1 Introduction
Wheat is one of the most important food crops. Wheat is grown under irrigated as well as rain-fed conditions worldwide. Under rain-fed conditions the developing grains of wheat are frequently exposed to mild to severe stress at grain development stage [1]. Grain of certain cultivars can often attract a price premium if it has a combination of good characters that make it suitable for bread making, rather than biscuit-making or livestock feed [2,3]. As we know, both protein content and protein quality have marked effects on bread-making quality [4]. In addition, wheat grain is rich in bioactive compounds which provide nutritional benefits to humans. Hence, changes in the elemental composition of wheat grain will affect the quality of human nutrition, especially for protein and microelement content, such as Fe and Zn, which are vital for human health [5]. Nutritional problems related to diets of cereal as wheat-based were observed throughout the country, and the consequences of malnutrition create immense economic and social costs [6,7].
Environmental factors, such as temperature, water and nitrogen nutrition also influence the ratio between soluble and insoluble proteins, affect bread-making quality, which is a function of the protein composition that is genetically controlled [8,9]. In addition, in studies focused on the environment or on the cultivar–environment interactions, a randomly chosen set of samples obtained in multisite experiments were often used, without a good knowledge of the potentially interactive factors causing these variations [10].
Wheat grain quality is crucial; in environments limiting the attainment of high quality, durum wheat is replaced by bread wheat of which the grain yield is higher than that of durum wheat [3,11]. Variations in flour quality in a hard-grained winter cultivar were related to changes in protein composition from drought stress during grain filling [12]. Certainly, timing is very important in modifying the effect of stress on yield formation [13]. Although the effect of drought stress on grain development and its yield in wheat is well documented [14,15], the studies on changes in nutritional value of the mature grains in strong-gluten wheat, in response to water stress at post-anthesis are, however, scanty. It is, therefore, imperative that a comprehensive analysis of the drought stress-induced changes in the nutritional value of wheat grains be carried out in cultivars categorized as strong gluten. This paper seeks to further clarify water stress environmental effects on grain quality and nutritional value (such as protein, mineral composition, lipid and starch content, etc.) of wheat using single different water conditions at post-anthesis stage.
2 Materials and methods
2.1 Plant materials and growth conditions
From 2007 to 2008, the experiment was conducted at Laiyang experimental station, Qingdao Agricultural University, China. Wheat seeds of strong-gluten variety (Triticum aestivum L., cv. Jimai 20) were collected from Crop Research Institute, SAAS (Shandong Academy of Agricultural Sciences). Plants were raised in 30 pots (36 cm diameter and 52 cm height, 18 seeds per pot) filled with local field topsoil in the rain-proof greenhouse. Organic compost (0.60 kg/pot), urea (62.46 g/pot) and (NH4)2HPO4 (90 g/pot) were applied prior to planting. Before sowing the soil was irrigated to 80% soil water capacity (SWC). The sowing was done on 8th October and the grains were harvested on 13th June. 20 days after sowing, nine plants left by thinning were maintained in each pot. The plants were irrigated daily and the quantity of water applied was always in excess, up to full drainage before flowering. For imposition of water deficit at post-anthesis stages, three soil water levels, 45% (W1), 65% (W2), and 85% (W3) SWC, were applied to each pot (10 pots per treatment) separately from flowering, and kept constant throughout the entire period of experiment by simply weighing each pot every 2 days, and adding the water lost accordingly. At the late growth phase, when total biomass of wheat accounted for more than 0.5% of the total pot weight (plastic pot + soil + soil water + biomass), that fraction of biomass was taken into account. At last, the grain samples were harvested at maturity in three replicates, which were stored in paper bags and kept in a freezer at −20 °C after being received in the laboratory until the related tests begun.
2.2 Protein isolation and quantification
Protein is analyzed as nitrogen on a Leco TruSpec carbon–nitrogen analyzer according to the method of Forage Analyses Procedures [16]. Protein content was calculated from nitrogen content multiplying by a constant of 5.7. The protein fractions from the grains were extracted using the protocol as described [17,18].
2.3 Mineral content
The ash content of the wheat grain was determined according to the AOAC method 923.03 [19]. Ground wheat samples were brought to room temperature prior to use. Crucibles were pre-dried in a furnace for 5 h at 525 °C. The crucible were cooled to room temperature in a desiccator. Approximately 2 g ground wheat grain samples were weighed into the dried crucibles and samples were ashed in a furnace for 5 h at 525 °C. Minerals were extracted from ash in hot 3 N HCl before analysis using Inductively Coupled Plasma Mass Spectrometer (ICP-MS).
2.4 Lipid content
Oil content was determined according to the AOAC method 960.39 [19]. Approximately 2 g ground wheat grain samples were weighed into a cellulose thimble. The thimbles were then placed in the Soxtec extraction unit (Tecator, Model 1043 Extraction Unit, Sweden), and 40 ml of petroleum ether was used to extract the oil from the samples. The extraction time was 30 min. The aluminum cup with the extracted oil was placed into vacuum oven (Fished Scientific, Isotemp® Oven, Fair Lawn, NJ) for 15 min to evaporate the excess moisture. The amount of extracted oil was determined gravimetrically.
