1 Introduction
A substantial fraction of the freshwater available in both temperate and tropical forests is impounded within phytotelmata (“plant-held waters”), including the fallen parts of plants, plant axils, bamboo internodes, pitcher plants, water-filled tree-holes and, in the Neotropics, tank bromeliads [1–4]. They form a highly discontinuous aquatic meta-habitat for microorganisms, macroinvertebrates (which constitute most of the animal biomass) and small vertebrates. So, phytolelmata are relevant natural microecosystems indicative of environmental effects on community assembly that can be sampled exhaustively and thus provide unbiased records of community-level diversity for studying food webs and the processes underlying them [5]. Incoming detritus (e.g., leaf litter from overhanging trees, dead arthropods, animal feces) constitutes the main source of nutrients for the aquatic communities living there [4–8]. The macroinvertebrate communities are structured by the characteristics of the aquatic compartment (i.e. habitat size and complexity, food resources, presence of a top predator) and the terrestrial matrix (particularly insects with aquatic immatures and terrestrial adults) [4,5,9–17]. Larger habitat sizes (water volume) and nutrient availability and quality, by favoring abundance and species richness, affect the aquatic community structure [5,16–19]. For tank bromeliads, greater complexity, related to the number of leaves forming wells, also favors species richness, so that differences in plant species, morphology, and location play an important role in habitat selection by invertebrate colonizers [20,21].
Phytotelmata are present in forest canopies, particularly in the Neotropics due to the presence of tank bromeliads. Nevertheless, the phytotelmata in rainforest canopies are difficult to study because of their dispersion which is further exacerbated by the difficulty in finding them from the ground and their relative inaccessibility. Indeed, reaching natural phytotelmata on tall tropical trees can be very challenging or even impossible in many cases and the most effective canopy access method permitting hands-on study, the single rope technique (see [22]), is extremely time-consuming. Exceptionally, Yanoviak [23] used this technique to study water-filled tree-holes in Barro Colorado Island, Panama, including 30 of them situated higher than 20 m from the ground (likely some of them in the crowns of trees), and showed that the species richness of the aquatic communities decreased with increasing height.
Hence, to temper the predictable effects of covariance between accessibility, habitat characteristics and invertebrate diversity on colonization patterns and community structure, we aimed to develop a method allowing “artificial phytotelmata” to be placed in forest canopies (see their different shapes in [23,24]). This method can potentially permit the diversity of the aquatic fauna in tropical rainforest canopies, including vectors of animal and human diseases (e.g., hematophagous Diptera that transmit protozoan and arboviral diseases) to be studied. Our approach took the form of a preliminary study conducted in French Guiana where we compared three situations: the artificial phytotelmata were installed in the canopy of a riparian forest (a natural corridor), the canopy of a rainforest, and the crowns of trees alongside a dirt road cutting through that rainforest (forest edge; artificial corridor). Forest edges act as dispersal routes or stepping stones for opportunistic species so that they lead to changes in biotic and abiotic conditions that might favor the emergence of dominant populations (e.g., mosquitoes).
2 Materials and methods
2.1 Scarcity of natural phytotelmata in forest canopies
Phytotelmata in tropical rainforest canopies are relatively rare, something we noted when using different canopy access techniques (i.e. the canopy raft, the canopy sledge, the canopy bubble and the single rope technique; see [22]). For example, we never recorded water-filled tree-holes in any of the 1077 tree crowns inspected in the tropical rainforest canopies of Cameroon, French Guiana, Gabon, Madagascar and Panama; they can be present just below the crown when a main branch has broken off (AD, pers. com).
Concerning tank bromeliads, in French Guiana, we noted the presence of Aechmea mertensii in three of the 167 tree crowns examined in the Paracou forest (1.8%; they were associated with ant gardens) and in one of the 87 tree crowns examined around the Nouragues Research station (1.15%) (AD, pers. com.). Yet, tank bromeliad presence is higher in riparian forests as, for the 306 large trees observed from the vantage point of a pirogue on the Sinnamary River below the Petit Saut dam, 36 (17.47%) obviously bore Aechmea aquilega or A. mertensii in their crowns (AD, pers. com.). A difference in epiphyte density, including tank bromeliads, has already been reported ([25]). Indeed, the proximity to the river (high elevated humidity and incident radiation) may explain why tree species richness and abundance are greater in riparian forests than in the adjacent terra firme rainforests. The taller canopy and the greater tree species richness provide epiphytes with more possibilities to anchor themselves, and the varying exposure to light permits the installation of both shade-tolerant and intolerant epiphyte species [26]. Furthermore, the corridor formed by rivers favors the movement of adult insects whose larvae develop in the phytotelmata of these areas that are thus easily colonized. This is why we placed artificial phytotelmata in the canopy of a riparian forest to be compared with those placed in the canopy of a mainland rainforest.
