant decrease of the relative water content of non-mycorhized plants grown in sterile soils in the treatment of 25% FC.
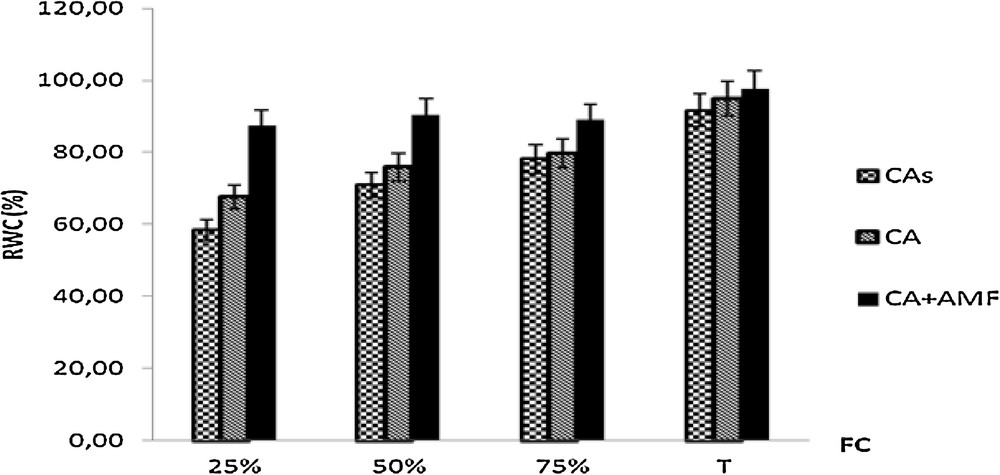
Relative water content of shoots of seedlings of Cupressus atlantica aged ten months and subjected to different treatments after a 4-month period of water stress.
Fig. 4 shows that C. atlantica mycorhized plants have higher water potential in needles between –1.75 and –0.90 bar than the control plants under severe (25% FC) and moderate (50% FC) water stress, while the non-mycorhized and mycorhized plants showed non-significant leaf water potential (1.5 bar) under water regimes of 75% and 90% FC.
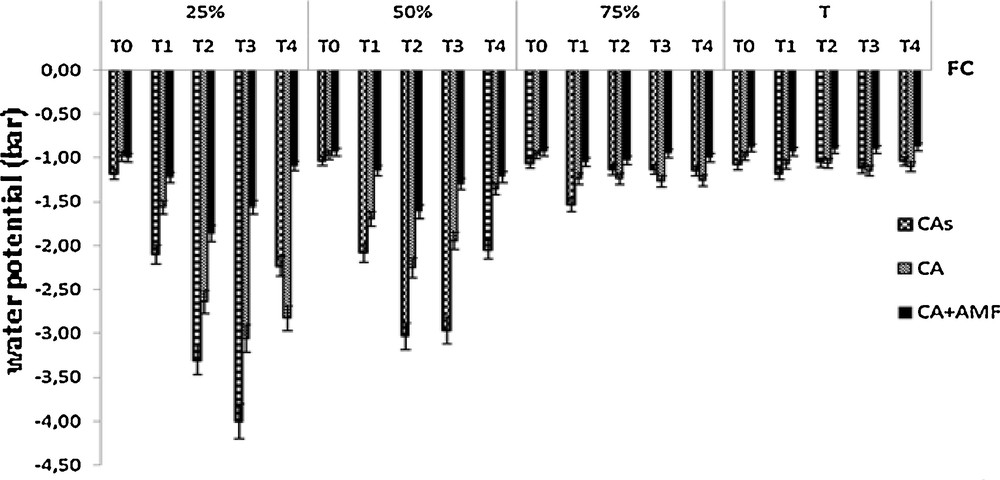
Change in water potential of seedlings Cupressus atlantica of ten months old and subjected to different periods of water stress (T0, T1, T2, T3, and T4).
3.3 Effect of water stress on biochemical parameters
Thus, it appears that the mycorrhizal complex used has a positive impact on morphological and water parameters host plants (Table 1).
