1 Introduction
Caridean shrimps from the family Alvinocarididae, and especially from the species Rimicaris exoculata Williams and Rona, 1986 〚1〛, dominate the megafauna of most of the Mid-Atlantic Ridge hydrothermal vent fields at depths reaching 3500 m 〚2〛. These shrimps form particularly dense swarms (up to 2500 individuals m–2), hiding the rock, around sulphide black smoker chimneys on tops of hydrothermal mounds 〚2–5〛. The water environment around the aggregations is turbulent, toxic and fluctuating because of mixing of the oxic, cold sea water (1–2 °C) with the hot (±350 °C) and acid (pH 3.0 to 4.0) hydrothermal fluid that is rich in dissolved and particulate metals and inorganic sulphide compounds 〚6, 7〛. The shrimps are regarded as primary consumers having a bacterial diet. They are supposed to graze on the free-living, surface bacterial community and/or to feed off the numerous epibiotic bacteria, regarded by many authors as ectosymbionts, which grow on their mouth parts as well as on the inner surface of their gill chamber 〚4, 5, 8–10〛. As the free-living bacterial mats, this epibiotic bacterial community is dominated by a genetic single type of filamentous ε-proteobacteria 〚11〛 and shows a high sulphur-dependent chemoautotrophic activity 〚9, 12〛. In this view, shrimps appear to be particularly exposed to toxic hydrothermal fluid sulphides that are potential poisons for their aerobic respiration but also essential nutriments for their ectosymbionts. It is thus hypothesised that transport, elimination and/or detoxication processes may occur in the gill chamber of the shrimps, usually solely devoted to ionic or osmotic regulation, exchanges with epibionts and/or protection against toxic substances.
To investigate their possible participation in such exchange and/or detoxication processes and to look for intracellular features potentially related to these processes, the gills of R. exoculata were examined at the ultrastructural level and compared with those of a littoral palaemonid shrimp, Palaemon adspersus.
2 Material and methods
Vent shrimps Rimicaris exoculata were captured in August 1997 at the Rainbow site of the Mid-Atlantic Ridge (N.O. Atalante MARVEL cruise). The gills were dissected, fixed aboard in 2.5% glutaraldehyde in 0.25 M Na-cacodylate buffer (pH 7.4) adjusted at 950 mosm kg–1 with sucrose. They were kept for several weeks at 4 °C in washing buffer. In the laboratory, they were fixed again for 2 h in a buffered 2.5% glutaraldehyde solution and post-fixed for 1 h in 1% OsO4 before embedding in epoxy resin according to a routine procedure (ethanol/epoxypropane dehydration). Palaemon adspersus shrimps were collected in the Mediterranean littoral lagoons close to Montpellier (France). Gills were glutaraldehyde-fixed for 2 h and processed as those of R. exoculata. Ultra-thin sections were performed with a diamond knife on a Reichert–Jung ultra-microtome (Ultracut E), contrasted with uranyl acetate (alcoholic solution) and lead citrate, and observed in a Jeol JEM 100-SX electron microscope at 80 kV of accelerating voltage.
3 Results and discussion
As previously reported 〚1, 8〛, R. exoculata possesses phyllobranchiate gills. In their organisation, they resemble those of P. adspersus and other palaemonid shrimps 〚13–16〛. The gill epithelium mainly consists of flange cells. The large perikaryons of these cells protrude into the haemocoel and make contact with median septum, while the flanges form a thin cytoplasmic sheet at the inner surface of the cuticle (Fig. 1). The gill epithelial cells of R. exoculata however contrast with those of P. adspersus by some ultrastructural features. The number of mitochondria is much higher in the gill epithelial cells of R. exoculata (Figs. 1 and 2) than in those of P. adspersus and other non-vent decapod crustaceans 〚16–19〛. In addition, the mitochondria are distributed exclusively in the perikaryons of the flange cells (Figs. 1 and 2), while they are absent from the flanges where cell organelles are almost completely missing, except for some rough endoplasmic reticulum cisternae (not shown). They also show numerous orderly-arranged cristae and regularly contain a number of small electron-dense granules (diameter of approximately 100 nm) in their stroma (Fig. 3). The cell bodies contain peculiar organelles that regularly appear to be associated with clustered mitochondria (Figs. 1, 2 and 4). These organelles resemble lysosomes by their heterogeneous electron-dense aspect and are single membrane-bounded. Their average diameter ranges from 0.7 to 2.5 μm. Beside a heterogeneous electron-dense matrix, their content is characterised by the presence of several stacks of parallel membranes (Figs. 4 and 5).