2.2 Study site and artificial phytotelmata
This survey was conducted in French Guiana (3500 mm of yearly precipitation distributed over 280 days in the area studied; the maximum monthly temperature averages around 33.5 °C and the minimum around 20.3 °C). During the 2013 rainy season, we installed artificial phytotelmata along 1.4 km-long transects in the pristine forest around the field station at Petit Saut, Sinnamary (05°03′N; 52°58′W) at three sites: one along the Sinnamary River ca. 600 m downstream from the Petit Saut dam; another in the rainforest; and the last along the Crique Plomb dirt road cutting through that rainforest.
The artificial phytotelmata, constructed according to the method developed by Srivastava [24], consisted of 150 ml plastic cups at the bottom of which we glued with aquarium safe silicon the bases of three pieces of green plastic sheets simulating the leaves of bromeliads (Fig. 1A and B). The fake leaves form wells that separate the container into compartments, showing a similar habitat complexity as true tank bromeliads, but because the latter are alive, they gather nutrients from their tanks and reject compounds [5,6]. Thus, in terms of their ecological characteristics, these artificial phytotelmata more closely resemble water-filled tree-holes. Rainwater in the Neotropics is acidic [27] and the water in the tree-holes and the tanks of bromeliads remains acidic through the release of organic acids during the decomposition of leaf detritus [16,28–30]; however, there is also carbonic acid from plant respiration in the tanks of bromeliads [5,6,31,32]. Therefore, at the beginning of the experiment, the cups were filled with 100 ml of water (i.e. 90% rainwater, 10% water from bromeliads that was filtered using 150 μm pore membranes to eliminate any aquatic invertebrates) and one decaying leaf (i.e. dry Vismia guianensis leaves previously placed in rainwater for 4 days) was placed in the central well (see also [16,17,24]). Thereafter, these artificial phytotelmata naturally collected rainwater (pH < 5).

A–B. Photographs of the artificial bromeliads. C. The device permitting us to set them in the tree crowns. D. The beginning of the installation of a device.
2.3 Placing the artificial phytotelmata in the tree crowns
Because tree height was ≈35 m, we used a big shot line launcher (a large slingshot mounted onto a sturdy fiberglass pole) and a polypropylene throwline with a weighted bag (350 g) on one end. The slingshot permitted us to place the throwline in the highest fork of large branches on each selected tree and, thanks to its weight, the bag pulls the throwline to the ground on the other side of the fork. Then, we untied the bag and tied a nylon rope to that end of the throwline and pulled on the other end to place the nylon rope in its final position, creating a large loop between the ground and the highest tree fork. This rope can be pulled back and forth, permitting us to raise the artificial phytotelmata into the tree crowns and later to lower them. We placed a line every 100 m along each transect (15 lines per transect).
The devices permitting us to set the artificial phytotelmata in the tree crowns consisted of three plastic bottles cut to size and held together with packaging tape. Due to changes in wind speed and direction in the forest canopy, small rocks were placed in their bases to keep the center of gravity low and overflow holes were drilled 5 cm from the bottom. The ends of a piece of nylon string were passed through holes drilled at the top of each device and were attached to the line (Fig. 1C).
At the beginning of the experiment, three artificial phytotelmata were placed in each device (i.e. one device per line; 15 devices per transect; a total of 135 artificial phytotelmata) that was attached to one line and then moved up into the corresponding tree crown (Fig. 1D). They were placed just below the fork in the branches to limit wind-induced movement. The devices were retrieved after 3, 6 and 9 weeks; each time, we gathered one artificial phytotelm per device to analyze its contents.
2.4 Aquatic invertebrate sampling
We recorded the water volume (WV; mL) by carefully pouring the water from each artificial phytotelm into a graduated cylinder and its contents were preserved in the field in 4% formalin (final concentration). After filtering and decantation, the fine particulate organic matter (FPOM) was dried in an oven and then weighed using a microbalance (1000–0.45 μm in size; mg). Aquatic invertebrates were sorted in the laboratory and preserved in 70% ethanol. They were mostly identified to species or morphospecies and enumerated. Professional taxonomists provided assistance for the identification of the Oligochaeta and other invertebrates (Prof. N. Giani and Dr. A.G.B. Thomas, University of Toulouse, France). The Culicidae and Chironomidae were found both as larvae and pupae; all other insects were only found as larvae. The use and limitations of morphospecies identification for some taxa are the subject of debate; however, this approach, which is a common and often inevitable practice in ecological studies on tropical invertebrates, remains appropriate when local systems are being compared [19,33].
