1 Introduction
Quinol-oxidizing complexes – the cytochrome bc1 complex of mitochondria and bacteria or its chloroplast counterpart, the cytochrome complex – are the most widespread protein complexes among energy-transducing membranes. They oxidize quinol while reducing a soluble electron carrier to generate an electrochemical gradient of protons across the membrane. According to the Q-cycle proposed by Mitchell [1], the oxidation of a plastoquinol (PQH2) at the Qo site (lumen) releases two protons and two electrons, one of which reduces a high-potential chain formed by the Rieske protein and a c-type cytochrome – cytochrome f in the case of cytochrome complexes – both of which are membrane-bound. The second electron is transferred to cytochrome then to cytochrome , which together constitute the low-potential chain. Re-oxidation of hemes and occurs at the Qi site (facing the stroma) through the reduction of a plastoquinone (PQ) molecule, generating a cyclic electron transfer around the b heme chain.
While numerous data and mutation studies have provided a fairly good description of the Qo site from both cytochrome [2,3] and bc1 (for a review see [4]) complexes, much less is known about structure/function relationships within the Qi site. Some information on the localisation of the Qi site in bc1 complexes were drawn from mutagenesis approaches and studies of inhibitor resistance in bacteria and yeast [4–6]. By contrast, information pertaining to the residues that form the Qi site of cytochrome complex and to its structure has long remained scarce. Before the cytochrome structure was solved at atomic resolution in both Chlamydomonas reinhardtii and Mastigocladus laminosus [7,8], our structural knowledge on this protein heavily depended on predictions based on the structure of the homologous complex [9–11]. Indeed, the major strategy to design site-directed mutations of the cytochrome complex was based on sequence homology between cytochromes and bc1. As will be described here, this strategy turned out to be poorly successful for the study of the Qi site.
Despite their similarities, bc1 and complexes feature fundamental functional and structural differences. In oxygenic photosynthesis, the electron pathway can switch from a linear mode, producing both ATP and reducing equivalents, to a cyclic one, where electrons transferred from cytochrome to PSI on the luminal face of the membrane return to complex on the stromal face, with production of ATP, but not of reducing equivalents. In addition, the complex, by activating a protein kinase, plays a key role in state transitions, a major regulatory process of the photosynthetic apparatus that has no counterpart in the respiratory chains [3,12,13]. Finally, cytochrome , as compared to cytochrome bc1, accommodates three additional cofactors, whose function still remains poorly understood: a chlorophyll a, a β-carotene and a heme named , with [14,15]. The discovery in the X-ray structure of this additional heme, which contributes to the formation of the Qi site, was a major surprise and gave an important boost to the study of structure/function relationships of cytochrome 's Qi site. Since this site has to accommodate both a substrate and heme , its structure indeed is quite different from that of cytochrome bc1, even if the polypeptide chain around Qi has the same topology in the and bc1 complexes. This could account for the lack of inhibitory effect of antimycin on cytochrome and, in fine, for strong discrepancies between the two complexes that have long remained unexplained.
The scarcity of mutagenesis data also originates from the fact that cyanobacteria do not tolerate severe alterations in the function of the complexes, while site-directed mutagenesis of cytochrome subunits remains technically difficult in land plants. Since the green alga C. reinhardtii shows dispensable photosynthesis and is amenable to chloroplast gene transformation, it is best suited for structure/function relationship studies of the Qi site [16,17].
In C. reinhardtii the cytochrome complex comprises four major subunits [15]: the Rieske protein, binding a Fe2S2 cluster, is encoded by the nuclear gene petC while the chloroplast genes petA, petB and petD respectively code for cytochrome f – a c-type cytochrome with of +330 mV [14] –, cytochrome , which binds the , and hemes, with s of −84 mV, −158 mV and −100 mV [14,15] – and subunit IV. Sequence comparisons have shown that cytochrome and subunit IV from cytochrome are respectively homologous to the N- and C-terminal parts of mitochondrial and bacterial cytochrome b [18]. The complex also comprises four additional small subunits, the products of the chloroplast genes petG and petL and of the nuclear genes PETM and PETN [15,19–22], which have no homologues in the cytochrome bc1 complex.
