Abbreviations
Muscle aponeuroses
Ace | aponeurosis caudalis |
Acg | ap. ceratoglossis |
Ado | ap. dorsalis originalis |
Ad | ap. depressoris |
Adi | ap. dorsalis insertionis |
Ali | ap. lateralis insertionis |
Alm | ap. lateralis mandibularis |
Alo | ap. lateralis originalis |
Alt | ap. lateralis temporalis |
Am | ap. medialis |
Amg | ap. superficialis magna |
Amq | ap. medioquadrata |
Amr | ap. mediorostralis |
Ams | ap. mediosuperficialis |
Ao | ap. occipitalis |
Ap | ap. posterior |
Aps | ap. pseudotemporalis superficialis |
Ar | ap. rostralis |
As | ap. superficialis |
Avi | ap. ventralis insertionis |
Avo | ap. ventralis originalis |
Other abbreviations
aem | m. add. md. ext. medialis |
aer | m. add. md. ext. profundus rostralis |
aes | m. add. md. ext. superficialis |
ap | m. add. md. posterior |
bas | basihyale |
bm | m. branchiomandibularis |
cb | ceratobranchiale |
cgp | m. ceratoglossus anterior |
ch | m. ceratohyoideus |
ci | medial condyle |
dm | m. depressor mandibulae |
eb | epibranchiale |
ent | entoglossum |
fm | foramen magnum |
gg | m. genioglossus |
hg | m. hypoglossus |
jg | jugale (arcus jugalis) |
lje | ligamentum jugomandibulare externum |
lji | lig. jugomand. internum |
lom | lig. occipitomandibulare |
lp | lig. postorbitale |
md | mandibula |
mst | mesethmoideum |
mh | m. mylohyoideus |
ns | nasale |
par | paraglossalia |
pcm | Proc. caudalis mandibulae |
pdl | m. pt. dorsalis lateralis |
pdm | m. pt. dorsalis medialis |
pcm | Proc. caudalis mandibulae |
pim | Proc. internus mandibulae |
pl | palatinum |
plm | Proc. lateralis mandibulae |
po | Proc. orbitalis (ossis) quadrati |
pp | Proc. postorbitalis |
ppm | Proc. palatinus (ossis) maxillaris |
pr | m. protractor pterygoidei et quadrati |
psp | m. pseudotemporalis profundus |
pss | m. pseudotemporalis superficialis |
pss(l) | m. pseudotemp. superfic. lateralis |
pss(m) | m. pseudotemp. superfic. medialis |
Pt | m. pterygoideus |
pt | (os) pterygoideum |
pvl | m. pt. ventralis lateralis |
pvm | m. pt. ventralis medialis |
pz | Proc. zygomaticus |
q | Quadratum |
sh | m. serpihyoideus |
ss | os sesamoid |
sth | m. stylohyoideus |
tp | tensor periorbitae |
zpr | prokinetic hinge |
Version française abrégée
Oiseaux tropicaux, omnivores consommant des aliments aussi variés que des fruits de différents types (mous, arillés, à grosses graines...), des arthropodes de tailles diverses et même des petits vertébrés, et bien que d'observation relativement aisée, les motmots (Momotidés, Coraciiformes) n'ont guère fait l'objet d'études liant leurs particularités morpho-anatomiques à des traits biologiques, comportementaux ou écologiques. Ainsi, une question aussi simple que la signification fonctionnelle des crénelures que présentent les tomies de leur bec demeurait sans réponse. Dans le présent article, nous décrivons la structure des appareils du bec et hyoı̈dien du Motmot houtouc (Momotus momota) et, en appliquant la méthode graphique de Dzerzhinsky d'analyse statique des conditions d'équilibre des forces au cours de la manipulation d'objets, nous cherchons à identifier les fonctions qui peuvent être interprétées comme des adaptations trophiques et qui peuvent générer des hypothèses testables par observation directe d'animaux vivants.
Le squelette crânien est décrit en insistant sur les particularités des motmots par rapport aux autres Néognathes : par exemple, rhamphothèque crénelée, arête du culmen dans le prolongement du front, charnière procinétique rectiligne et pourvue d'une très fragile plaque osseuse, mur postérieur franc de la mâchoire supérieure, lacrymal absent, long processus postorbitaire, palatins étroits, carré court par rapport à la hauteur de la mâchoire supérieure à sa base, articulation mandibulaire avec trois condyles.
De même, dans les ligaments, l'attention est attirée sur le postorbitaire très développé qui s'insère le long du bord postérieur du processus postorbitaire. Le jugomandibulaire externe est presque vertical ; la partie antérieure de la grande aponévrose superficielle est repliée médialement et s'étend le long de la face postérieure du processus maxillaire renflé du nasal, arrivant presque à la hauteur de la cloison interorbitaire. Plus caudalement à son attache sur la mâchoire supérieure, cette aponévrose est liée au bord latéral de l'aponévrose périorbitaire.
Dans la description de la musculature crânienne, un accent particulier est mis sur les aponévroses, riches en information fonctionnelle. Les adducteurs sont bien développés, de même (ce qui n'est pas le cas chez les autres Coraciiformes) que les mm. pseudotemporalis superficialis et profundus. Le m. pterygoideus est remarquable par sa tendance à une orientation longitudinale le long de la ligne médiale du palais.
Pour ce qui concerne l'appareil hyoı̈dien, contrairement à divers autres oiseaux, le motmot montre des cornes hyoı̈diennes qui ne contournent pas le dépresseur de la mandibule, mais s'appliquent sur la surface inférieure du muscle ptérygoı̈de.
Au plan morphofonctionnel, l'appareil du bec du motmot se caractérise par un fort cinétisme, aisément mis en évidence par des préparations syndesmologiques et favorisé par la structure particulière de la charnière procinétique, le large espace entre le bord postérieur de la mâchoire et la paroi interorbitaire, et surtout par le fait que, même quand cette mâchoire est en protraction extrême, le carré peut encore se déplacer. Des mécanismes de sécurité renforcent la stabilité du mécanisme du palais, notamment par la présence de sésamoı̈des dans la partie postérieure développée du ligament jugomandibulaire interne.
Il semblerait que, chez les motmots, le lacrymal soit incorporé à la mâchoire supérieure : des études des stades de développement seraient utiles à ce sujet. La forte hauteur basale de cette mâchoire supérieure, jointe à un fort cinétisme du bec, a pour conséquence d'accroı̂tre la mobilité longitudinale des bords crénelés de la rhamphothèque, qui fonctionneraient alors comme un système interactif de scies, permettant aux oiseaux de ramollir et de découper les gros items qui entrent dans la composition de leur régime alimentaire. Par ailleurs, le m. brachiomandibularis avec le m. mylohyoideus et le basihyal font que la langue remonte vers le palais bien plus haut qu'habituellement chez les oiseaux, ce qui autorise le motmot à traiter ses aliments dans tout l'espace libre entre les mâchoires, même quand le bec est grand ouvert.