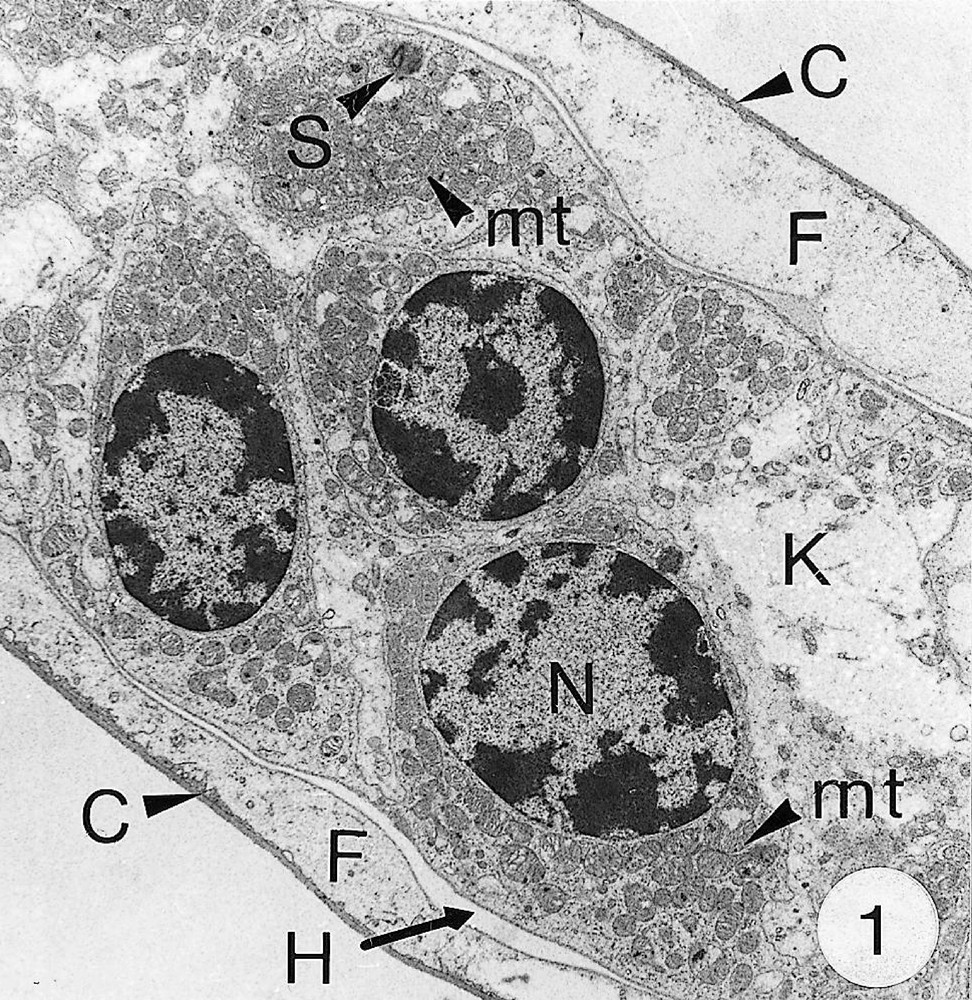
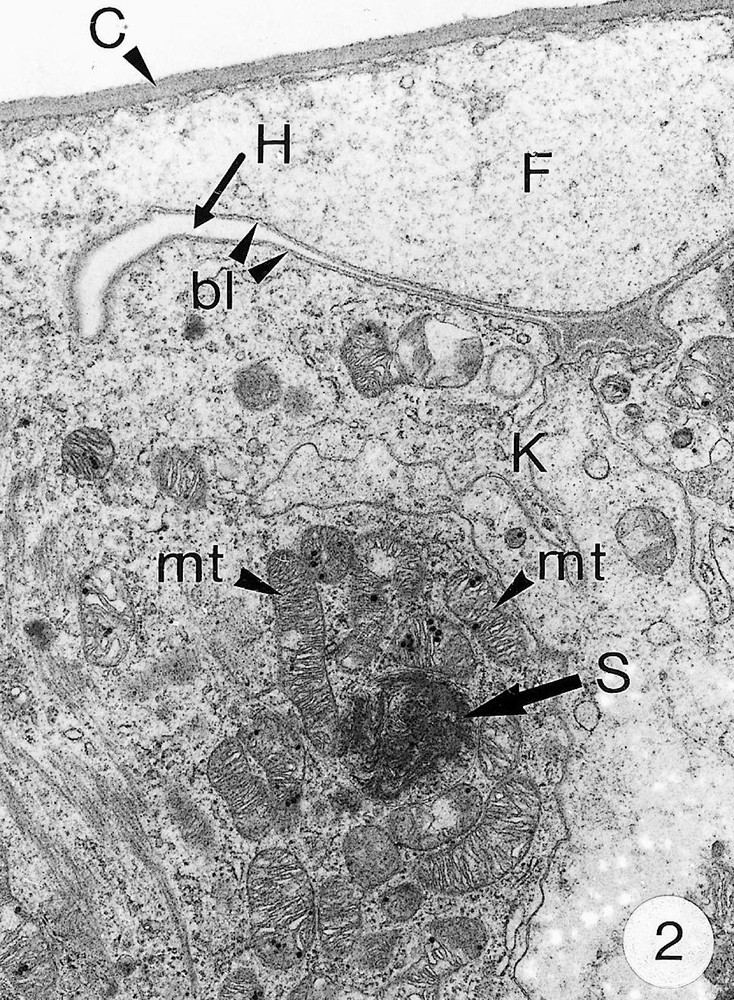
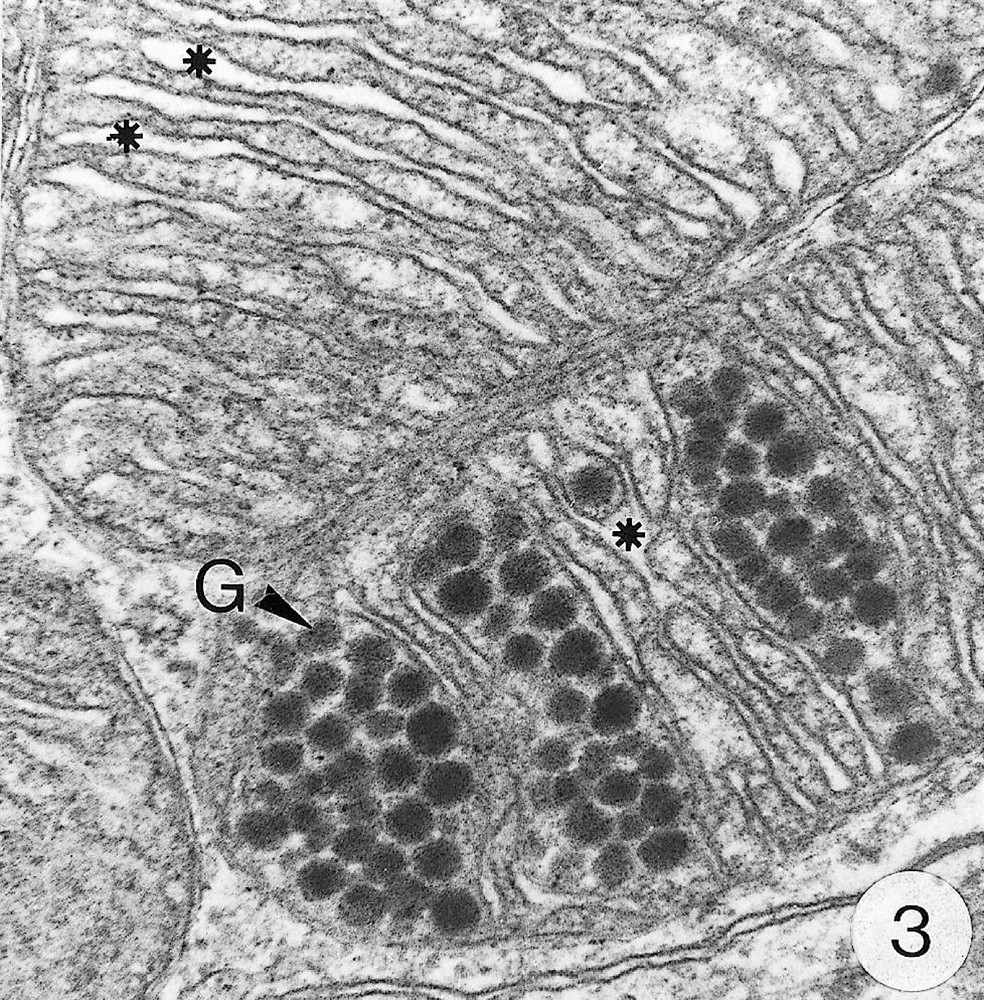


Ultra-thin sections of gill lamellae of R. exoculata. 1. General view of a lamella with flange cells forming the gill epithelium (× 2250). 2. Flange cell showing a cluster of mitochondria (mt) around a sulphide-oxidising body (SOB) (× 8000). 3. Detail of mitochondria (mt) with orderly arranged cristae (asterisk) and containing electron dense granules (g) (× 45 000). 4. Detail of a SOB showing its single bounding membrane (m) (× 30 000). 5. High magnification of a membrane stack in a SOB (× 160 000). bl, basal lamina; C, cuticle; F, flange (cytoplasmic extension); G, electron-dense granules; H, hemolymph; K, perikaryons of flange cells of the gill epithelium; N, nucleus; m, membrane; mt, mitochondria; S, sulphide-oxidising bodies; st, membrane stack; asterisks , mitochondrial cristae.