2.5 Abundance-based rarefaction curves and data analyses
Species rarefaction curves were plotted on the data matrices that were pooled per sampling period. To do so, we used EstimateS 9.1.0 software [34] with 100 randomizations of the sampling order without replacement.
Then, the data concerning the artificial phytotelmata (6- and 9-week timetables pooled; see explanation below) were Hellinger-transformed prior to analysis (see equation “13” in [35]) which is a common practice when records contain many zeros [36]. A Principal Component Analysis (PCA) was used to ordinate the samples according to the macroinvertebrate taxa, thus summarizing community variability, and the different types of forests (vegan and ade4 packages in R software [37]).
3 Results
A total of 1808 aquatic macroinvertebrate individuals belonging to 21 taxa were sorted out of the artificial phytotelmata sampled (Table 1). Insects were largely dominant in number and richness while annelids, arachnids and nematodes were also found.
List of the aquatic macroinvertebrate species or morphospecies (hereafter, “taxa”) noted in the three forest habitats compared. Data after 3, 6 and 9 weeks are pooled and taxa are listed alphabetically by phylum, class, order, and family.
Phylum | Class | Order | Family | Morphospecies/species | Rainforest (30 cases) | Dirt road (30 cases) | Ripicolous forest (30 cases) |
Nematoda | Nematoda spp. | 4 | 3 | 5 | |||
Annelida | Clitellata | Oligochaeta | Naididae | Aulophorus superterrenus | 71 | 0 | 243 |
Arthropoda | Arachnida | Trombidiformes | Hydracarina sp.1 | 0 | 0 | 1 | |
Maxillopoda | Copepoda spp. | 19 | 0 | 0 | |||
Insecta | Odonata | Anisoptera sp.1 | 1 | 0 | 0 | ||
Coleoptera | Dysticidae | Copelatus sp.1 | 0 | 1 | 0 | ||
Diptera | Culicidae | Culex pleuristriatus | 0 | 0 | 17 | ||
Culex (Car.) sp.1 | 1 | 0 | 0 | ||||
Toxorhynchites sp.1 | 1 | 0 | 0 | ||||
Wyeomyia argenteorostris | 134 | 50 | 197 | ||||
Wyeomyia compta | 4 | 2 | 511 | ||||
Wyeomyia splendida | 44 | 0 | 0 | ||||
Wyeomyia testei | 0 | 0 | 9 | ||||
Cecidomyiidae | Cecidomyiidae sp.1 | 34 | 8 | 95 | |||
Cecidomyiidae sp.2 | 8 | 6 | 186 | ||||
Cecidomyiidae sp.3 | 0 | 1 | 62 | ||||
Cecidomyiidae sp.4 | 0 | 0 | 6 | ||||
Ceratopogonidae | Ceratopogonidae sp.1 | 1 | 0 | 0 | |||
Chironomidae | Tanytarsus sp.1 | 9 | 0 | 2 | |||
Orthocladiinae spp. | 66 | 0 | 0 | ||||
Tabanidae | Tabanidae sp.1 | 0 | 0 | 5 | |||
Number of taxa | 14 | 7 | 13 |
Rarefied species richness was significantly lower after 3 weeks than after the two other series of sampling (i.e. 6 and 9 weeks) for which the confidence intervals around the mean species richness were overlapping, indicating no significant difference (Fig. 2).