Here, we critically re-examine our previous attempts to obtain mutants of the Qi site, impossible to interpret in the absence of the 3-D structure. Mutant construction was based on sequence and structure homology between cytochromes and bc1, which display, as described previously, fundamental functional and structural differences. The recent X-ray structure of complex [8] has allowed a successful strategy of mutagenesis, but even then we faced some paradoxes, which we will try to discuss.
2 Results
2.1 Before the structure
In cytochrome bc1 the Qi site is formed by cytochrome b and most mutations altering the functionality of the Qi site target the amino-terminal part of cytochrome b or the de loop connecting helices D and E [4]. Cytochrome and subunit IV from cytochrome are homologous to the N- and C-terminal parts, respectively, of mitochondrial and bacterial cytochrome b [18]. It was therefore expected that both subunits would contribute to the formation of the Qi site.
Two residues in helix A from cytochrome of C. reinhardtii, bG37 and bF41, were targeted since substitutions of the corresponding residues in Rhodobacter's cytochrome b (G48 and A52 respectively) had provided interesting results [23]: in Rhodobacter, substitution of G48 by an Ala residue preserved a wild-type phenotype in the G48A mutant, but replacements with Val or Asp residues led to impaired re-oxidation of causing the loss of photosynthetic growth capability or to failure to assemble the bc1 complex respectively; when Rhodobacter residue A52, equivalent to residue bF41 in C. reinhardtii, was changed into a Val, the resulting mutant strain displayed a 5-fold slowing down of Qi site activity and became resistant to antimycin, a specific inhibitor of the bc1 Qi site that has no effect on the complex [23].
By site-directed mutagenesis of plasmid pWB encompassing the petB coding sequence [24], we substituted the conserved Gly37 of cytochrome by Val or Asn residues in order to change the local bulkiness and polarity respectively, while residue Phe41 was mutated to Gly to reduce the local bulkiness (Fig. 1). In addition, we also substituted for Ala the residue Phe44, located one helix turn further from the conserved Gly37.
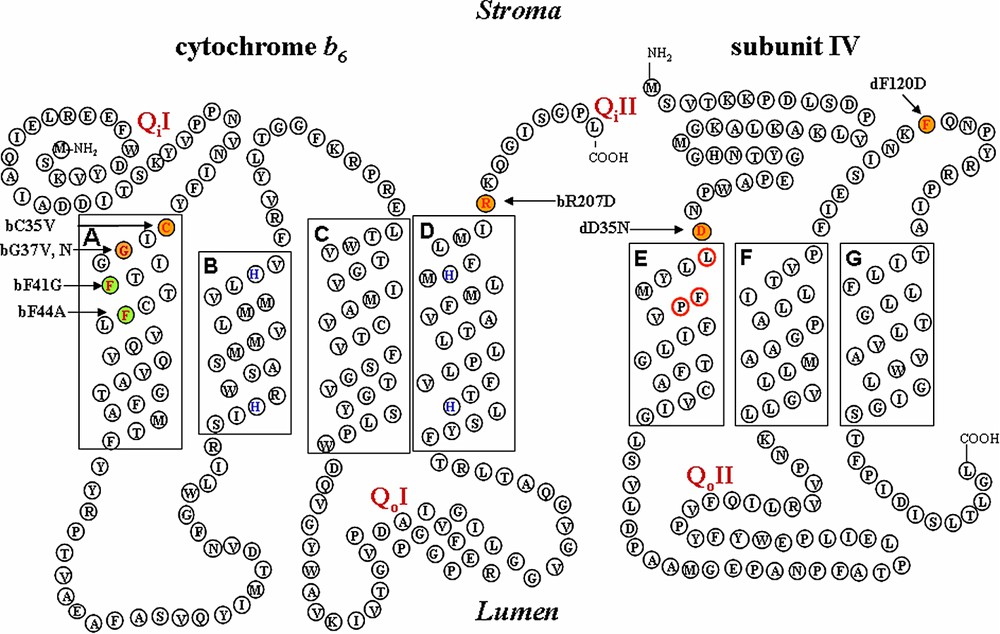
Schematic representation of cytochrome /subunit IV regions forming the Qi site. Mutated residues are indicated in colors: orange: non photosynthetic mutants; green: photosynthetic mutants; yellow: mutants selected on spectinomycin resistance.
