Version française abrégée
Dans les gı̂tes à moustiques subalpins, les litières arborescentes présentent une toxicité différentielle sur les larves détritivores, grâce à des complexes lignine–polypeptide formés au cours de la décomposition foliaire. Ce nouveau type de larvicide pourrait être utilisé dans une nouvelle stratégie de démoustication visant à remédier aux nombreux problèmes engendrés par l'usage généralisé des insecticides conventionnels. L'intérêt de cette stratégie est évalué par rapport aux stratégies conventionnelles, en comparant les propriétés larvicides de la litière décomposée d'aulne brute à celles du téméphos (insecticide organophosphoré) et de Bacillus thuringiensis var. israelensis (Bti) (bactério-insecticide) sur trois modèles différents de larves de moustiques détritivores (Aedes albopictus Skuse, Culex pipiens L. et Anopheles stephensi Liston).
Une comparaison par bio-essais effectués en conditions standard montre une forte toxicité du téméphos par rapport au Bti et à la litière décomposée, et une tolérance particulière de chaque modèle larvaire en fonction du type de larvicide. Une comparaison par bio-essais effectués dans différentes conditions de dose de larvicide (concentration) et de géométrie de la colonne d'eau (surface, volume, hauteur) met en évidence des effets différents de ces paramètres sur la mortalité, selon le type de larvicide et/ou de larve. Pour la litière décomposée, on observe un effet de surface significatif pour Ae. albopictus et Cx. pipiens, ainsi qu'un effet de volume significatif pour An. stephensi. Pour le Bti, on observe un effet de volume significatif pour An. stephensi et Cx. pipiens. Pour le téméphos, on observe des effets de surface et de volume significatifs, opposant An. stephensi à Cx. pipiens et Ae. albopictus.
Ces différences entre performances toxiques, selon le type de bio-essai, de larvicide et de modèle larvaire, montrent la complexité des interactions entre les différents facteurs intervenant dans la biodisponibilité du xénobiotique par rapport à sa cible. Au niveau du xénobiotique, le comportement physique du larvicide dans l'eau interfère avec la géométrie du milieu : on distingue les effets du téméphos, soluble dans l'eau, de ceux du Bti et de la litière, formant des suspensions plus ou moins stables dans l'eau. Au niveau de la cible, le positionnement dans la colonne d'eau et le comportement alimentaire de chaque modèle larvaire interfèrent avec la répartition du larvicide dans le milieu : on oppose An. stephensi, filtreur–collecteur extensif de surface, à Cx. pipiens, filtreur–collecteur intensif de pleine eau, et à Ae. albopictus, brouteur–collecteur intensif de fond. La complexité de ces interactions, révélée dans notre système expérimental simplifié, doit s'accentuer sur le terrain.
L'ensemble des résultats de notre comparaison montre l'intérêt potentiel d'une stratégie « litière décomposée » en démoustication, notamment dans la gestion durable d'espèces anthrophiles vectrices potentielles (Culex, Aedes). Aux arguments toxicologiques s'ajoutent des arguments environnementaux, biologiques et opérationnels, la litière décomposée contenant naturellement à la fois la substance active et l'adjuvant, nécessaires à toute formulation insecticide. Au-delà de son intérêt appliqué, notre étude révèle la complexité des facteurs intervenant dans l'écologie nutritionnelle et toxicologique des larves de moustiques en milieu aquatique.
1 Introduction
The extensive and widespread use of synthetic insecticides has caused some concerns regarding the toxicological, economical and environmental impact of those xenobiotics [1]. Because of these concerns, some search for new insecticide strategies is now being conducted, particularly on natural plant products, in order to develop phytochemicals possessing efficient, environmentally safe and target-specific pesticidal activity [2,3].
Among these phytochemicals, polyphenols are known for their dietary toxicity to phytophagous insects [4] and detritivorous larval culicine taxa [5]. Particular involvement of polyphenolic complexes in plant-larval mosquito dietary interactions was recently evidenced in the subalpine mosquito breeding sites [6]. These insoluble complexes, included within the cell-wall fraction of crude ten-month-decomposed arborescent leaf litter, exert a dietary toxicity against some detritivorous larval culicine taxa [7,8]. Once ingested, these xenobiotics wreck the larval midgut epithelium [9] following a lysis pattern similar to that observed after intoxication with the bacterio-insecticide Bacillus thuringiensis ssp. israelensis (Bti) [10]. Preliminary phytochemical characterizations have revealed that these larvicidal molecules are related to lignin–polypeptidic complexes [11–13]. Comparative toxicological and behavioural investigations have suggested that the dietary use of decomposed arborescent leaf litter may constitute a new larvicidal strategy usable against anthropophilic vector-competent invasive species resistant to conventional insecticides [14,15].