The application of high levels of water stress induced a significant increase in proline content in the leaves of mycorhized and non-mycorhized plants (CAs and CA) (Fig. 5). The highest levels of proline were recorded in non-mycorhized plants subjected to 25% FC compared to mycorhized plants. These proline levels increase during the first month of the application of water stress, reaching a maximum level in the second month, and these levels decline slightly over time in the third and fourth months.

Variation of the accumulation of proline of seedlings Cupressus atlantica aged ten months and subjected to different periods of water stress (T0, T1, T2, T3, and T4).
At 50% FC, plants grown in sterile soils showed higher proline content compared to other treatments during the 4-month water stress period, while under water regimes of 75% and 90% FC, the levels of proline remained low and generally similar in mycorrhizal and non-mycorrhizal plants.
Water stress induced an increase in the levels of soluble sugars in the inoculated (CA + AMF) or non-inoculated (CA) and (CAs) young plants tested (Fig. 6). Indeed, as water stress becomes severe, non-mycorhized plants grown in non-sterile soil showed a very high level of soluble sugars compared to mycorhized and non-mycorhized plants grown in sterile soil. Under severe water stress (25% FC), we observed high concentrations of soluble sugars in plants (CA) grown in non-sterile soils. These concentrations have been gradually reduced in the third and fourth months of water stress. A similar pattern was also observed in mycorhized plants. However, concentrations of soluble sugars in plants grown in sterile soils increased over time and reached high levels in the fourth month of water stress.

Variation of the accumulation of soluble sugars of seedlings Cupressus atlantica aged ten months and subjected to different periods of water stress (T0, T1, T2, T3, and T4).
The SOD activity was higher in non-mycorhized plants than in mycorhized plants when water deficit becomes severe, regardless of the period of application of water stress (Fig. 7). At 25% (FC) and in the first month of water stress, this activity reached its higher level in non-mycorhized plants grown in sterile or non-sterile soils. The SOD activity was reduced over time for all treatments. However, the values of SOD activity remain higher in non-inoculated plants than in mycorrhizal plants. At 50% (FC), plants grown in sterile soils showed the highest values of SOD activity compared to other treatments for the 3rd and 4th months of water stress. At 75% and 90% (FC), SOD activity remained low and unchanged.
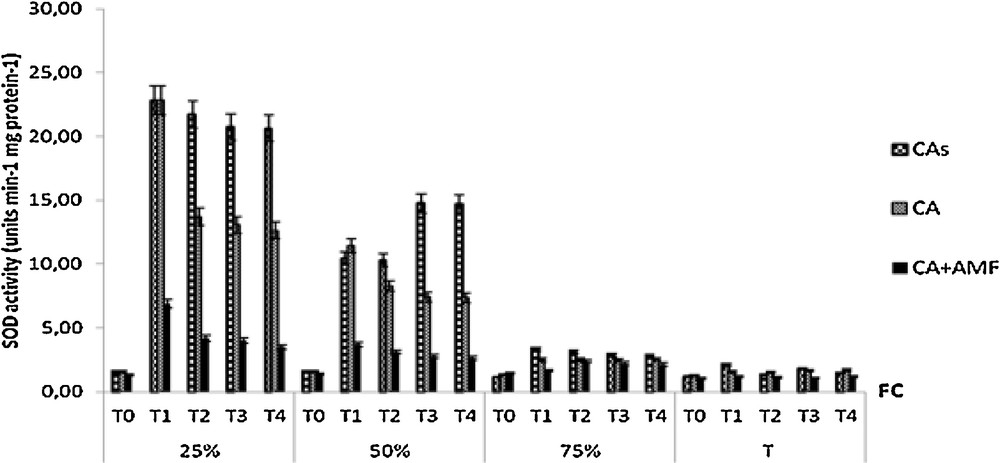
Variation in the activity of SOD of seedlings Cupressus atlantica aged ten months and subjected to different periods of water stress (T0, T1, T2, T3, and T4).
High CAT activity was detected in non-mycorrhizal plants subjected to moderate and severe water regimes (Fig. 8). However, the CAT activity of mycorrhizal plants decreased despite the water stress, which causes an increase in CAT activity after 1 month of stress. During this period, non-mycorrhizal plants grown in non-sterile soil and subject to moderate moisture regime showed the highest CAT activity. This activity is higher in non-inoculated plants compared to inoculated plants for the three water regimes applied (25%, 50% and 75% FC). Over time, we noted a decrease in CAT activity for all plants tested.