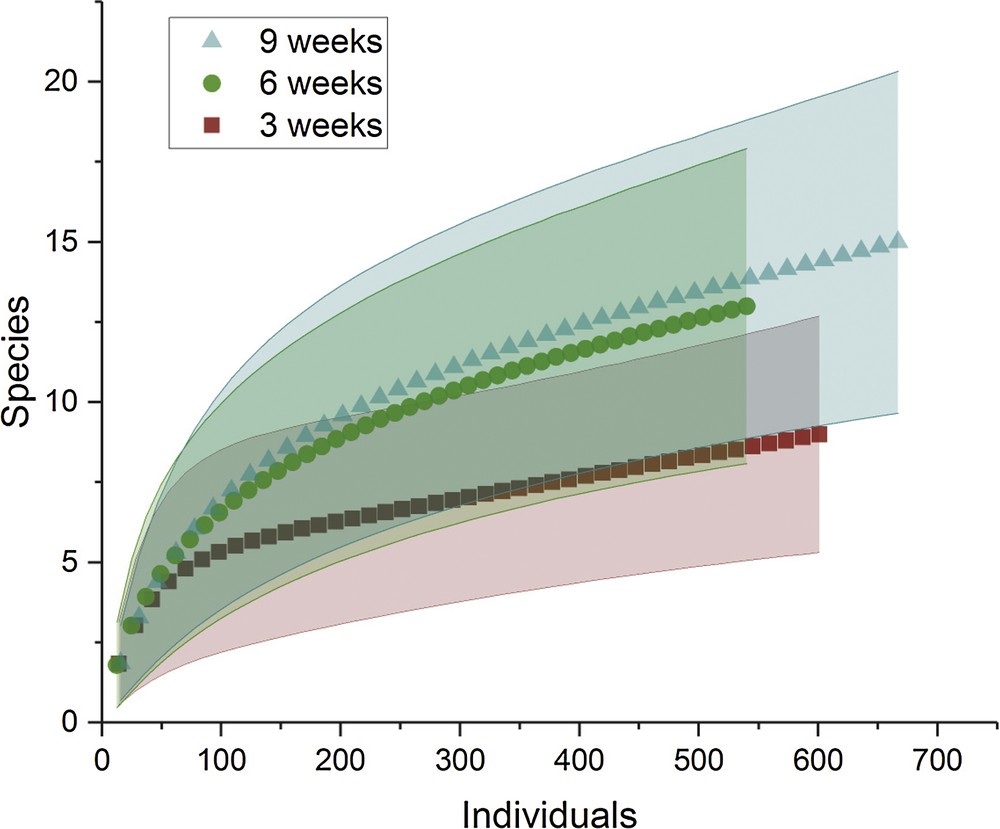
Standardized comparison of species richness (abundance-based species rarefaction curves) between the three temporal series of sampling, all geographical situations pooled. The solid lines represent the 95% confidence intervals.
Because of this result and because most aquatic invertebrate taxa complete the aquatic part of their development in 6 to 9 weeks [8], further comparisons were conducted by pooling the data from these two periods. The sampling conducted after 3 weeks served as an indication of the speed at which the artificial phytotelmata were colonized by aquatic invertebrates.
The clustering of samples by habitat membership in the PCA space suggested a shift in the aquatic community structure between the three habitats compared based on Axes 1, 2 and 3 that represent 30.84%, 20.82% and 14.15% of the variance, respectively (differences mostly visible between Axes 1 and 3; Fig. 3A). Axis 3 clearly separates the aquatic communities of the riparian forest from those in the rainforest, while the communities situated along the dirt road mostly constitute a subset of those in the rainforest (Fig. 3A).

Principal Component Analysis (PCA) ordination of samples (6 and 9 weeks pooled) according to their taxonomic composition. A. Clustering of the samples by habitat membership. B. Correlation between factors (species) and artificial bromeliads (as “individuals”) in the PCA. RI: riparian forest; FO: rainforest; DR: trees situated along the dirt road cutting through the forest. The different taxa represented by short arrows are shown alongside a dashed line.
The PCA space shows that three dipteran species in the aquatic communities are the main taxa characterizing two of the three habitats compared: Wyeomyia compta, Wyeomyia argenteorostris and Cecidomyiidae sp.1 for the riparian forest; and W. argenteorostris, Wyeomyia splendida, and the Orthocladiinae chironomids for the rainforest (Table 1; Fig. 3B). Note that the oligochaete Aulophorus superterrenus was well represented in both cases. However, the taxa noted in the artificial phytotelmata placed in the trees situated along the dirt road cutting through the rainforest corresponded to a subset of those of the rainforest (Fig. 3B).
4 Discussion
4.1 Is using artificial phytotelmata a useful method for gathering pertinent ecological data from forest canopies?
Except for Yanoviak who used the single rope technique [16,17,23], previous studies using artificial phytotelmata in the Neotropics were conducted by placing them on tables in the shade or directly on the ground [24,38,39], whereas our method permits results to be gathered from the forest canopy without needing to climb into the canopy.
The results presented in Fig. 3 show that 6 to 9 weeks are sufficient to note differences in the three habitats compared, illustrating the efficaciousness of the method. Indeed, even taxa that entered into the artificial phytotelmata through phoresy were noted (i.e. Hydracarina, copepods, nematodes and especially annelids; Table 1; [40]). This was corroborated by the fact that a tree frog (Anura: Hylidae), Osteocephalus oophagus, was recorded in one artificial phytotelm. An experiment conducted using artificial containers arranged on the ground in the Costa Rican rainforest showed that, in addition to terrestrial arthropods sheltering in the parts situated above the water level, after 7 weeks, aquatic taxa such as mosquitoes and annelids were also present [39]. Moreover, the color of the containers plays a role in macroinvertebrate abundance and species richness, certain species mostly colonizing black containers [41].