The efficacy of any mosquito larvicide may, however, depend upon its bioavailability for target larvae in the environmental water together with the own tolerance level of each larval taxon. Several environmental parameters may influence the performances of a given larvicide [16,17]. Contrary to a water-soluble larvicide like temephos, which is used under homogeneous solutions without any dietary interest [18], decomposed leaf litter and Bti are used under heterogeneous suspensions with a dietary interest [15,19]. The physical heterogeneity of these dietary larvicicides may interfere with their toxicity, because of possible modifications in their bioavailability to target larvae in the water column. These modifications may occur according to the dose of toxic material and the geometric characteristics of the water column, which interfere with the own larval feeding behaviour.
This is why, in this paper, we examined the larvicidal performances of toxic leaf litter in comparison with those of temephos and Bti in different conditions of xenobiotic dose and geometry of the environmental water column, liable to simulate field conditions. Such a comparative investigation was performed against different taxa representative of the main detritivorous mosquito larval feeding behaviours [20]. Our results will help us to predict the real efficacy of leaf litter versus conventional larvicides in different field conditions. Moreover, nutritional ecology of mosquito larvae is poorly understood, because resulting from the complex interaction between numerous abiotic and biotic parameters [21]. The different performances of the xenobiotics used in this comparative study under various environmental conditions may serve as indicators liable to evidence the different parameters involved in xenobiotic bioavailability for mosquito larvae in the aquatic medium.
2 Materials and methods
2.1 Animals
Aedes albopictus Skuse, Culex pipiens L., and Anopheles stephensi Liston are widespread anthropophilic vector-competent mosquitoes, whose larvae are detritivorous non-selective feeders. Ae. albopictus is collecting-gathering in loose deposits on the substratum, while Cx. pipiens and An. stephensi are collecting–filtering particulate food in suspension respectively in the main body and at the surface of the water column [20]. In order to obtain reproducible results, experimentation was performed using calibrated third instar larvae from laboratory strains (Ae. albopictus: Italian strain [22]; Cx. pipiens: S-LAB strain [23]; An. stephensi: ST15 strain from ‘Institut Pasteur’, Paris).
2.2 Larvicidal materials
Temephos (Abate 500 E) was used in aqueous solutions. Bti (Bactimos WP, 6000 AAU mg−1, and natural crude ten-month decomposed alder leaf litter were used in aqueous suspensions. Bti particle size did not exceed 50 μm [24]. Leaf litter (average particle size: 0.4 mm) was sampled and prepared following David et al. [9]. All larvicidal media were made with pure tap water adjusted to pH 7.5 according to Rey et al. [15].
2.3 Standard bioassays
These three larvicidal materials were toxicologically evaluated against the three larval types using the standard bioassay technique [25] performed in single-use vials containing 50 ml aqueous media. Temephos was used at concentrations varying from 0.0004 to 0.02 mg l−1. Bti was used at concentrations varying from 0.05 to 50 mg l−1. Leaf litter was used at concentrations varying from 20 to 7000 mg fresh mass per litre. Each bioassay was conducted in triplicate at 25 °C, on 20 starved larvae, during 24 h. Controls were reared in pure tap water with pH adjusted as above.
2.4 Modified bioassays
Different parameters susceptible to interfere with the larval mortality were examined for each toxic material throughout different bioassay designs. Those parameters were: dose (expressed as concentration) of toxic material (four concentrations assayed: C1<C2<C3<C4, see Tables 1, 2); volume of the water column (two volumes assayed: V1=50 ml<V2=200 ml); surface of the water column (two surfaces assayed: S1=10 cm2<S2=60 cm2); height of the water column (four heights assayed: H1=1 cm <H2=3.5 cm<H3=5 cm<H4=20 cm). One parameter varying for one bioassay to another, eight different bioassay designs for each toxic material were selected (Table 1). Because of the differential toxicity levels of the bioassayed materials, mortality ranges suitable for a comparable dose-mortality correlation among the three larval types were preliminary adjusted according to the dose of toxic material and the time of exposition. This allowed us to adopt the dose and time parameters indicated in Table 2.