Le bec du motmot fonctionne selon le premier type de serrage des mâchoires selon la terminologie de Dzerzhynsky, où les adducteurs agissent ensemble sur les deux mâchoires qu'ils déplacent simultanément. Une analyse biomécanique des équilibres des forces exercées durant le traitement des items alimentaires conduit à concevoir que, tant durant la protraction que durant la rétraction des mâchoires, un état d'équilibre indifférent s'installe chez le motmot, permettant de serrer correctement les items et de les faire rouler sans risque le long des mâchoires crénelées. Le ligament postorbitaire, bien que développé, est en fait plus faible que, par exemple, chez les Coraciidés. Il est peu efficace pour transformer la force du dépresseur en une force de protraction de la mâchoire supérieure, mais est en revanche très utile pour mettre la mandibule en adduction.
Bien qu'en mesure de fragmenter des items alimentaires, mais n'étant pas streptognathes, les motmots incluent pourtant régulièrement dans leur régime des éléments de grande taille qu'ils sont capables de traiter en entier. Cette étude morphofonctionnelle a montré qu'ils utilisent pour cela le jeu de mouvements réciproques, comparable à celui d'un système de scies, de leurs mâchoires crénelées qui leur permettent, avec le support de l'appareil hyoı̈dien surélevant les aliments dans la cavité buccale, de traiter ces derniers de manière à les rendre propres à l'ingestion et ainsi de conserver une gamme relativement large de taille des items alimentaires.
1 Introduction
An extensive literature exists (e.g., for America, [1–4]) on the ecology and more generally the biology of tropical rainforest birds. However, studies of their ecomorphology are sorely needed. Even quite easy to observe species such as neotropical motmots remain poorly known on these aspects. For instance, no satisfactory explanation has yet been proposed for peculiar, sometimes striking morphological features of these birds, e.g., the crenate edges of their rhamphotheca.
In the present paper, we examine how data available on food and general behaviour of the blue-crowned motmot (Momotus momota, Momotidae) and results of a morphofunctional analysis of its bill and hyoid apparatus can be contrasted in order to understand the trophic adaptations of this species and see whether some working hypotheses can be set forth and extended to others.
Motmot bill and hyoid structures were previously studied [5]. Comparisons with other Coraciiformes (e.g., [6,7]) and analyses based on Dzerzhinsky's graphical method [8] may disclose peculiar functions that could be interpreted as feeding adaptations and generate hypotheses on foraging and food processing techniques that field observations could confirm or disprove. Such a theoretical approach also helps to define the place of these species within the forest biota, and their evolutionary pathway within the order Coraciiformes.
2 Material and methods
2.1 Specimens examined
Skull (three specimens) and cranial muscles, hyoid skeleton and musculature (one specimen) of Momotus momota were dissected and syndesmological preparations of motmot heads were manipulated to evaluate the possible freedom of movement in the bill apparatus. A special attention was paid to the identification of the various muscular units and to how internal aponeuroses were arrayed. The latter are indeed particularly useful for identifying the complex muscular layers and are functionally informative as they give the direction of the muscular lines of action. We followed the nomenclature of aponeuroses used by Dzerzhinsky and Potapova [9]. Skull of Barypthengus ruficapillus and bills of all Momotidae species present in the collections held at ‘Muséum national d'histoire naturelle’ (Paris) were examined for comparison.
2.2 Motmot diet
Motmots are omnivorous species with varied foraging behaviour and quite diverse prey types. Detailed though scanty information on their diet is mainly found in Skutch [2,10–13], but also in, e.g., [14–22]. Small species would be more carnivorous and less frugivorous than larger ones [2]. M. momota is a relatively large bird that feeds almost equally on animal prey (particularly large arthropods) and fruit. It sallies from a not very high perch to take prey from a trunk or leaf, and may even drop to catch prey on the ground. It may also pick up fruit on the ground (pers. obs.) or even glean soil litter for insects with jerky head movements. It may join mixed-flocks around raiding army ant swarms or come around cattle in search of dung beetles. The insect part of its diet consists mainly in large cicadas, also very long stick and leaf insects (Phasmida), caterpillars, grasshoppers, dragonflies, and less often moths. It catches also frogs, lizards and snakes (pers. obs.) and may bring small birds to nestlings. Before swallowing or bringing it to young, the bird softens or dismembers its prey with forceful smashes on perch or on ground. Many fruit types are eaten, either soft (e.g., figs), or with arilate seeds (e.g., some Zingiberaceae, Myristicaceae), or fleshy large-seeded (e.g., Burseraceae, Lauraceae). Fruit is often plucked with the tip of the bill and rolled along the jaws to be processed in their medial or basal part, being after that swallowed entire or with their seed(s) squeezed out (pers. obs.). The bird may bite off pieces of large soft fruits. Blue-crowned is probably the only motmot able to eat bananas. Skutch [2] noted also that, as they grow, nestlings receive larger and larger quantities of oil-rich fruits and starch-rich arils. He was also surprised by the size of these fruits, which looked wider than gape. Once a 15-cm-long snake was given to a nestling.
2.3 Biomechanical analysis
We used Dzerzhinsky's morphofunctional approach of a graphic analysis of static balance of the forces [8] (see also [23]), which is derived from a classical practice in building engineering based upon fundamental laws of mechanics. Here, contrary to dynamics, statics does not need any knowledge of the absolute value of the forces or even what causes them. The mere objective is to find the conditions of static balance between the forces involved in the system. The graphical solution implies that the system is reduced to three forces arranged head to tail so that their algebraic sum is null. Anatomical analysis gives the lines of action of forces that are applied on actual sites. Forces as every vector can be slid along the lines of action to combine several forces or determine the components of a single force. To begin, an arbitrary vector value is chosen (or effectively measured) for one of the significant forces, then all other vectors and their values are obtained by means of strict rules of theoretical mechanics. The resulting graphical model exemplifies the mechanical properties of a particular apparatus as an integrated system.