Arrays of mitochondria and unusual organelles similar to those observed in the gills of R. exoculata were observed in different tissues of thiobiotic and/or sulphide adapted species in which they have been reported to act in sulphide detoxication. Mitochondria displaying numerous ordered cristae and containing small dense granules, have been reported in the gills of the ‘Pompeii worms’ Alvinella pompejana and Paralvinella grasslei 〚20〛 that dwell on active sulphide chimney walls with environmental H2S concentrations up to 300 μmol l–1 〚21〛. Similar characteristic features of mitochondria also occur in the meiobenthic, thiobiotic turbellarian, Solenofilomorpha funilis, a species able to detoxify sulphides, despite the absence of associated or symbiotic bacteria 〚22〛.
Electron-dense organelles displaying membrane stacks were originally described and termed ‘sulphide-oxidising bodies’ (SOBs) in the gill of the symbiont-containing clam Solemya reidi 〚23〛, which was recognised to detoxify sulphide in its tissues and not in its endosymbiotic bacteria. In this species, enzymatic oxidation of sulphide to thiosulphate was demonstrated to occur in mitochondria and SOBs, and to be coupled with ATP production, thus providing additional energy to the organism 〚24, 25〛. Similar SOBs were reported from the hindgut and tegumental tissues of the sulphide-tolerant echiurid worm, Urechis caupo 〚26, 27〛, in which sulphide oxidation to thiosulfate was demonstrated to be performed by crude homogenate of the tissues 〚28〛. The latter authors suggested that U. caupo SOBs could be lysosomal structures that digest damaged mitochondria and accumulate sulphur compounds, perhaps the end products of sulphide oxidation. Some of the R. exoculata SOBs also exhibit lysosomal features (e.g. an heterogeneous content), but the presence of ordered membrane stacks does rather suggest a functional role and remains enigmatic. Cytoplasmic structures resembling SOBs were also described in the gills of Pompeii worms 〚20〛, but the authors have not considered their role.