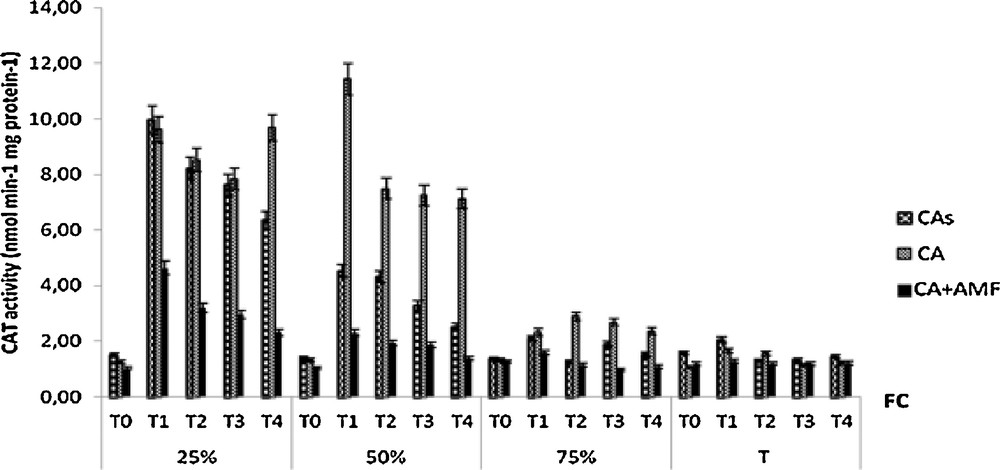
Variation in the activity of CAT of seedlings Cupressus atlantica aged ten months and subjected to different periods of water stress (T0, T1, T2, T3, and T4).
Peroxidase (POD) activity increased significantly when soil water deficiency is increasing regardless of inoculation (Fig. 9). The higher activity was found in mycorrhizal plants.
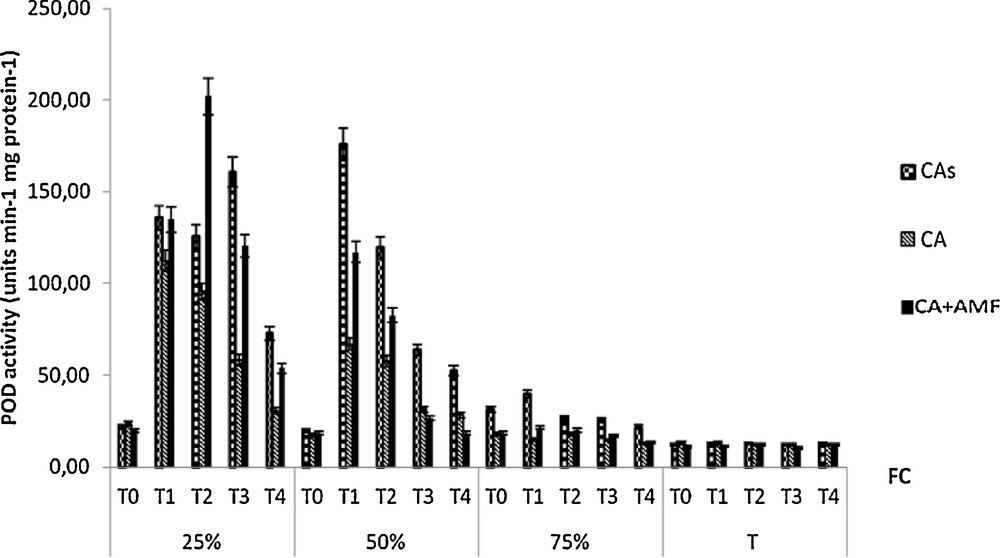
Variation in the activity of POD of seedlings Cupressus atlantica aged ten months and subjected to different periods of water stress (T0, T1, T2, T3, and T4).