These mutated petB genes were introduced to the chloroplast genome of C. reinhardtii by biolistic transformation, according to two strategies used in parallel (see Section 4). In the first one, deletion strains – here ΔpetB [24] – were used as recipient and transformed clones were selected on minimum medium for the recovery of photosynthetic capability, when the mutations preserve the function of the cytochrome complex. In the second one, the mutations were first associated with the aadA [25] cassette, conferring resistance to the antibiotic spectinomycin, inserted into a neutral site [26]. Transformed clones, derived from a wild-type recipient strain, could be recovered on spectinomycin-supplemented medium, even when defective for cytochrome complex activity.
Two mutants (bF41G and bF44A) were recovered after transformation of strain ΔpetB, following the capability of the deleted strain to recover photosynthesis. Characterisation of these mutant strains did not reveal any drastic changes, since they accumulated the complex to a wild-type level, were still sensitive to the NQNO inhibitor of the Qi site, and showed normal turnover of the complex [27].
By contrast, no phototrophic clones could be recovered upon transformation of the ΔpetB strain with petB genes carrying substitutions of the Gly37 residue, suggesting that these mutations are detrimental to photosynthesis. Consistently, spectinomycin-resistant transformants obtained from the wild-type recipient strain displayed fluorescence induction kinetics typical of mutants with impaired cytochrome activity [26,28], while Western blot analysis revealed that cytochrome subunits fail to accumulate. Since similar substitutions in the complex have widely different effects, our results pointed to some structural differences between the Qi sites of the two complexes, as was already suggested by their different sensitivity to inhibitors [29].
The structure of the cytochrome bc1 complex that became available in the late 1990s [9,11,30] (PDB accession 1bcc and 3bcc) allowed us to select other residues putatively contributing to the formation of the Qi site. We chose the residues Arg207 at the C-terminus of cytochrome , which we substituted for Asp, carrying an opposite charge, as well as residues Asp35 and Phe120, at the N-terminus and in the fg loop of subunit IV, which we modified to Asn and Asp respectively, in order to change both bulkiness and polarity (Fig. 1). The wild-type ΔpetB or ΔpetD strain – this latter carrying a deletion of the petD gene [24] – was transformed with site-directed mutagenised plasmid pWB or pWQ [24], with and without the aadA selectable marker [25]. Again, we could not recover phototrophic clones upon transformation of the ΔpetB or ΔpetD deletion strains and, indeed, the mutants selected for resistance to spectinomycin lacked any accumulation of the complex (Fig. 2).
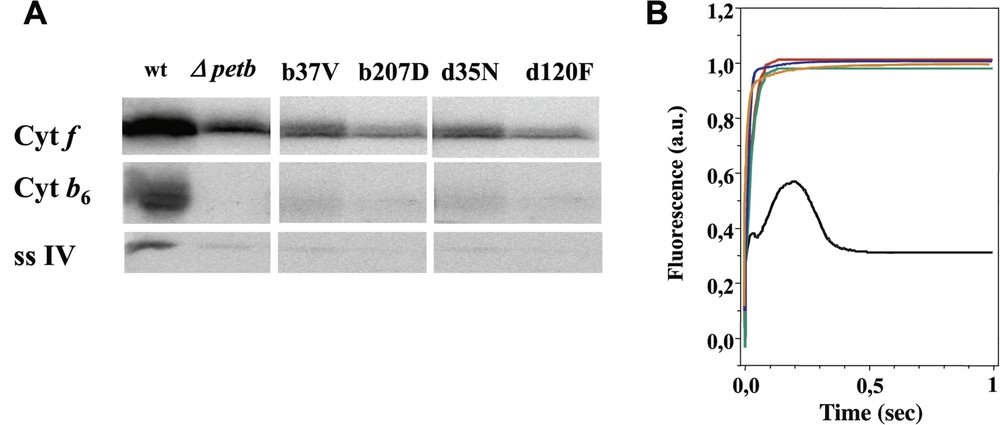
Panel A: Immunoblot against subunits and IV in wild type and Qi mutant strains; the recipient deletion strain ΔpetB is also shown as a control. Panel B: fluorescence induction kinetics of wild type and Qi mutant strains. Black: wild type, orange: bG37V, green: bR207D, red: dD35N, blue: dF120D.