Thus, the present data suggest that sulphide detoxication may occur in the gill epithelium of the hydrothermal shrimp R. exoculata, since SOBs and mitochondria were regularly observed in the tissues of species adapted to sulphide-rich environments 〚23, 24, 26–28〛. The simultaneous presence and the association of both types of organelles are regarded as a morphological evidence of the occurrence of a sulphide detoxication process. Moreover, the sulphide detoxication mechanism in R. exoculata should be different from that described in the Pacific vent crab Bythograea thermydron that is reported to tolerate at least 1.4 mM sulphide with little effect 〚29〛. In the latter species, such a tolerance is apparently achieved in the digestive gland by oxidising sulphide to relatively non-toxic thiosulphate that is accumulated in the haemolymph and probably eliminated across the gills 〚29, 30〛. The sulphide oxidation activity in the gills of B. thermydron was considered as not significant by these authors. Such a difference in the sulphide oxidation tissue site between B. thermydron and R. exoculata may be explained by behavioural and diet differences between these species. B. thermydron is a top-consumer that can adapt the duration of its stays in a sulphide-rich environment. It can also regulate the water flow through its gills just to compensate its own oxygen demand 〚30〛. In contrast, the shrimp R. exoculata, considered as a primary consumer, stays almost permanently close to the hydrothermal fluid, either to graze on chimneys or most presumably to supply sulphide to the ectosymbiotic bacteria growing on its mouthparts and gill chamber walls 〚4, 5, 10〛. Despite the presence of bacteria that could detoxify the fluid by using sulphide in their chemosynthetic activities, the gills of the shrimp are particularly exposed and continuously bathed by the sulphide-rich water flow passing through the gill chamber. Moreover, it is obvious that the very thin gill cuticle of R. exoculata (less than 0.5 μm thick) cannot act as a barrier against sulphide, which is known to permeate easily through tissues and tegumental structures 〚31〛. Thus, sulphide may easily reach the haemolymph by passing through the cuticle and the flange of the gill epithelial cells. Most of the sulphide detoxication could occur during the transit of the haemolymph in the gill haemocoel in close contact with the epithelial cell bodies, rich in mitochondria and SOBs.
4 Conclusion
The present ultrastructural observations are the first to suggest that the gills of a decapod crustacean can act as sulphide detoxication organs. In addition, the presence of SOBs and mitochondria exhibiting characteristic features in the gill epithelium of R. exoculata strongly suggests that sulphide detoxication could occur at the level of both organelles and might be coupled with ATP production providing energy to the shrimps as it is the case in S. reidi 〚24, 25〛.
These findings exclude neither other physiological functions of the gills of R. exoculata nor the involvement of other tolerance mechanisms to high environmental concentrations of sulphide, since multiple tolerance mechanisms have been simultaneously developed in other species 〚28, 31〛.
On the other hand, the fact that R. exoculata has developed tolerance to sulphide can also be regarded as one more convergent ecophysiological adaptation of these shrimps (which farm bacterial ectosymbionts) with the other vent species housing endosymbiotic or ectosymbiotic bacteria. As previously pointed out by Segonzac et al. 〚32〛 and reviewed by Desbruyères et al. 〚21〛, such an analogy appears particularly close when considering the Pompeii worms that also dwell on active sulphide chimneys. These worms are supposed to be trophically dependent on the bacterial communities they house, and are also supposed to detoxify sulphide in their gills 〚20, 21〛. However, in shrimps and Pompeii worms, characterisation and biochemical evidence for a sulphide oxidation activity and process remain to be provided.
Acknowledgements
This work was partly funded by the EC MAST programme MARVEL, the VENTOX programme EVK3-1999-00056P and the French programme DORSALES. The authors are very grateful to Dr Daniel Soyer (director scientist of the research project in the DORSALES programme) and to Dr Daniel Desbruyères (chief scientist of the MARVEL cruise). The authors wish also to express their appreciation to Mrs N. Decloux (ultramicrotomy) and Mrs C. Breeur (iconography) for their excellent technical assistance.
Version abrégée
Par leur organisation, les branchies de Rimicaris exoculata (Alvinocarididae) ressemblent à celles des crevettes Palaemonidés : ce sont des phyllobranchies limitées par un épithélium de cellules à « collerette » (flange cells). Les « collerettes » de ces cellules bordent la face interne de la cuticule et sont dépourvues d’organites, tandis que les péricaryons, qui contiennent tous les organites cellulaires, font saillie dans l’hémocoele, où ils se rejoignent pour former ensemble un septum médian. Les cellules épithéliales à « collerette » de R. exoculata et celles des crevettes Palaemonidés présentent toutefois un certain nombre de caractéristiques ultrastructurales qui leurs sont propres.