Different experimental parameters selected in the modified bioassays
Bioassay number | Experimental parameters | |||
Geometry of the water column | Dose of toxic material | |||
Surface | Volume | Height | Concentration | |
1 | S 2 | V 1 | H 1 | C 3 |
2 | S 2 | V 1 | H 1 | C 4 |
3 | S 2 | V 2 | H 2 | C 1 |
4 | S 2 | V 2 | H 2 | C 2 |
5 | S 1 | V 1 | H 3 | C 3 |
6 | S 1 | V 1 | H 3 | C 4 |
7 | S 1 | V 2 | H 4 | C 1 |
8 | S 1 | V 2 | H 4 | C 2 |
Doses (expressed as concentrations) of toxic material and times of exposition used in the modified bioassays
Type of toxic material | Dose of toxic material (mg l−1) | Time of exposition (h) | Larval types | |||||||||
C 1 | C 2 | C 3 | C 4 | T 1 | T 2 | T 3 | T 4 | T 5 | T 6 | T 7 | ||
2 | 4 | Ae. albopictus | ||||||||||
Leaf litter | 25 | 50 | 100 | 200 | 5 | 6 | Cx. pipiens | |||||
2 | 4 | An. stephensi | ||||||||||
17 | 22 | 24 | Ae. albopictus | |||||||||
Bti | 0.0375 | 0.0750 | 0.150 | 0.300 | 17 | 22 | 24 | Cx. pipiens | ||||
17 | 22 | 24 | An. stephensi | |||||||||
17 | 22 | 24 | Ae. albopictus | |||||||||
Temephos | 0.0009 | 0.0018 | 0.0036 | 0.0072 | 2 | 4 | 5 | Cx. pipiens | ||||
17 | 22 | 24 | An. stephensi |
2.5 Data analysis
After correction with Abbott's formula [26], mortality data were analysed with the log-probit program of Raymond [27]. Efficacy of the different parameter combinations on the mortality rate of each larval type for each toxic material were assessed by a repeated measure analysis of variance using GLM procedure [28]. In this study, between-subject effects were: dose (expressed as concentration: C), surface (S), and volume (V). Within-subject effects were the above effects with the additional effects of time (T).
3 Results
3.1 Differential larvicidal effects of leaf litter, Bti and temephos in standard conditions
Standard bioassays allowed us to evidence a differential tolerance of Ae. albopictus, Cx. pipiens and An. stephensi against leaf litter, Bti and temephos, according to both the toxic material and the larval type (Table 3). Leaf litter was less toxic than conventional insecticides, and temephos was more toxic than Bti. When using leaf litter, Cx. pipiens was the most sensitive, followed by An. stephensi and Ae. albopictus. When using conventional insecticides, An. stephensi, relatively less sensitive to Bti and temephos, may be opposed to Ae. albopictus and Cx. pipiens, relatively more sensitive.
Comparative larval tolerance to leaf litter, Bti and temephos among the three larval types, obtained with standard bioassays. Mortality rates are expressed as mean 24-h median lethal concentration (LC50) values
24 h median lethal concentration (mg/l) | |||
LC 50 | 95% confidence limits of LC50 | Slope±SE | |
Leaf litter | |||
Ae. albopictus | 35.31 | 28.75–42.82 | 2.67±0.34 |
An. stephensi | 22.36 | 11.1–33.54 | 1.35±0.21 |
Cx. pipiens | 11.98 | 1.35–25.78 | 1.24±0.32 |
Bti | |||
An. stephensi | 0.18 | 0.14–0.23 | 2.80±0.68 |
Cx. pipiens | 0.12 | 0.11–0.14 | 7.60±2.55 |
Ae. albopictus | 0.04 | 0.02–0.08 | 2.89±0.95 |
Temephos | |||
An. stephensi | 0.15 | NC | 1.31±0.72 |
Cx. pipiens | 0.07 | NC | 0.64±0.39 |
Ae. albopictus | 0.03 | 0.006–0.14 | 0.94±0.58 |
3.2 Influence of dose of toxic material, time of exposition and environmental geometrical parameters on the larvicidal effects of leaf litter, Bti, and temephos
Mortality data obtained throughout the 8 modified bioassay designs showed that, whereas dose and time effects were always significant, the effects of the other parameters varied according to the toxic material and the larval type (Table 4, Figs. 1–3).