When the bill works as a pair of tongs, clamping a food item in the jaws implies a steady equilibrium state of the apparatus, all the more as the item is held towards bill tip. This equilibrium is established when the forces that the upper jaw and the mandible (lower jaw) exert on the item are opposed and equal. When it is not the case, i.e., one jaw applies a stronger force than the other, the item is pushed out of the bill. To illustrate how the conditions of the static equilibrium are determined, let us comment Fig. 5A. Clamping forces are produced by the adductors of the mandible, which act simultaneously on the mandible and on the upper jaw. The elaboration of the graphical model begins by placing the forces exerted by the adductors; the orientation of these forces is indicated by the arrangement of the muscles on the skull. The adductors exert the force on the mandible and the force (equal but opposed to ) on the skull. Force acts on the food item and, through the quadratomandibular joint, on the quadrate. Clamping forces are transmitted to the food item along an axis passing at the two points of contact between the item and the jaws. To determine the clamping force from the mandible, force is slid to j at the junction of the axis of clamping and that along which the adductors work. At j, can be decomposed into two forces: (the mandibular clamping force) and , which works towards the quadrate along the axis passing at point q (quadratomandibular joint). The action of this latter force is understood when it is slid to q, where it can be decomposed into two forces: , along the quadrate axis q–x and which is the pressure on the neurocranium via the quadrate, and , which retracts the upper jaw through the jugal bar q–c (for simplification we use here the term jugal bar to designate the complex jugal bar, pterygoid, palatine and m. pterygoideus, which all transmit forces in the same direction and in almost the same plane). This latter force produces both the clamping force from the upper jaw and a pressure that the upper jaw exerts on the neurocranium through the kinetic hinge b. These two forces can be identified when is slid to point a, junction of the clamping axis and that along which works. At point a, can be split into the upper jaw clamping force , which works along the clamping axis passing through the food item, and the force pressing the neurocranium along the axis passing through the kinetic hinge a. The skull is thus submitted to three forces: and , which are the components of force (action of the adductors on the mandible), and (action of the adductors on the skull). There is a steady equilibrium state when these three forces are applied together at point z; in that case, as ( and are equal but opposed), the algebraic sum of and is null. As shown in Fig. 5A, point z in the equilibrium condition is always at the junction of the axes of action of the adductors, of the quadrate and of the upper jaw pressure on the neurocranium.
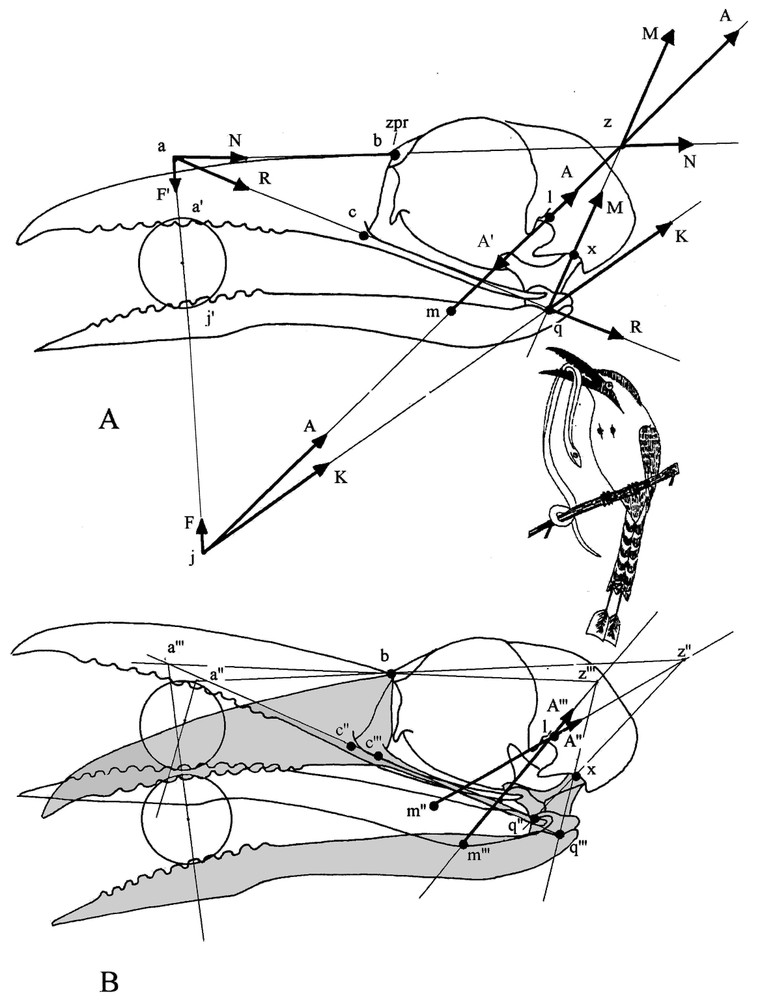
(A) Graphic of the equilibrium state of forces when a food item is squeezed in the middle of the bill. Forces are quoted sequentially in the order of the graphical construction (see methods). (1) , force vector of the mandible adductors (with an arbitrary value); , attachment point on the neurocranium; , attachment point on the mandible; , resolving point of the vector A. Note that we have in reality a force A oriented from l to m and a force A′ (not represented on the graph at point l, superimposed, equal and opposed to A) oriented from m to l. (2) F, component responsible of mandible clamping; , application point of this force on the item. (3) K, component passing through the rotation centre (q) of the quadratomandibular joint. (4) , pressure component of the force K that passes through the axis of the quadrate q–x; , centre of the quadratocranial joint. (5) , component of the force K responsible for the retraction of the upper jaw (transmitted by the jugal bar); c, attachment point of the jugal bar on the upper jaw; a, resolving point of the vector . (6) , component of the force responsible for clamping of the upper jaw; , application point of this force on the item. (7) , pressure component of the force passing through the prokinetic hinge (b). The two pressure forces and make resultant from their intersection at point . As above, is the force vector resulting from the action of the adductors on the skull and slid to z; it is equal to but opposite to . The global equilibrium state results from the equality of the opposed forces and ', and and but is independent of the value taken for . (B) Modifications of the equilibrium in favour of the upper jaw (shaded and elements marked with ‴ ) or the mandible (not shaded and elements marked with ″) (see methods). Note that bill position in (A) is intermediate between the two positions illustrated here; so z would be located here halfway between z″ and z‴.
This graphical analysis allows us to locate easily point z for every position of a food item in the bill. Quadrate axis is always identifiable whereas that of the pressure from the upper jaw to the neurocranium varies with the location of point a, which moves according to the position of the item in the bill. However, point a is always on the jugal axis and is readily located by the clamping axis passing through the item. Thence it is easy to identify the axis of the upper jaw pressure on the neurocranium and to locate point z at its junction with the quadrate axis.
The graphical analysis allows us to see what happens when the bird changes the axis along which its adductors work (force ) which then does not pass anymore through point z (see Fig. 5B). When is more vertical and its axis frontal to point z, the retraction force is reduced and priority is given to the mandible, which rises and pushes up the upper jaw. Conversely, when is skewed downward and its axis caudal to point z, is larger and gives the advantage to the upper jaw, which pushes down the mandible. Thus by alternating the superiority of its jaws, the bird can work and roll the item in its bill.