4 Discussion
In the present study, morphological, physiological and biochemical aspects related to drought tolerance in mycorhized and non-mycorhized plants under water deficit were investigated. Non-mycorhized plants were more sensitive to changes in soil moisture than mycorhized plants. Mycorrhization by the used inoculum promoted plant growth. Thus, this mycorrhizal inoculation has improved the stem diameter, the root collar diameter and the increase in plant height on the whole water treatment (25% FC, 50% FC, and 75% FC). Similarly, the dry biomass was improved compared to control plants. It is currently accepted that AM symbiosis protects host plants against the detrimental effects of water deficit and that the contribution of AM symbiosis to plant drought tolerance is due to a combination of nutritional, physical, and cellular effects [12]. Generally, AM symbiosis increases host plant growth due to improved plant nutrition [12,28–30] and direct water uptake and transport via external hyphae [10,31]. The beneficial effects of different AM fungi on plant growth under drought conditions have been demonstrated in a wide range of plant species [32]. In this study, the positive effects of AM symbiosis on plant growth were clearly visible. Similar results were reported by Ryan and Ash [33] and Al-Karaki and Al-Raddad [34]. Previous studies show the positive effects of mycorrhiza on plant growth under drought-stress conditions [35–37].
The mycorrhizal inoculum also increased the mineral nutrition of C. atlantica, regardless of the soil regime. Similar results were reported by [38–41]. Moreover, P, N, K+, Ca2+ and Mg2+ in non-mycorhized plants were reduced significantly under drought stress conditions for plants growing in non-sterilized or sterilized soil. Several studies have indicated that AM fungi contribute to plant growth via assimilation of immobile soil nutrients [34]. The improved mineral uptakes could result from the increased absorption area provided by extensive fungal hyphae beyond the roots [9,42–44]. For example, potassium, which is a co-factor for many enzymes, plays an important role in plant metabolism, in relation to the opening and closing of stomata in response to changes in leaf water status [45].
Our results showed that plants inoculated with mycorrhizal inoculum showed a higher RWC and leaf water potential than non-inoculated plants grown in sterile or non-sterile soils under 25% and 50% FC water treatments. However, drought stress decreased the RWC in mycorhized and non-mycorhized plants. Overall, our study showed that AM symbiosis increased RWC and leaf water potential in C. atlantica plant leaves under drought stress.
The relative water content (RWC) is generally used to assess the level of water balance in plants; it is a reliable means by which the state of osmotic stress is measured. Indeed, it allows measuring the state of the water in the plant, reflecting the metabolic state in its tissues. Under drought stress, RWC is generally considered a good indicator of water stress tolerance in a wide variety of plants [46–49]. It was found that mycorrhizal inoculation increased the RWC of the leaves [50,51]. Many mechanisms related to RWC have been reported, such as stomatal regulation through hormonal signals [52]; higher stomatal conductance and transpiration fluxes [53]; indirect effect of improved phosphate and other nutrient uptake [54]; greater osmotic adjustment [55] and/or higher root hydraulic conductivity [32] than in non-mycorhizal plants.
Similar results were also documented in conventional cultivars in ramie under water deficit condition [51,56]. Beltrano and Ronco [57] reported that mycorrhizal colonization of wheat under water stress conditions had beneficial effects on the water status. It was previously reported that RWC of shoots of Juniperus oxycedrus persisted during the progression of drought stress [30]. Preserving higher RWC in mycorrhizal plants suggests a tolerance strategy by reducing the water loss and making the water stress pressure on the plant evade. The ability of mycorrhizal root to improve water absorption under lower soil water potential contributed to maintain RWC under severe drought stress. Similar responses have been reported in the case of several mycorrhizal plants [41,58,59].
Moreover, the physiological responses of symbiosis may vary depending on host plant and fungal species. Nevertheless, the influence of these processes on the leaf water potential in mycorrhizal plants under drought stress is still unclear, despite numerous reports showing positive effects of AM symbiosis on leaf gas exchange. Several studies have shown that under water stress, the water potential of the leaf did not differ between mycorhized plants and non-mycorhized plants [32], while other studies have shown that mycorrhizal plants subjected to water deficit maintain higher water potential than non-mycorrhizal plants [41].