Analysis of variance for the effects on the larval mortality of dose of larvicidal material (expressed as concentration), environmental geometrical parameters (surface, volume) and time, using toxic leaf litter (a), Bti (b), and temephos (c). Significant results at P<0.05 are in bold
Source | Aedes albopictus | Anopheles stephensi | Culex pipiens | ||||||
dF | F value | P>F | dF | F value | P>F | dF | F value | P>F | |
(a) Leaf litter | |||||||||
Dose (C) | 1 | 15.66 | 0.0010 | 1 | 15.35 | 0.0018 | 1 | 20.87 | 0.0004 |
Surface (S) | 1 | 89.67 | <0.0001 | 1 | 0.87 | 0.3691 | 1 | 44.35 | <0.0001 |
Volume (V) | 1 | 0.45 | 0.5101 | 1 | 30.60 | <0.0001 | 1 | 0.09 | 0.7689 |
C×S | 1 | 2.62 | 0.1242 | 1 | 4.92 | 0.0449 | 1 | 33.11 | <0.0001 |
C×V | 1 | 4.97 | 0.0396 | 1 | 1.59 | 0.2299 | 1 | 0.69 | 0.4211 |
S×V | 1 | 13.77 | 0.0017 | 1 | 4.14 | 0.0629 | 1 | 0.02 | 0.8902 |
C×S×V | 1 | 23.62 | 0.0001 | 1 | 2.43 | 0.1433 | 0 | ||
Error | 17 | 13 | 14 | ||||||
Time (T) | 1 | 200.37 | <0.0001 | 1 | 178.24 | <0.0001 | 1 | 42.11 | <0.0001 |
T×C | 1 | 0.81 | 0.3801 | 1 | 0.04 | 0.8499 | 1 | 6.35 | 0.0245 |
T×S | 1 | 0.09 | 0.7636 | 1 | 0.26 | 0.6205 | 1 | 16.81 | 0.0011 |
T×V | 1 | 7.85 | 0.0123 | 1 | 1.31 | 0.2732 | 1 | 0.17 | 0.6883 |
T×C×S | 1 | 13.37 | 0.0020 | 1 | 3.82 | 0.0725 | 1 | 14.45 | 0.0019 |
T×C×V | 1 | 13.45 | 0.0019 | 1 | 3.93 | 0.0690 | 1 | 0.00 | 0.9635 |
T×S×V | 1 | 0.79 | 0.3858 | 1 | 0.24 | 0.6321 | 1 | 0.48 | 0.4984 |
T×C×S×V | 1 | 6.08 | 0.0246 | 1 | 0.13 | 0.7231 | 0 | ||
Error | 17 | 13 | 14 | ||||||
(b) Bti | |||||||||
Dose (C) | 1 | 14.54 | 0.0015 | 1 | 49.99 | <0.0001 | 1 | 19.33 | 0.0005 |