We also substituted a Val for Cys35, a residue specifically conserved in all cytochrome , but only present in those very few cytochromes b that retain a heme upon denaturation by SDS (see Ref. [31]). Meanwhile, Cys35 was identified by X-ray diffraction as the residue to which heme is covalently attached [8]. The C35V mutant was unable to grow phototrophically because this substitution prevented the accumulation of cytochrome subunits [32].
2.2 After the structure
We have already pointed out how the unexpected discovery of the heme in the Qi site of the cytochrome complex [7,8] has given a new impetus to the study of structure/function relationships in cytochrome . The crystallographic structure provided a sound structural background to the construction of new mutants altering the Qi site. We chose three residues in the proximity of the heme: Leu36, Phe40 and Pro41 of subunit IV (Figs. 1 and 3). Leu36, standing at the entrance to the Qi site, was substituted for Ala to enlarge the entrance of this site, while Tyr/His or Leu residues were substituted for Phe40 that may shield the iron of heme from the external medium so as to provide a possible axial ligand to iron of heme or to test its protective role. We also tested whether residue Pro41 imposed structural constraints to the environment of heme by mutating it for an Ala residue.
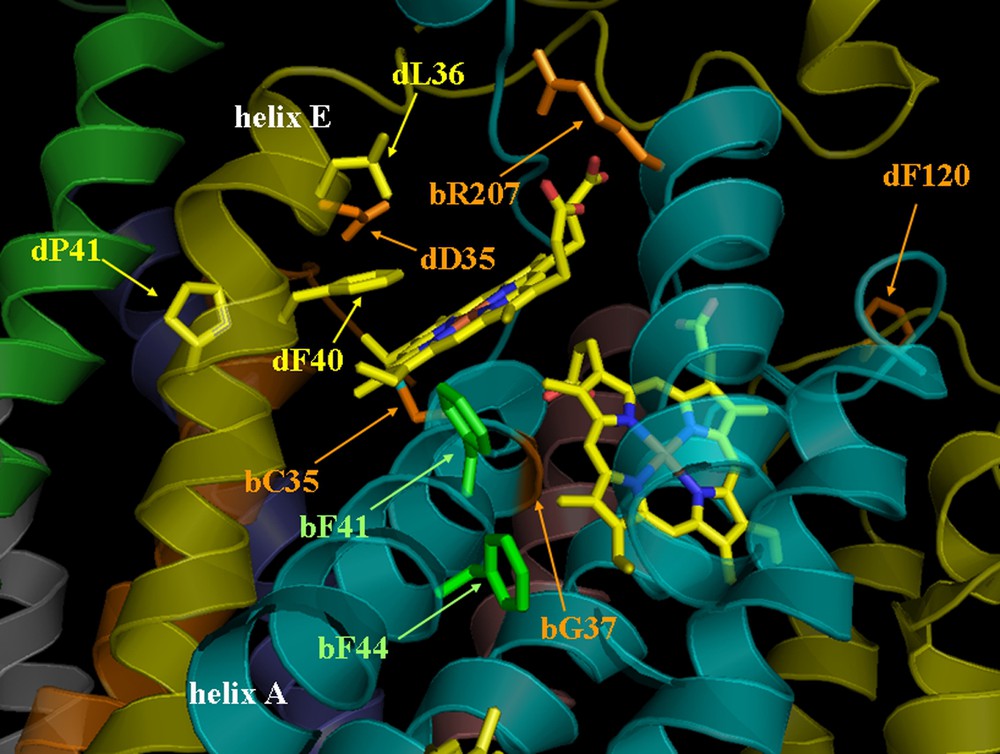
View of Qi site (1Q9 pdb data). Mutated residues are located in the structure; in orange: mutations leading to non-assembled complexes and non-photosynthetic strains (on cytochrome : bC35V, bG37V, bR207D and on subunit IV: dD35N, dF120D), in green: mutations leading to photosynthetic strains (on cytochrome , bF41G, bF44G), and in yellow: mutations leading to photosynthetic strains that could only be selected on spectinomycin (on subunit IV: dL36A, dF40L/H/Y and dP41A).