The number of taxa sheltered by our artificial phytotelmata (Table 1) approaches that of the A. mertensii (Bromeliaceae) developing in ant gardens on trees growing in the same area [19]. Note that the latter were situated lower and so were less exposed to the winds and also benefitted from the accumulation of nutrient-rich ant wastes [21]. The main differences were the absence in the artificial phytotelmata of certain phoretic species (mostly annelids), plus scirtid Coleoptera (detritivorous shredders) and predators. Indeed, although they were qualitatively represented, few predators were found in the artificial phytotelmata after 9 weeks. This was particularly true for the mesopredator larvae of the order Diptera (i.e. Corethrellidae, Ceratopogonidae of the genus Bezzia and Chironomidae of the subfamily Tanipodinae that were completely absent). Among the top predators, we noted only one Toxorhynchites (Culicidae), one Anisoptera (Odonata) and one Dysticidae (Coleoptera) (Table 1) whereas they were much more frequent in the tanks of the A. mertensii [19]. So, it is likely that predators only began to occupy these artificial phytotelmata after a period of 6 to 9 weeks.
Because most aquatic invertebrate taxa complete the aquatic part of their development in 6 to 9 weeks [8] and because predators only began to be present in our samples after 6 to 9 weeks, a longer period of time is needed for the artificial phytotelmata to be completely colonized. So, we recommend waiting for a much longer period, for example 12 weeks, for experiments using artificial phytotelmata to permit predators and phoretic taxa to fully occupy the devices.
4.2 Tailoring artificial phytotelmata to different research targets
Although we did not attempt to associate the invertebrates that colonized our artificial phytotelmata with any particular type of phytotelm, it is worth mentioning that the five mosquito species noted in this study are bromeliad specialists [42]. This result may indicate that these artificial phytotelmata can be tailored to different research targets all the while acknowledging that, more than other phytotelm fauna, mosquitoes can occupy multiple phytotelm habitats [2]. In Neotropical rainforests, water-filled holes are rare compared to tank bromeliads (see section 2.1 in the Materials and Methods). For instance, the tank bromeliad A. mertensii was relatively abundant along the dirt road crossing through the rainforest studied (see details in [19]). Indeed, mosquitoes have colonized a great variety of water bodies, including the smallest phytotelmata [1,42,43], and much of their adult biomass is obtained during the aquatic part of their life cycle that has therefore an impact on their fitness [44]. Also, the relative intensity of biological interactions such as predation and competition change with habitat size [42,45,46]. Thus, the size and color of the artificial phytotelmata and/or the number of wells displayed in them might qualitatively and quantitatively influence the mosquito species recorded and likely several other taxa.
Therefore, artificial phytotelmata can be tailored based on their size (the larger, the more species richness and abundance; [16,17,47]), their color (black containers attracted more insects than did green) and their complexity (i.e. the number of leaves and so the presence of wells influencing egg laying by certain mosquito species [48]). In all cases, tree-hole-developing species are more abundant than are bromeliad-developing species [41], see also [48,49].
In conclusion, we show that placing artificial phytotelmata in the crowns of ≈35-m tall trees in a tropical rainforest allowed us to gather information on the types of macroinvertebrates developing therein. We noted different patterns of community diversity in relation to the three areas compared illustrating the feasibility of further studies using this technique with the possibility of varying the size and color of and the number of wells in the artificial phytotelmata. We also estimated that 12 weeks should be long enough for the artificial phytotelmata to be left in the canopy to obtain a good spectrum of all trophic levels plus phoretic taxa. Finally, by tailoring these structures, it is possible to set up experiments favoring certain taxa rather than others, whereas a comprehensive experiment using different artificial phytotelmata, each simulating a type of phytotelm, might permit a wide spectrum of taxa in the forest canopy to be recorded.
Disclosure of interest
The authors declare that they have no competing interest.
Acknowledgments
We are grateful to Andrea Yockey-Dejean for proofreading the manuscript and the Laboratoire Environnement de Petit Saut (French Guiana) for furnishing logistical assistance. This study has benefited from an “Investissement d’Avenir” grant managed by the Agence Nationale de la Recherche (CEBA, ref. ANR-10-LABX-0025).