Surface (S) | 1 | 3.50 | 0.0796 | 1 | 0.58 | 0.4564 | 1 | 0.13 | 0.7186 |
Volume (V) | 1 | 0.01 | 0.9172 | 1 | 12.46 | 0.0030 | 1 | 115.80 | <0.0001 |
C×S | 1 | 4.02 | 0.0622 | 1 | 0.60 | 0.4519 | 1 | 0.73 | 0.4045 |
C×V | 1 | 0.12 | 0.7361 | 1 | 0.41 | 0.5329 | 1 | 2.83 | 0.1117 |
S×V | 1 | 0.16 | 0.6955 | 1 | 9.35 | 0.0080 | 1 | 0.45 | 0.5115 |
C×S×V | 1 | 0.15 | 0.7026 | 1 | 0.02 | 0.8991 | 1 | 0.76 | 0.3600 |
Error | 16 | 15 | 16 | ||||||
Time (T) | 2 | 4.82 | 0.0148 | 2 | 11.72 | 0.0002 | 2 | 85.18 | <0.0001 |
T×C | 2 | 3.08 | 0.0597 | 2 | 4.20 | 0.2460 | 2 | 5.21 | 0.0110 |
T×S | 2 | 0.90 | 0.4171 | 2 | 1.51 | 0.2374 | 2 | 0.94 | 0.4000 |
T×V | 2 | 0.67 | 0.5207 | 2 | 0.69 | 0.5088 | 2 | 23.34 | <0.0001 |
T×C×S | 2 | 1.18 | 0.3214 | 2 | 2.72 | 0.0821 | 2 | 2.99 | 0.0644 |
T×C×V | 2 | 0.45 | 0.6414 | 2 | 1.39 | 0.2657 | 2 | 1.72 | 0.1957 |
T×S×V | 2 | 1.20 | 0.3146 | 2 | 1.84 | 0.1767 | 2 | 0.27 | 0.7678 |
T×C×S×V | 2 | 2.31 | 0.1155 | 2 | 0.64 | 0.5332 | 2 | 1.53 | 0.2316 |
Error | 32 | 30 | 32 | ||||||
(c) Temephos | |||||||||
Dose (C) | 3 | 85.94 | <0.0001 | 3 | 14.69 | <0.0001 | 3 | 9.17 | 0.0005 |
Surface (S) | 1 | 26.14 | <0.0001 | 1 | 12.16 | 0.0033 | 1 | 18.62 | 0.0005 |
Volume (V) | 1 | 192.81 | <0.0001 | 1 | 21.41 | 0.0003 | 1 | 42.09 | <0.0001 |
S×V | 1 | 0.10 | 0.7590 | 1 | 0.04 | 0.8533 | 1 | 0.51 | 0.4853 |
C×S×V | 4 | 74.18 | <0.0001 | 4 | 16.12 | <0.0001 | 4 | 2.06 | 0.1345 |
Error | 23 | 15 | 16 | ||||||
Time (T) | 2 | 10.74 | 0.0001 | 2 | 22.59 | <0.0001 | 2 | 15.70 | <0.0001 |
T×C | 6 | 2.05 | 0.0748 | 6 | 2.47 | 0.0409 | |||