A plant strategy to tolerate drought stress is to enhance and accumulate a high concentration of soluble sugars, proline or other amino acids to regulate the osmotic potential of cells under drought stress [60]. According to our results, the concentrations of soluble sugars in leaves increased during water stress in both mycorhized and non-mycorhized plants. The content of soluble sugars concentrations in leaves in non-mycorhized plants was higher than in mycorhized plants under moderate and severe drought stresses. These results disagree with previous studies [60–62], and they suggest that the accumulation of soluble sugars in mycorhized plants could act as defence mechanism against drought [50] and salt stresses [45,63]. The low accumulation of soluble sugars under different stress conditions in non-mycorhized CA plants found in our study may be explained by the use of sugars by AM fungi for their growth [64].
Proline is an important osmoprotectant osmolyte synthesized by many plants to enhance osmosis and prevent dehydration [45]. The proline – the second osmoregulator used in our study – content in the leaves was significantly lower in mycorhized seedlings than in non-mycorhized seedlings under moderate and severe drought stresses. This result is in agreement with the reports of Wu and Xia [55] and of Manoharan et al. [65], who showed that mycorhized orange and vagiegata, respectively, seedlings accumulated less proline in leaves than non-mycorhized plants under severe water stress conditions.
Severe drought stress may induce oxidative damage caused by the transformation of electrons to molecular oxygen to form ROS [66]. ROS accumulation depends greatly on the balance between ROS production and ROS scavenging [67]. Different pathways in plant metabolism are evolved for ROS removal, including non-enzymatic molecules, such as total phenol, or enzymatic molecules as well as antioxidative enzymes (SOD, CAT, and POD) [68,69]. Severity and duration of stress treatment and also the species and age of the plant cause variations in the activities of the antioxidant enzymes [49].
In this study, mycorhized CA had a less SOD activity in the leaves under several drought stresses. SOD is a major scavenger of superoxide anion radical. This result is in agreement with the reports of [30], where they found the decreases the SOD activity in shoot of the AM J. oxycedrus plants compared to non-mycorhized control plants. A similar pattern was reported by Porcel et al. [70], in the case of soybean mycorhized plants. It was reported that increased SOD activity in mycorrhizal plants is not related to the improvement of their nutritional status, but is the direct effect of AM association in response to the drought treatment undergone by the host plant [71,72].
Severe water stress (25% FC) increased CAT activity in both soil treatments. However, the increase was more pronounced in non-mycorhized plants. The low CAT activity of mycorhized plants in response to severe water stress precludes a direct role of this enzyme in the protection against oxidative damages. Nevertheless, an alternative mode of H2O2 destruction is peroxidase production, which is found throughout the cell and has a much higher affinity for H2O2 than CAT [13]. The difference in such antioxidant activities (high POD activity and low CAT activity) reflected a physiological diversity and an intrinsic osmotic stress tolerance needed by the AM complex, which allows them to adapt and survive oxidative stress produced by severe drought stress (25% FC). This result agrees with the findings of [30] showing high activities of POD in AM J. oxycedrus plants.
POD activity observed in leaves of inoculated can protect the chloroplast, which under stress conditions presents sustained electron flows and is the main producer and target of ROS action [73].
Overall, two possibilities can be suggested to explain the low oxidative damage found in the AMF treated plants:
- • either they suffered less drought stress due to a primary drought avoidance effect by the symbiosis (by direct water uptake by fungal hyphae and transfer to the host plant) that kept plants protected against the generation of ROS;
- • mycorrhizal infection increased the activities of a set of defense enzymes, especially PODs, involved in the elimination of ROS.
5 Conclusion
Evidence showed that drought stress can cause a negative impact on plant growth and development by affecting nutritional and hormonal imbalances. However, the impact of such stress can be alleviated and or minimized by naturally occurring microorganism, such as AM fungi. As shown in our study, AM symbiosis can confer a great degree of tolerance against drought stress in C. atlantica seedlings. The results of our study suggest that the inoculation of C. atlantica with AM fungi increased drought tolerance, which could be explained by an improved water uptake directly by extended extraradical hyphae of AM fungi, nutrient uptake, especially P and higher osmotic adjustments. In summary, our investigation clearly demonstrated that indigenous AM fungi were effective in improving the tolerance of C. atlantica under water stress. One of the implications of this study is the use of this inoculum in the frame of a conservation program aiming at reestablishing and protecting C. atlantica in Morocco.