Exclusivement distribuées au niveau des péricaryons, comme chez les Palaemonidés, les mitochondries de R. exoculata apparaissent plus nombreuses. Elles présentent également un plus grand nombre de crêtes régulièrement ordonnées et contiennent souvent des granules denses aux électrons (100 nm de diamètre) dans leur stroma. Mais la différence majeure entre l’épithélium branchial des crevettes littorales (Palaemonidés) et celui de R. exoculata réside dans la présence, chez cette espèce, au niveau des péricaryons, d’organites particuliers, souvent entourés de mitochondries, avec lesquelles ils semblent associés. La taille de ces organites varie de 0,7 à 2,5 μm de diamètre. D’aspect hétérogène et limités par une simple membrane, ces organites ressemblent à des lysosomes, mais s’en distinguent par la présence d’un ou de plusieurs empilements réguliers de membranes, qui occupent la plus grande partie de leur volume.
Les caractéristiques structurales des mitochondries de l’épithélium branchial de R. exoculata correspondent à celles que l’on rencontre dans les branchies d’annélides polychètes (Alvinella pompejana et Paralvinella grasslei) vivant dans les parois de cheminées hydrothermales, un environnement où la concentration en sulfures dissous peut atteindre 300 μmol l–1, ou encore chez une espèce de turbellarié du méiobenthos, Solenofilomorpha funiculis, connue pour sa capacité à détoxiquer les sulfures. Par ailleurs, la présence d’organites cellulaires à empilements membranaires, associés aux mitochondries, a été observée à plusieurs reprises dans les tissus d’espèces adaptées à des environnements riches en sulfures. Elle est considérée comme un indice morphologique de l’existence d’un mécanisme de détoxication des sulfures. C’est ainsi que des organites identiques à ceux observés chez R. exoculata sont bien connus chez le bivalve Solemya reidi, dont l’épithélium branchial héberge des bactéries endosymbiotiques. Il a été montré chez cette espèce que l’oxydation des sulfures en thiosulfates ne s’effectuait pas dans les bactéries symbiotiques, mais bien dans les tissus de l’hôte, précisément dans les mitochondries et dans ces organites particuliers qui ont été dénommés sulphide-oxidising bodies (SOBs), en français « corps oxydant les sulfures » (COS), termes que nous proposons d’adopter pour désigner les organites de même structure rencontrés dans l’épithélium branchial de R. exoculata. Récemment, des structures semblables ont été décrites dans les branchies de vers polychètes alvinellidés (A. pompejana et P. grasslei) ainsi que dans l’intestin et le tégument de l’échiurien, Urechis caupo. Chez U. caupo, les produits terminaux de l’oxydation des sulfures s’accumuleraient dans les SOBs, qui sont considérés comme des lysosomes particuliers assurant la lyse des mitochondries (endommagées ?) après oxydation des sulfures.
Les résultats obtenus chez R. exoculata sont les premiers à fournir des indices structuraux suggérant un rôle des branchies dans la détoxication des sulfures chez un crustacé, alors que l’épithélium branchial des crustacés décapodes est essentiellement connu pour sa participation dans la respiration et l’osmorégulation. C’est également la première fois que sont décrites chez un crustacé des structures intracellulaires probablement impliquées dans la détoxication des sulfures. Chez R. exoculata, comme chez S. reidi et A. pompejana, la présence de COS associés à des mitochondries modifiées suggère que l’oxydation des sulfures est couplée à une production d’ATP. L’existence d’un tel mécanisme a été démontrée chez S. reidi, l’oxydation des sulfures représentant dès lors un apport énergétique direct pour l’organisme.
D’un point de vue écophysiologique, R. exoculata rejoint les espèces hydrothermales, en particulier les Alvinellidés (« vers de Pompéi »), qui vivent sur des cheminées actives. Comme les vers alvinellidés, les crevettes R. exoculata sont supposées dépendre trophiquement des bactéries chimioautotrophes qu’elles hébergent et paraissent détoxiquer les sulfures au niveau de leurs branchies. Les résultats obtenus chez R. exoculata n’excluent toutefois pas l’intervention des branchies dans d’autres fonctions physiologiques, ni l’existence d’autres mécanismes de détoxication des sulfures, plusieurs processus pouvant coexister dans un même organisme.