T×S | 2 | 2198.00 | <0.0001 | 2 | 0.20 | 0.8211 | 2 | 0.07 | 0.9314 |
T×V | 2 | 10.45 | 0.0002 | 2 | 1.49 | 0.2410 | 2 | 8.04 | 0.0015 |
T×S×V | 2 | 14.60 | <0.0001 | 2 | 2.11 | 0.1393 | 2 | 8.48 | 0.0011 |
T×C×S×V | 8 | 2.93 | 0.0099 | 8 | 1.99 | 0.0826 | 8 | 1.62 | 0.1584 |
Error | 46 | 30 | 32 |
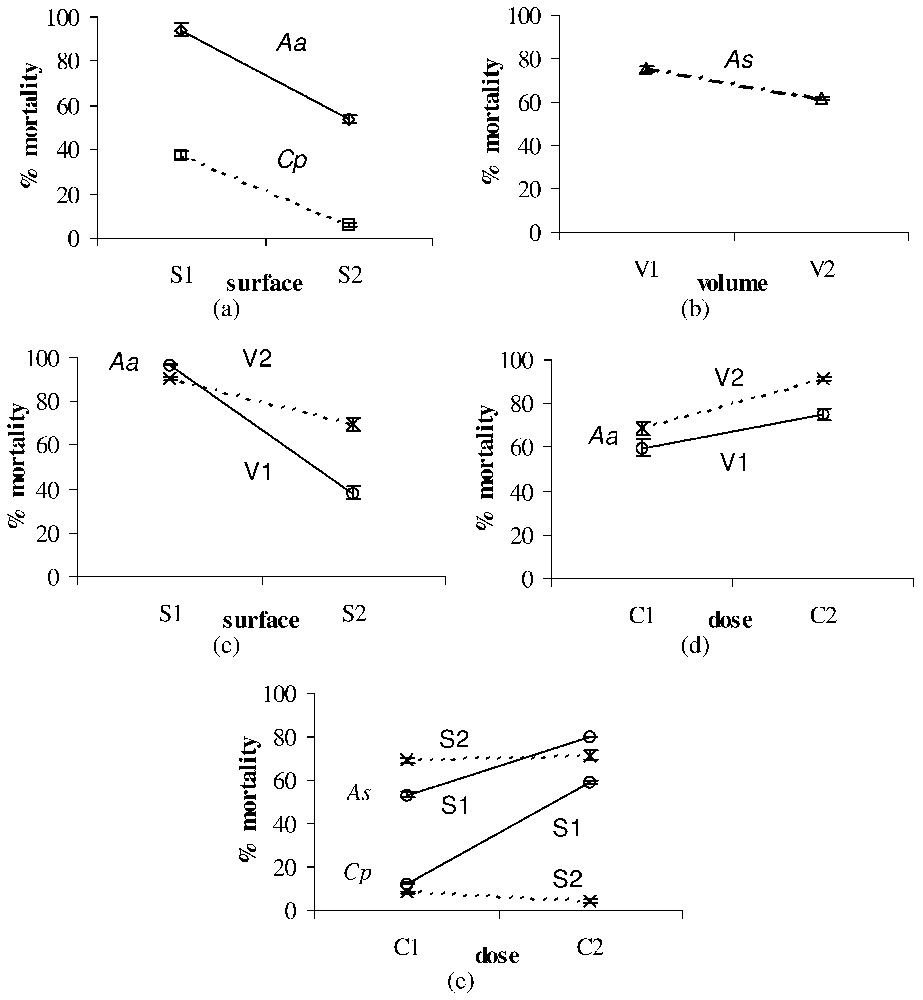
Significant surface, volume and dose effects on the larval mortality (%±SE) after treatment with leaf litter of Ae. albopictus (Aa), Cx. pipiens (Cp), and An. stephensi (As). All effects were observed for each larval type at the second time of exposition used for each toxic material (see Table 2). (a) Surface effect after treatment with leaf litter of Aa (observed at T2) and Cp (observed at T4). (b) Volume effect after treatment with leaf litter of As (observed at T2). (c) Surface × volume interaction after treatment with leaf litter of Aa (observed at T2). (d) Dose × volume interaction after treatment with leaf litter of Aa (observed at T2). (e) Dose × surface interaction after treatment with leaf litter of As (observed at T2) and Cp (observed at T4).
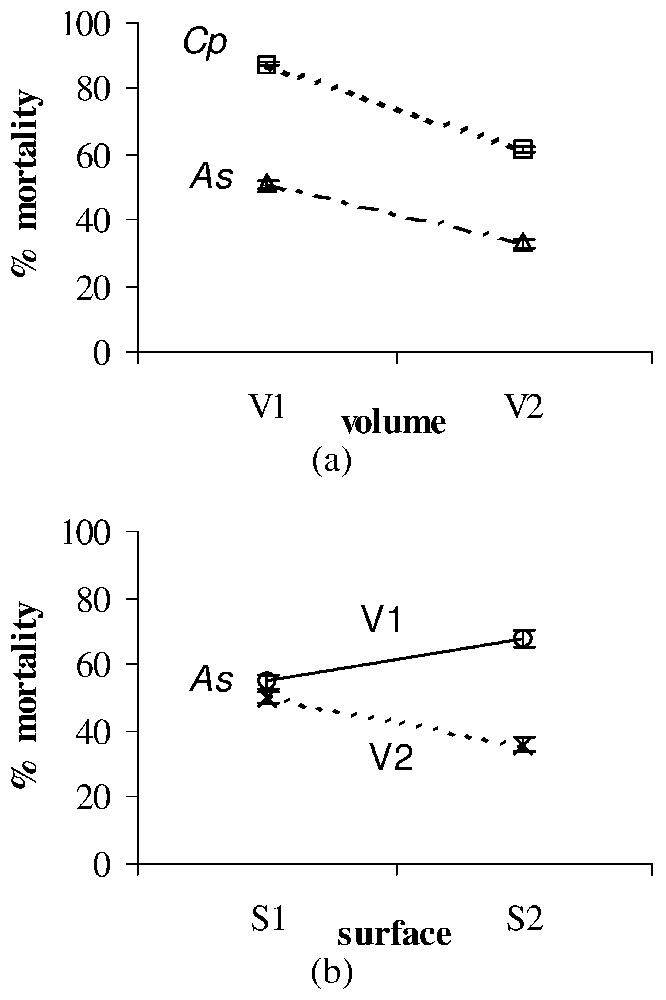
Significant surface, volume and dose effects on the larval mortality (%±SE) after treatment with Bti of Ae. albopictus (Aa), Cx. pipiens (Cp), and An. stephensi (As). All effects were observed for each larval type at the second time of exposition used for each toxic material (see Table 2). (a) Volume effect after treatment with Bti of As (observed at T6) and Cp (observed at T6). (b) Surface × volume interaction after treatment with Bti of As (observed at T6).