Our attempts to recover phototrophic clones upon transformation of the ΔpetD strain were unsuccessful, leading us to suppose that also these mutations were detrimental for photosynthesis. We then used the wild-type strain as a recipient for transformation experiments with plasmids carrying the specific mutations associated with the aadA cassette. Unexpectedly, the transformed clones recovered on antibiotic-supplemented medium retained wild-type fluorescence induction kinetics, even after they reached homoplasmy for the mutation (when all copies of the polyploid chloroplast genome carry the mutation while all wild-type versions of the petD gene have been eliminated). Paradoxically, the mutations therefore were not harmful to photosynthesis, even though the mutated genes were not able to rescue deletion mutants upon transformation.
3 Discussion
For more than ten years, we have tackled the ‘mystery’ of the Qi site. The resolution of the 3-D structure of the cytochrome complex with the discovery of an additional heme in the Qi site has partially solved this puzzle and shed a new light on the molecular organisation of this site. Heme has quite disconcerting features, such as a single thioether linkage to the protein, no amino acid side chain serving as an axial ligand, and, possibly, a molecule of water or an hydroxide ion as a fifth putative ligand. Since we are not able yet to draw a precise model for the role of the heme, the critical analysis of previous alterations to the Qi site may help us to understand its peculiar structure and organisation.
The mutated amino acids can now be located on the 3-D structure (Fig. 3) [8] in order to ascertain the structural effects of the mutations on the Qi site environment and to correlate it with the corresponding phenotype.
Gly37 of cytochrome (Fig. 3) residue coloured in red on the cytochrome helix A in cyan), is at 3.7 Å from the heme porphyrin cycle, with which it may be in Van der Waals contact. Accommodating a Val residue in mutant bG37V may require a displacement of helix A, which is possible in cytochrome bc1, since the corresponding mutant does not display any effect on the assembly. By contrast, the thioether linkage between heme and Cys35 at the stromal end of helix A requires a precise protein environment and this displacement probably prevents cytochrome assembly, as suggested by the phenotype of the mutant. Similarly, substitution of Cys35 (Fig. 3, residue coloured in orange on the cytochrome , helix A in cyan) led to a phenotype much very similar to that of mutants in C. reinhardtii [32,33]. This Cys residue is conserved in all species carrying a heme covalently bound to the protein moiety, either on chloroplast cytochrome or on cytochrome b from thermophilic bacilli [34].
The detrimental effect of substitution for an Asp of residue Arg207 (Fig. 3, residue coloured in orange on the cytochrome , helix D in cyan), which interacts with the propionate group of heme , may be related to the radical change in the Qi local charge. Arg207 participating to a network that involves propionate, water molecules and plastoquinone, the substitution probably modifies the interaction between plastoquinone and heme .
Substitutions of the two Phe residues of cytochrome in positions 41 and 44 (Fig. 3, residues coloured in green on the cytochrome , helix A in cyan) led to a wild-type phenotype. These residues are far from the Qi site and point rather towards the heme. A reduction in their bulkiness did alter neither the folding nor the accumulation of the complex [27].
Two other mutations were carried out on subunit IV (Figs. 1 and 3), both of which yielded non-phototrophic mutants. Residue Asp35 (residue coloured in orange on the subunit IV, helix E in yellow) is in strong electrostatic interaction with residue Arg95 from the PetN subunit. Its substitution with Asn by removing the negative charge changes the local polarity, which seems important for the conformation of the site and the stability/assembly of the complex, as shown by the phenotype of the mutant. It is more difficult to explain the detrimental effect of the Phe120 → D mutation, since residue dPhe120 (coloured in orange on the subunit IV, fg loop in yellow) is located quite far away from the Qi site and does not seem to interact with other cofactors. However, Phe120 is close to a positively charged patch (Arg112 on cytochrome , Tyr119 and Arg125 on subunit IV); its replacement by a negatively charged residue would alter the charge network. Hydrogen bridges may rigidify the structure, preventing efficient assembly of the complex.