3 Results: bony and muscular systems of the bill and hyoid apparatus
3.1 Skull
Motmot skull is remarkable by the length of the slightly decurved bill. The edges of the rhamphotheca are crenelated, with blunt teeth-like projections evenly spaced forward from nostril level. The upper jaw is rather massive, deep at base. The culmen extends forehead without any break in slope. The bill corner is set well back, level with the centre of the orbit. The prokinetic hinge is an almost straight thin and narrow transverse furrow. The bony blade that constitutes this hinge is so thin and weak that all examined dried specimens had the skull broken at this level. The nasal and maxillary bones and their palatal processes are pneumatized and swollen. The palate is desmognathic. The maxillary bones come into contact by their medial part, are fused along most of their length and form the main part of the buccal roof. Strangely, the caudal border of the upper jaw constitutes a quite vertical pneumatized wall.
The lacrimal (praefrontal) is missing in motmots and consequently, the orbit has no wall in its anterior part, only the lateral wing of the mesethmoid (or ectethmoid) being in front of the eye. Likewise as another effect of lack of lacrimal, the anterior part of the jugal is deprived of dorsal support. The postorbital process is particularly long. A quite long and stout zygomatic process of the squamosal extends almost up to the postorbital ligament. The palatines are relatively narrow, each one bearing medially a ventral and a dorsal small flange. The ventral flanges constitute the lateral walls of the choanae and each one bears a small rostral hook that supports an aponeurosis linked to the roof of the buccal cavity. The dorsal wings of the palatines join and fuse extensively along the medial line and caudally fuse also with the enlarged rostral parts of the pterygoids. Thence they wrap the ventral border of the interorbital wall and form a deep furrow, which firmly supports the sliding mechanism of the palate over the parasphenoid rostrum. The pterygoid is in wide contact with the quadrate, which is not particularly large comparatively to the height at base of the upper jaw (Fig. 1). Each quadrate bears a long orbital process, separated from the posterior wall of the orbit by a large gap. The condyles of the quadratocranial joint are almost spherical. The mandibular joint has clearly three condyles; the medial one is high and flattened with its axis almost in line with the longitudinal axis of the pterygoid. The caudal extremity of the mandible bears posterior, lateral and internal processes.
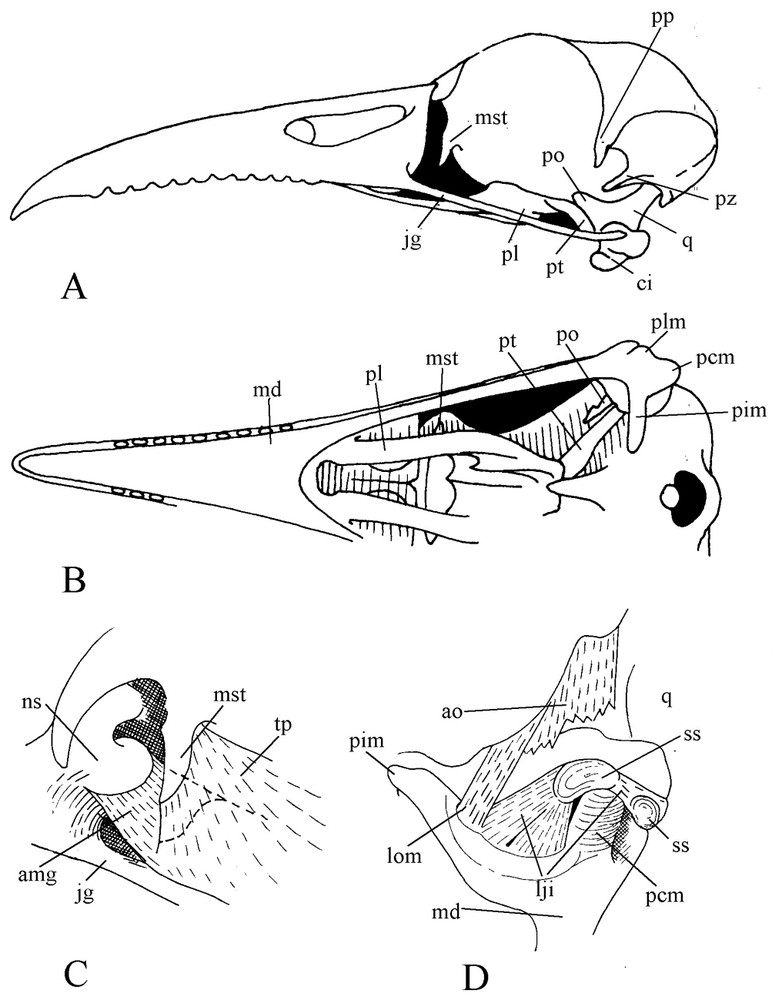
Skull of blue-crowned Motmot (Momotus momota). A: Lateral view. B: Ventral view. C: View of anterior part of the orbit. D: Caudal view of the mandibular articulation.
3.2 Ligaments
The postorbital ligament is well developed and originates at the base (not at the tip) of the postorbital process and passes along its posterior border. The external jugomandibular ligament is bound to the apex of the upwards bent caudal end of the jugal, and almost vertically from there joins the mandible together with the postorbital ligament. The internal jugomandibular ligament originates just in front of the dorsally flexed caudal portion of the jugal, in continuity with the longitudinal direction of the bone (Fig. 2). Passing above the caudal end of the mandible, this ligament spreads in a fan-like shape and is attached at the base of the internal process of the lower jaw along the quadrate joint border. It contains two sesamoid bones: one is located above the medial condyle of the quadrate, whereas the other is where the ligament crosses the mandible edge. The occipitomandibular ligament extends from the ventromedial edge of the otic wing at base of the occipital to the internal process of the mandible. An aponeurotic bridge joins the postorbital ligament to the jugal process of the squamosal. Below, another ligament is spread between the base of the postorbital ligament and the aponeurosis am. Removal of the eye shows that the anterior part of the large superficial aponeurosis is folded medially and lies along the caudal face of the swollen maxillary process of the nasal, almost up to the interorbital wall. Caudal to its attachment on the upper jaw, this aponeurosis is bound to the lateral edge of the periorbital aponeurosis.