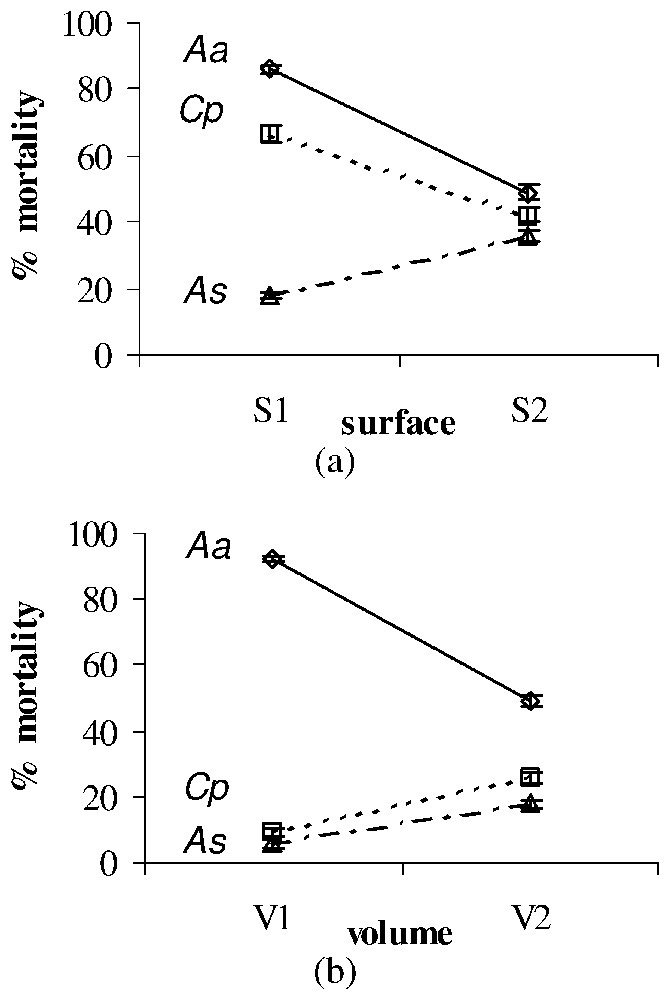
Significant surface, volume and dose effects on the larval mortality (%±SE) after treatment with temephos of Ae. albopictus (Aa), Cx. pipiens (Cp), and An. stephensi (As). All effects were observed for each larval type at the second time of exposition used for each toxic material (see Table 2). (a) Surface effect after treatment with temephos of Aa (observed at T6) and Cp (observed at T2) versus As (observed at T6). (b) Volume effect after treatment with temephos of Aa (observed at T6) versus Cp (observed at T2) and As (observed at T6).
Leaf-litter larvicidal effect appeared to be significantly influenced by the water surface for Ae. albopictus and Cx. pipiens, the mortality rates decreasing with the surface of the water column (Fig. 1a). On the other hand, the volume effect was significant for An. stephensi, the mortality rates decreasing with the volume (i.e., height) of the water column (Fig. 1b). Significant surface × volume and dose × volume interactions were found for Ae. albopictus (Figs. 1b and 1c). Conversely, a significant concentration × surface interaction was found for An. stephensi and Cx. pipiens (Fig. 1e).
Bti larvicidal effect appeared to be significantly influenced by the environmental volume only for An. stephensi and Cx. pipiens, the mortality rates decreasing with the volume (Fig. 2a). A significant surface × volume interaction was also observed for An. stephensi (Fig. 2b).
Temephos larvicidal effect appeared to be significantly influenced by the environmental surface and volume, whatever the dose and the larval type, with opposite effects on An. stephensi versus Ae. albopictus and Cx. pipiens (Fig. 3a). On the contrary, the volume (or height) effect allowed us to oppose Cx. pipiens and An. stephensi versus Ae. albopictus (Fig. 3b).
4 Discussion
Results from our different bioassay designs reveal that numerous intrinsic and extrinsic factors may influence the toxicological performances of a given insecticide against a given target mosquito larva. All these factors are to be taken into account for examining the real interest of any larvicidal strategy.
4.1 Comparative bioavailability characteristics of leaf litter, Bti and temephos for mosquito larvae
The bioavailability for target larvae of a xenobiotic in the ambient water is primarily influenced by its physicochemical performances in the medium [29]. This allows us to distinguish water-soluble materials (e.g., temephos), which tend to be homogeneously distributed throughout the whole medium whatever its geometry, from water-insoluble Bti and leaf litter, distributed under more or less stable suspensions. Because of the thinner size of their particles, Bti suspensions are more stable in the water column than leaf litter suspensions [15], which sink according to the basal surface geometry of the water column. Such a variable basal distribution of leaf litter particles may greatly influence their bioavailability for Ae. albopictus and Cx. pipiens, living much deeply in the water column, rather than for An. stephensi, living at the top of the water column [20,30]. In the case of Ae. albopictus and Cx. pipiens, our modified bioassay results reflect those bioavailability differences between Bti and leaf litter according to the environmental surface or volume (i.e., depth) of the water column.