Our successful attempts to obtain Qi site mutants were based on the available 3-D structure. However, we could not rescue the ΔpetD deletion strain for photosynthetic growth upon transformation with the mutated plasmids. Paradoxically, after selection for resistance to antibiotics, the dL36A, dF40H/Y/L and dP41A mutants nevertheless turned out to be capable of phototrophic growth. The molecular basis of this paradoxical observation is not fully understood yet and was first hypothesised to reflect the complex organization of the Qi site. As evoked above, the full structure of this site is particularly complex, since it requires interactions between two subunits, cyt. and subunit IV, two cofactors, hemes and and a liposoluble electron carrier, plastoquinone. It may thus be very sensitive to changes in the proteic environment. However, those mutations altering the conformation of the Qi site were not detrimental for the assembly of cytochrome , since wild-type levels of the complex are found in the mutants recovered on spectinomycin-supplemented medium (data not shown). Mutants defective for cytochrome activity are light-sensitive, probably because the fruitless charge separation occurring in photosystems I and II leads to the formation of deleterious radical species. Radicals should be produced at high levels during the initial selection step of phototrophic transformants derived from deletion strains under high light. By contrast, their concentration should remain low when transformants are selected on spectinomycin-supplemented medium under low light from a wild-type recipient strain, which is capable to transfer electrons from PSII to PSI. This suggests an increased sensitivity to radical species (like the reactive oxygen species, ROS) of the mutants with altered Qi site, which would be specific of this site, since mutants with altered conformation of the Qo site do not present the same phenotype. This hypothesis may indicate a possible protective function for the ci heme, which is currently under investigation.
4 Materials and methods
4.1 Strains, media, and growth conditions
A wild-type strain derived from strain 137C [35] and the deletion strains ΔpetD and ΔpetB [24] were used as recipient strains in chloroplast transformation experiments. WT and mutant strains were grown on Tris-acetate-phosphate (TAP) medium (pH 7.2) at 25 °C, under dim light (5–6 μE m−2 s−1), unless otherwise indicated.
4.2 Site directed-mutagenesis
Plasmids pdWQ and pdWB, encompassing the regions coding for subunit IV and cytochrome respectively, have been described previously [24]. Mutations on cytochrome , Cys35Val, Gly37Val, Phe41Gly, Phe44Gly, Arg207Asp and on subunit IV Asp35Asn and Phe120Asp were introduced in plasmids pWB or pWQ, respectively, by site directed mutagenesis according to Kunkel [35].
Mutations Leu36Ala, Phe40Leu/His/Tyr and Pro41Ala were introduced in plasmid pWQ by PCR-mediated site-directed mutagenesis [36]. The aadA cassette conferring resistance to spectinomycin [25] was then inserted into the pWB or pWQ plasmids carrying the various mutations, respectively at the NsiI or EcoRV neutral restriction sites [2,26].
4.3 Chloroplast transformation in C. reinhardtii
Wild-type as well as ΔpetB and ΔpetD strains, respectively deleted for petB or petD genes, were transformed by tungsten particle bombardment according to Boynton et al. [37]. Transformants were selected either on minimum medium under dim light (60 μE m−2 s−1) or on TAP medium supplemented with spectinomycin (100 μg ml−1) under low light (10 μE m−2 s−1). They were submitted to several rounds of subcloning on selective medium until they reached homoplasmy, assessed by RFLP analysis of specific PCR amplification fragments.
4.4 Preparative and analytical techniques
For SDS-PAGE, membrane (or whole cells) proteins were resuspended in 100 mM dithiothreitol and 100 mM Na2CO3, and solubilised by 2% SDS at 100 °C for 1 min. Polypeptides were separated on a 12–18% polyacrylamide gel containing 8 M urea [38]. Immunoblotting was performed as described in Pierre et al. [15].
4.5 Fluorescence measurements
Fluorescence measurements were performed at room temperature on a home-built fluorimeter, using a light source at 590 nm. The fluorescence response was detected in the far-red region in the near IR region [26].
Acknowledgements
The authors are grateful to Yves Choquet and Jean-Luc Popot for their critical reading of the manuscript. Thanks are due to Daniel Picot for the fruitful discussion and the help in drawing Fig. 3. F.Z. whishes to thank Francis-André Wollman for his fundamental input in this study. A.L.L. was the recipient of a fellowship from the ‘Ministère de l'Enseignement supérieur et de la Recherche’, France.