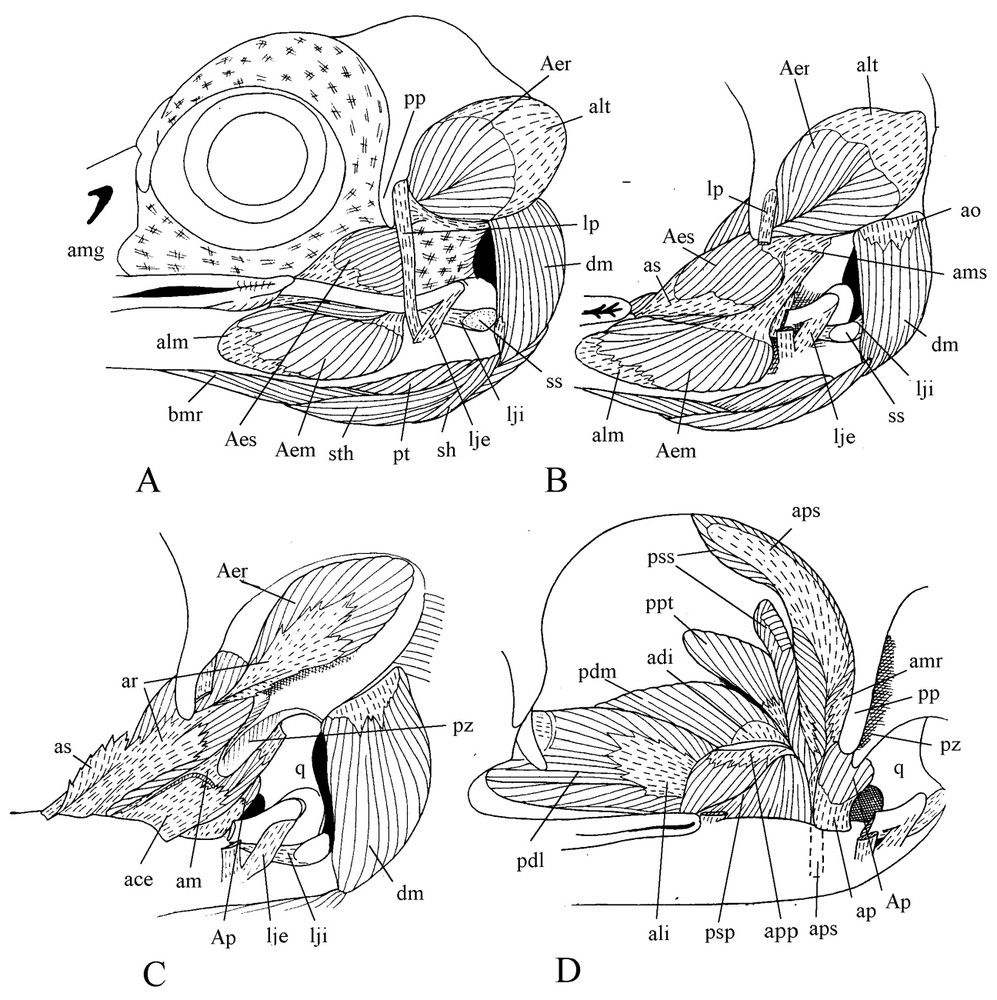
Cranial musculature. Lateral views of various planes of the dissection ().
3.3 Cranial musculature
The powerful cranial musculature appears when both the eye and the large superficial aponeurosis are removed. The large medial portion of the external mandible adductor spreads from under the jugal over a great part of the lateral surface of the caudal part of the mandible and reaches the buccal rictus where it is inserted by the aponeurosis alm. This medial portion of the external mandible adductor originates by the aponeurosis am, which is attached to the long jugal process of the squamosal and, to a lesser extent, to the otic process of the quadrate. From the aponeurosis am rise the dorsorostral and transversal sheets of the aponeurosis ams, which are part of the well-developed superficial portion of the external mandible adductor. The contractile fibres of this part are inserted on the aponeurosis as, a lateral blade of ar, the aponeurosis for insertion of the rostral part of the deep portion of the adductor (Fig. 2C). This part lies in the temporal fossa and contains two aponeuroses of origin, alt and amr. The aponeurosis ace of the caudal part of the deep portion of the external mandible adductor rises from the lower part of ar and spreads backwards along the mandible edge. Its muscular fibres are spread in the space between the jugal process of the squamosal and the otic process of the quadrate.
Contrary to what is found in other Coraciiformes, m. pseudotemporalis superficialis is quite enlarged with an extended lateral and a smaller medial portions. Fibres of the lateral portion fill almost half of the surface of the posterior wall of the orbit. They are very short (1.5 to 2 mm) and are inserted on the large wing of aps. This aponeurosis narrows and becomes a strong cylindrical tendon before inserting on an outgrowth of the mandible present in front of the mandibular articulation. The two parts of the muscle are separated by the insertion of the m. tensor periorbitae (Fig. 2D). M. pseudotemporalis profundus is also well developed. It extends from a wide part of the orbital process of the quadrate to a large surface of the medial part of the mandible. It is sharply separated from the posterior adductor by the insertion aponeurosis of m. pseudotemporalis superficialis. Mm. protractor pterygoidei and Mm. protractor quadrati are difficult to tell apart.
M. pterygoideus shows a noteworthy trend toward a longitudinal orientation along the medial line of the palate (Fig. 3). The aponeuroses avo and alo by which originates the ventral part of this muscle are separated by a fascicle of muscle fibres spread from the insertion aponeurosis avi over the palatine, filling the hollow of this bone. The aponeurosis avo is attached to the edge of the ventral wing of the palatine, bordering the choana. The aponeurosis alo is also attached to the lateral border of the palatine, and with the mucosa of the palate covers the wide gap between the palatine and jugal bar. The medial edge of alo sinks into the muscle and gives the wing . From avo, muscular fibres reach the aponeurosis avi, which is attached near top of the internal process of the mandible. The insertion aponeurosis ali of the lateral part of m. pterygoideus is attached at base of the internal process of the mandible. At its surface muscular fibres coming from the palatine and the dorsal face of alo constitute the dorsolateral part of m. pterygoideus. As is often the case in birds, the dorsomedial part of this latter shows a steep slanting orientation relative to the sagittal plane and contains two aponeuroses: a small origin one and a larger insertion one. At last, medial to the pterygoid is the caudal part of m. pterygoideus without aponeurosis.