The relative larvicidal performances of leaf litter, Bti and temephos are also influenced by the larval behaviour. This appears to be the case for temephos, where differential surface and volume (i.e., depth) effects oppose An. stephensi, living at the water surface, from Cx. pipiens, and Ae. albopictus, living much deeply in the water column. Such an interaction may be of key importance for Bti and leaf litter, as the differential larval feeding behaviours appear to greatly interfere with the differential distribution of those dietary larvicidal materials throughout the water column. This is clearly suggested by the surface and volume (i.e., depth) effects discriminating An. stephensi, feeding at the water surface, from Cx. pipiens and Ae. albopictus, feeding in much deeper water or at the bottom [31,32].
Moreover, the differences in larval feeding rates may be crucial in the differential larval tolerance against toxic dietary xenobiotics [33]. This has been observed for Anophelinae, which are less susceptible to Bti than other mosquito taxa, because of their low feeding rates [34]. This is also true for leaf litter, where the most sensitive taxa (i.e., Aedinae) are those that can ingest the maximum amount of toxic material because of their active collecting–gathering feeding mode [35].
4.2 Comparative efficacy of leaf litter and conventional insecticide strategies in larval mosquito control
The results of our modified bioassays suggest that the mortality rates obtained through standard bioassays are to be modulated by the environmental conditions in conjunction with the own larval behaviour. The influence of those abiotic and biotic factors may thus be taken into account to understand the real efficacy of a given larvicide against a given larval type.
Our current results confirm that chemical insecticides (e.g., temephos) are indistinctively effective against widespread anthropophilic larval Aedes, Culex and Anopheles taxa. These wide toxicological performances, however, are modulated at the operational level by environmental and human health concerns [36,37] together with emergence of resistance phenomena in the target fauna [38]. In the same way, the larvicidal use of Bti in human dominated breeding sites is checked by its differential efficacy against anthropophilic culicine taxa [19], the presence of environmental factors affecting its bioavailability to target larvae [39], and its ephemeral time of action [40].
Although the standard bioassays indicate larvicidal performances for toxic leaf litter lower than for conventional larvicides, the modified bioassays reveal the bioavailability performances of this phyto-larvicide, which, in return for a sufficient dose in the medium (e.g., 200 mg l−1), displays acute toxicity effects more rapidly than conventional larvicides [15]. Moreover, a possible use of this vegetable larvicide may be recommended in human dominated mosquito breeding sites because of its environmental interest. Interestingly, crude leaf litter material represents per se a whole insecticide formulation, i.e., including both the active ingredient and the adjuvant. Whereas the active ingredient is not yet precisely characterized [11,13], a preliminary purification procedure revealed that its toxic efficacy would be 100-fold higher than that of crude leaf litter [12]. The adjuvant corresponds to the non-toxic components of the cell-wall fraction remaining after the ten-month decaying process of the foliar material [7]. This cell-wall material is both stable in the aquatic medium [11] and appetent for the larvae [14]. Such biological performances, associated to the sinking ability of crude leaf litter suspensions in the water column, predispose this material to control preferentially Aedes taxa, which feed on the substratum [20]. Innovative control strategies are there needed urgently because of the progressive inefficacy of conventional insecticides which have been over-used against those invasive taxa [41] known for their wide vector competence [42].
5 Conclusion
As shown by the current experimental study, the bioavailability of xenobiotics for mosquito larvae in the aquatic medium appears to result from the complex interaction among several factors: dose and physicochemical characteristics of the xenobiotic, time of exposition, geometry of the water column, and larval behaviour. As those xenobiotics may be of dietary and/or toxicological interest, knowledge of such interactions may allow us to better understand the nutritional and/or toxicological ecology of mosquito larvae, in order to improve control strategies.
Acknowledgements
This investigation was supported by a grant from the ‘Entente interdépartementale pour la démoustication Ain–Isère-Rhône–Savoie’. We thank I. Thiery and A. Failloux for supplying A. aegypti strain, R. Bellini for supplying A. albopictus strain, C. Nielsen-Leroux for supplying A. stephensi strain, J. Patouraux for technical assistance, and I. Till-Bottraud for help in statistics.