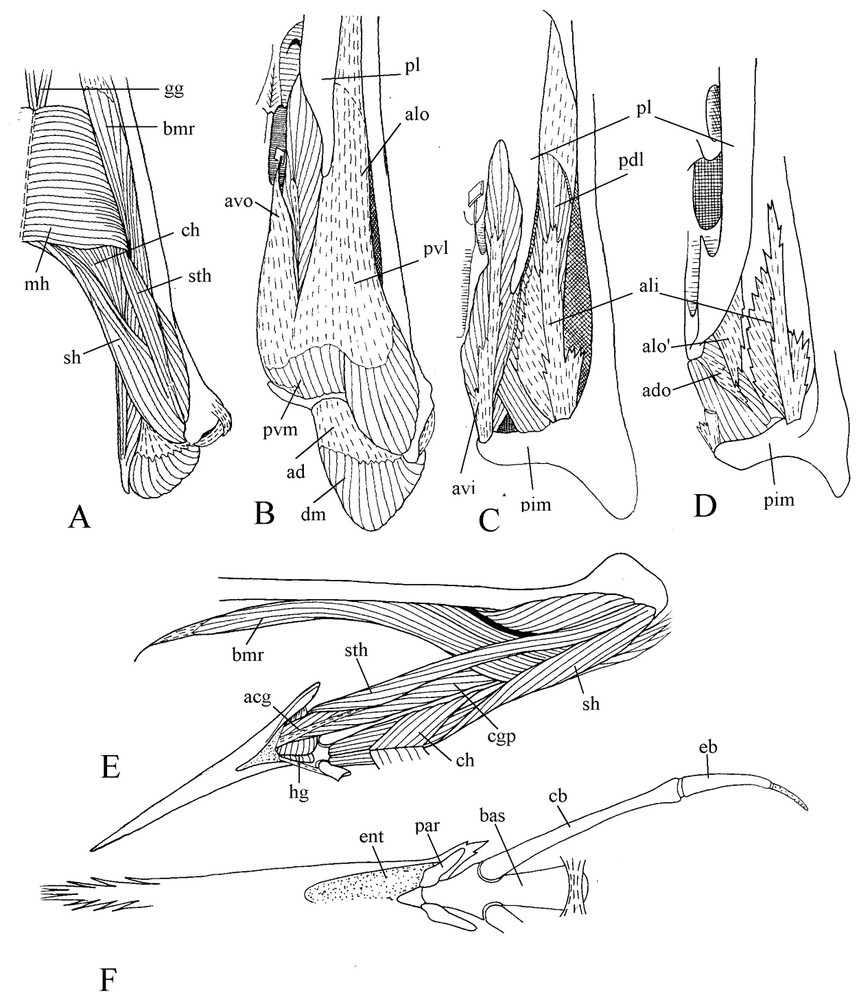
Ventral views of successive planes of the dissection of m. pterygoideus (A, B, C, D). Lateral view of the ventrally displaced hyoid apparatus (E). Ventral view of the bony hyoid apparatus (F).
The protractors of the pterygoid and quadrate fill the wide gap between the internal surface of the orbital process of the quadrate and the interorbital wall.
M. depressor mandibulae has two aponeuroses: the origin aponeurosis ao, which begins along the lateral end of the otic wing of the occipital, and the insertion aponeurosis ad attached to the caudal end of the mandible.
3.4 Hyoid apparatus
Rather full information on motmot hyoid apparatus is found in Burton [5], and illustrated by Fig. 3 (E and F). The tongue is flat with a brush-like tip. Articulating with the basihyale, the paraglossalia are ossified with strong keratinised projections at their posterolateral part. The rostral part of the basihyale bends upwards, so the tongue articulation is located more dorsally than the attachment place of the rather short hyoid horns mainly constituted by the ossified but elastic ceratobranchiales. Contrary to various other birds, motmot has hyoid horns that do not turn round the depressor of the mandible but are applied against the lower surface of the pterygoid muscle.
With regard to hyoid musculature, let us mention briefly here that m. mylohyoideus is located under the tongue, m. branchiomandibularis is well developed though relatively short, and there are no mm. ceratoglossi anteriores.
4 Discussion: functional analysis of the bill and hyoid apparatus
4.1 High kinetism of the bill apparatus
Quite ample pro- and retraction movements of the upper jaw are easily simulated when syndesmological preparations of motmot heads are manipulated and a series of morphological cues show that a high degree of cranial kinesis is one of the clearest morphofunctional properties of motmots.
Particularly obvious is the structure of the flexible zone, i.e., a very thin bony sheet in the bottom of a straight transverse slit between the jaw and the neurocranium. Such a structure helps to reduce the resistance of the prokinetic hinge to the forces that govern upper jaw movements with consequently an increase of its mobility. Although this bony sheet is very thin, both stability and mobility of the area are insured by a syndesmosis, the slit being filled with conjunctive tissue.
Great amplitude of jaw movement relies also on the particularly wide cranio-facial gap between the vertical posterior end of the upper jaw and the anterior edge of the interorbital wall. Furthermore, jaw protraction is possible only until the orbital process of the quadrate abuts against the posterior wall of the orbit. However, movement is still more limited by the presence of protractor muscles in the gap between the orbital process of the quadrate and the posterior wall of the orbit. So, jaw movement would be limited by the size of this gap but syndesmological simulations show that, in motmot, some movement of the quadrate is still possible even when the upper jaw is extremely protracted. Likewise, the almost vertical orientation of the external jugomandibular ligament is another indication of high cranial kinesis. As in mousebirds [24], this peculiarity is linked to a high freedom of upper jaw movements.
A wide range of movements needs security mechanisms that increase the stability of the shape of the palate mechanism. The mandibular joint is particularly concerned in this respect. Its security is increased by the internal jugomandibular ligament, which has a very enlarged posterior part containing sesamoids and which, caudal to the joint, fills the gap between the lateral and posterior processes of the mandible. Other mechanisms that enhance palate stability during forceful movements of the upper jaw include the wide contact between the pterygoid and the quadrate as well as between the pterygoid and the palatine that slide along the lower edge of the interorbital wall.
4.2 Role of the crenate edges of the rhamphoteca: a system of saws?
Such a striking morphofunctional specificity of the motmot bill apparatus gets us to look for a better interpretation than the simplistic one of a gape merely adapted to swallow the relatively large preys the bird is known to feed on. First we may ask why the upper jaw is so high at base; its thickness looks maximal and the frontal angle is missing. Such a dorsocaudal displacement of the flexible area that has even induced a lack of identifiable lacrimal must have come under particular circumstances. The existing connections between the periorbital aponeurosis and the rostral part of the large superficial aponeurosis as well as the fact that the latter is directly attached to the upper jaw (not on the lacrimal as usual in most other birds) let suppose that, in motmots, the lacrimal has not disappeared, but is incorporated into the upper jaw. The answer requires an embryological study.
In the upper jaw, a high base coupled to a quite high kinetism has for main functional consequence an increased longitudinal mobility of the crenate edges of the rhamphotheca [25]. Neognathae with a generalised bill apparatus are able to roll food items by changing the balance of forces alternatively to the advantage of one or the other jaw [8]. In motmots, this ability has been supplemented by the transformation of the jaws into an interactive system of saws. Albeit direct observations of such saw-like actions are missing, morphofunctional characters of motmots are so striking as is their habit to keep for long relatively large food items in their bill before they swallow them that we cannot avoid to see in all these peculiarities a major system that enables these birds to soften and cut before ingestion rather large plant or animal items important for their diet. Saw-like edges of rhamphotheca can work in two ways. First, when the food item is fixed, each jaw can effectively saw it; it is probably like this that the jaws act when they clamp a relatively large item (e.g., a snake) that does not roll between them. Second, when the item rolls along the jaws, crenelations pierce through it and make it softer, more pliant and suitable for ingestion; in motmots such a food processing would have evolved from an ancestral one based on a vigorous smashing of the prey on a hard support.
4.3 Role of the tongue
During this food processing, it is easy to see how the tongue can help turn items up so that their intact parts are progressively brought in contact with crenelations.
In motmot, the particular tongue elevation mechanism plays an important role during intraoral processing of food items. In many Neognathae, tongue elevation mechanism consists in that the caudal part of m. branchiomandibularis leans against the surface of the mandible depressor and works as the stiff string of the bow made up by the long flexible hyoid horn [26,27]. The reaction force from the depressor and the force of m. serpihyoideus give a couple of forces that rotates the hyoid and raises the tongue towards the palate (Fig. 4B). The joint action of the tongue and the upper jaw allows move of the item into the buccal cavity.
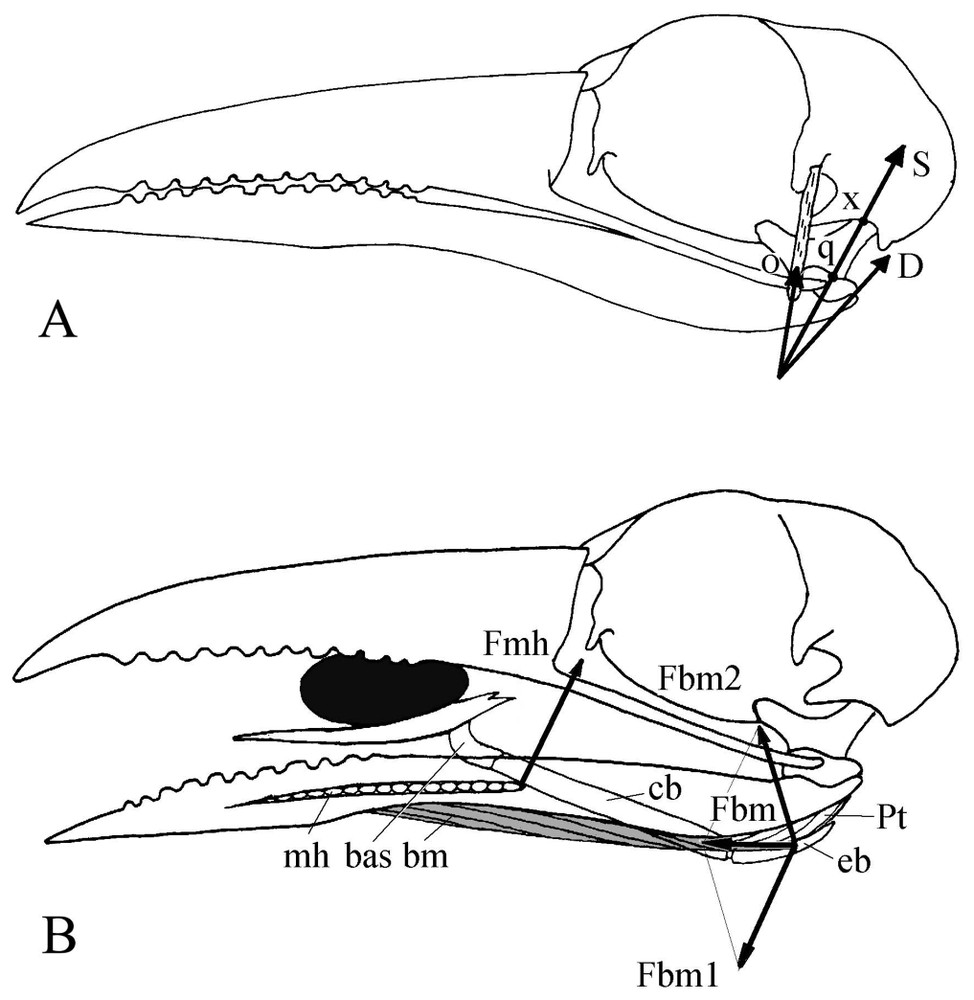
(A) Mechanism of the postorbital ligament in motmots. D: Force from the mandible depressor, O: tension (reaction) force from the postorbital ligament, S: resultant of the forces O and D. In motmots S works along the quadrate axis q–x and is completely absorbed by the neurocranium. Because of this position and contrary to what happens in many other birds, this single force cannot be decomposed at the quadratomandibular joint q and thus there is no protraction of the upper jaw produced when the mandible is depressed. (B) Mechanism for tongue elevation. Fbm1, Fbm2: components of the force Fbm from branchiomandibular muscle (shaded). Fmh: reaction force of mylohyoideus muscle. Fmh and Fbm1: pair of forces that turns the hyoid apparatus upwards.
In motmot, the stiff hyoid horn is applied against the pterygoid muscle, not the depressor (Fig. 4B). In that case, the force of the rather short m. brachiomandibularis and that of the m. mylohyoideus make a couple that rotates the hyoid and elevates the tongue. The dorsally oriented rostral part of the basihyale helps also to raise the tongue towards the palate beyond the level usually permitted in birds by m. mylohyoideus. The motmot tongue is thus able to process an item within the entire space between the jaws even with a wide-open bill.
4.4 Balance of forces during food processing
This capacity of saw-like movements of jaws reflects an intrinsic property of the motmot bill apparatus, which works according to the first type of jaw clamping [8,23], called ‘joint muscular control’, where the adductors act simultaneously on both jaws and can change the equilibrium of forces in favour of one or the other jaw.
The graphical method proposed by Dzerzhinsky [8] shows (Fig. 5A) that when an item is clamped in the medial part of the bill, both jaws exert equal forces () if the resultant of forces from the dorsal (external and superficial and deep pseudotemporal) adductors passes at point z. If the vector of this resultant passes beneath z, the equilibrium is changed in favour of the upper jaw, which is retracted. If it passes above z, the equilibrium is changed for the benefit of the mandible, and the upper jaw is protracted.
As seen above, in motmots all the muscles correcting for the orientation of the vector are proportionate and well developed. The superficial pseudotemporal is almost vertical and quite strong for a Coraciiformes; it can change the balance of forces to the advantage of the mandible. The deep pseudotemporal retracts the quadrate and thus insures superiority of the upper jaw; the force from this muscle passes always through the quadratocranial articulation (i.e., beneath z [8,23]). In motmots, the superficial pseudotemporal may be a secondary reinforcement through a remarkable enlargement of its lateral part. Its medial part, mainly transformed into a tendon, is probably ancestral, and corresponds to the muscle characteristic of several Coraciiformes [5,7]. The general disposition of the external adductor and the orientation of its main aponeuroses (mainly ar and am) show that the vector of the force it exerts passes a little beneath the point z found when the food item is pressed in the medial part of the bill. Likewise, Dzerzhinsky [8] showed that when the item is clamped at the tip of the bill, the resultant of all forces from the dorsal adductors, thanks to a supplementary effort from the deep pseudotemporal, passes beneath this same point z. As the item is moved towards the base of the bill and in order to maintain the balance of forces from the jaws, the resultant force from the dorsal adductors must (and thanks to a supplementary effort from the superficial pseudotemporal) be more vertically oriented than shown in Fig. 5A. In all cases, if the balance of forces is changed, for instance by a particular effort of the deep pseudotemporal, the upper jaw is retracted, and the clamped item rolls along between the crenate jaws. When retraction is maximal, a further contraction of the superficial pseudotemporal induces adduction of the mandible and consequently the item rolls in the opposite direction.
Jaw protraction and retraction result in changes of the orientation of the line of action of the adductors resultant and of the quadrate axis. Dzerzhinsky [8] showed that when the balance of forces is changing in favour of one or the other jaw, three conditions can be considered for the direction of the resultant vector of forces from the dorsal adductors. First, when this vector passes through point z, whatever the position of the jaws, the system is in a state of neutral balance. Second, when this resultant vector tilts faster than the quadrate axis, some resistance to this change of equilibrium will always develop on the side of the disadvantaged jaw and the system is then in a state of stable equilibrium. Third, when this resultant vector tilts slower, the system gets into a complex unstable equilibrium, where, as shown by [8], while they clamp forcefully a food item and as their clamping force increases, the jaws can reach extreme pro- or retraction, a situation that can even damage the mechanisms that limit these movements.
We looked for which of these three situations is best suited to the motmot bill apparatus. We used the same graphical method as above (Fig. 5A) to find out the location of the resultant of forces from the dorsal adductors when the bill is either protracted or retracted. In both cases, the resultant passes through the same point l (Fig. 5B), conventionally considered as the attachment point of the adductors to the neurocranium. So, in that case, there is probably a neutral equilibrium when, during ample pro- and retraction movements, the item is clamped by the resultant of all dorsal adductors and rolls along the jaws without giving rise to any risk of unstable equilibrium.
The use of simultaneous forces from the powerful dorsal adductors for an efficient processing of a food item requires in motmots a pterygoid muscle especially adapted by its particular longitudinal orientation to transmit their resultant to the upper jaw.
4.5 Role of the postorbital ligament
In such a food processing that, according to the morphofunctional peculiarities of the bill apparatus, seems a major one for motmots, the well-developed postorbital ligament could be seen as undesirably locking on mutual movements of the jaws. The role of this ligament has been defined in birds [28–32], and a thorough synthesis of the various discussions has been done by Dzerzhinsky [8]. It slings the mandible where its attachment point works as a rotation centre of this jaw: when the ligament becomes taut, it pulls up the posterior part of the mandible as this latter depresses. So the mandible would rotate around the attachment point of the ligament and press the quadrate both forward and upward. The force is transmitted through the jugal bars, palatines and pterygoids to the upper jaw, which automatically rises (coupled kinesis, sensu [30]). We consider as particularly important Dzerzhinsky's conclusion [8] that to guarantee this coupled kinesis, the postorbital ligament must also be stretched by a medially oriented force. This one originates from the dorsomedial part of the pterygoid muscle and from the quadrate and pterygoid protractors. Though its role is generally undeniable, the postorbital ligament appears weak or even almost lacking in some cases of feeding adaptations with great mutual mobility of the jaws (e.g., in cuckoos [23] and bee-eaters [pers. obs.]). A rapid glimpse would give the false impression that because of its great development, the motmot postorbital ligament is quite important for the bill apparatus to work. However, available data on this ligament that define the main aspects of the bill apparatus functioning in other Coraciiformes [6,7] show that in fact motmot has a weaken ligament comparatively to that of these species. For instance, in Coraciidae, it is responsible of the automatic closure of the bill when, in flight, snatched prey hits the palate of the hunting bird [6,7]. In that case, the mainly ossified ligament is almost always stretched, i.e., ‘ready to work’. In motmot, there is no such ossification: the ligament passes along the posterior edge of a long postorbital process, but is in no way an extension of it.
Applied to motmot (Fig. 4A), Dzerzhinsky's [8] graphical method of analysis of the cooperation between the postorbital ligament and the mandible depressor shows that when the mandible is depressed, the protraction force of the upper jaw seems so trifling or even lacking that we can probably doubt it is effective. The resultant of the forces from the depressor and postorbital ligament indeed passes almost through the quadratocranial joint (Fig. 4A). Hence motmot shows a trend towards a reduced coupled kinesis (sensu [30]) associated with the adaptation to a particular food item processing between the jaws. Nevertheless, although the geometric configuration of the postorbital ligament mechanism reduces the transformation of the depressor force into a protraction force of the upper jaw, it is quite useful for the reverse, i.e., mandible adduction by the tension force of the postorbital ligament.
5 Conclusion
The width of the prey size spectrum in birds depends on their ability to swallow large food items. Most of the time they do this by tearing pieces from the item (e.g., diurnal raptors). As the distance between the mandible rami limits prey size, a bird will swallow a large item as a whole either because it has (e.g., owls) a wide gape or because its streptognathism [33] allows mandible spreading (e.g., gulls). The morphofunctional analysis of the motmot bill apparatus showed a highly distinctive adaptation of this bird to use mutual jaw movements when processing a food item with the crenate edges of its rhamphotheca. This enables it to soften progressively a large prey, making it more and more pliable, easy to distort and suited for ingestion. These food-processing methods allow the bird to widen its prey-size spectrum. Such ability may be a specificity of every motmot bill apparatus because in almost all examined species we found distinct crenelations or at least hint of serrations along rhamphotheca edges. These morphofunctional conclusions call of course for rigorous, e.g., kinematic testing of the inferred food processing potentialities of motmots.
Acknowledgements
We are most grateful to the curators in the ‘Muséum national d'histoire naturelle’, Paris, who gave us access to the material in their care, to Professors Felix J. Dzerzhinsky and Adrien Casinos, and two anonymous referees for useful comments and suggestions. This study benefited from grants RFBR No. 03-04-48958, RFBR Nos. NS-1808 2003 04 and UR N-07-03-003.