2.5 Starch characteristics
Stiff dough was prepared by mixing (100 g) meal with distilled water (45–55 ml) in a laboratory pin mixer (National Mfg Co., Lincoln, NE) for 3 min at slow speed [20]. The dough ball was kept covered with moist cheese cloth at 30 °C for 1 h. Starch was washed from the dough ball, by kneading with hand under a stream of distilled water, over a sieve with mesh openings of 70 μm, fitted over a 2-liter vessel. The starch slurry was wet-sieved twice through the bolting cloth, to remove bran and endosperm cell-wall impurities. The material retained on the cloth was discarded. Starch slurry was then centrifuged at 2500g for 10 min. The upper pigmented layer (tailings) was carefully removed, using a small spatula, mixed with water (20 ml) and decanted from any starch which had settled after 30 min. The starch fraction plus starch from the decanting step were purified by resuspending in distilled water and centrifugation. Four such purification cycles were carried out to obtain pure starch. The starch was finally dried at 40 °C in a forced-air drier. The starch recovery was between 45% and 48%.
Amylase and amylopectin content of the starches isolated from different varieties was determined using the method of Williams, Kuzina, and Hlynka [21]. The quantity of amylase was determined from a standard curve plotted using blends of amylose and amylopectin.
2.6 Statistical analysis
Data were analyzed using SPSS 12.0 software for two-way ANOVA, t-test and standard deviation. The standard deviations were shown with numerals in tables and with error bars in figures, respectively. It was considered significant when (marked with (*)) and extremely significant when (marked with (**)).
3 Results and discussion
3.1 Protein characteristics
Protein content of grain is well known to affect the functional properties of processed wheat products. Hence it is an important parameter analyzed to evaluate quality attributes of wheat varieties. Protein amount and composition in grain are significantly influenced by environmental conditions such as temperature and mineral availability to plant. This study examined the protein contents of strong-gluten winter wheat grown at different soil water conditions during the later growth stage. Protein contents of the strong-gluten winter examined in this study varied from 14.8% to 16.7% (Table 1). Wheat grain samples from the experiments, 45% SWC (w1) and 85% SWC (w3) had the highest (16.7%) and lowest (14.8%) protein contents, respectively, which showed that over high SWC was not benefit to the accumulation of protein in wheat grain at post-anthesis stage, that is to say, proper water deficit (65% SWC) was benefit to protein formation of wheat grain at later growth stage. Whereas the opposite effect was observed in wheat grain yield [21], the obtaining of grain yield was higher under w2 (65% SWC) than w1 (45% SWC) and w3 (85% SWC).
Effects of soil water content at post-anthesis stage on protein and fractions in strong-gluten wheat grain.
Treatment | Protein (%) | Albumin (%) | Globulin (%) | Gliadin (%) | Glutenin (%) | Glutenin/gliadin ratio |
W1 | 2.5 | 2.2∗ | 1.49∗ | |||
W2 | 15.9∗ | 2.6∗ | 2.1 | 4.0∗ | 5.7 | 1.42 |
W3 | 14.8 | 2.4 | 2.0 | 3.6 | 5.1 | 1.42 |
In addition, the protein fractions (globulin, gliadin, glutenin, and glutenin/gliadin ratio) in grain samples were observed to be down-regulated in response to SWC increasing at post-anthesis except albumin (Table 1), which showed that the accumulation of protein components was sensitive to water stress at the later stage. The differential regulation of accumulation of proteins (especially gliadins and glutenins) in the mature grains of wheat by water conditions may have important implications in the processing qualities, and thus warrants further investigation. The results of protein fractions also revealed that good management of water in wheat field at post-anthesis stage was helpful both to improving protein content and composition in wheat grain and to yield formation.
3.2 Mineral composition
Ash content which refers to the mineral content of grain varies with variety, agronomic practices such as fertilization and irrigation. Mineral composition of wheat grain affects flour extractability. Wheat can be an important and inexpensive source of certain minerals for humans in the developing countries including China. The low concentrations of several essential micronutrients in modern crops contribute to the problem of micronutrient malnutrition, for instance, nearly half of the world's population is at risk of inadequate Zn intake [5]. So, the lower (nutritional value):(caloric value) of crops under agronomic practices including water supply will aggravate the micronutrient malnutrition problem in the world.
Five minerals (P, K, Ca, Mg, and Zn) were identified in ash samples from the experiments. Table 2 shows that the relationships between their contents and SWC. P, Ca, Mg, and Zn content in grain samples were linearly correlated with the increasing in SWC (ranged from 45% to 85%), their contents increased, except that K of all the samples was enhanced in response to SWC (ranged from 45% to 85%) increasing. The results indicated mineral contents in the grains of winter wheat were greatly affected by soil water conditions in the field, which showed that nutrient concentrations (P, Ca, Mg and Zn) in wheat grain were decreased by too high SWC. Whereas water deficit (low SWC such as w1 in the experiment) was benefit to mineral accumulation in wheat grain at post-anthesis stage, serious water shortage can lead to high decreasing in grain yield during the stage. As indicated above on SWC effects, proper water conditions (such as w2 in the experiment) at the later growth stage of wheat can be favorable to more mineral concentrations and higher yield in wheat grain than the other.
Effects of soil water content at post-anthesis stage on mineral composition (mg/g) in strong-gluten wheat grain.
Treatment | P (mg/g) | K (mg/g) | Ca (mg/g) | Mg (mg/g) | Zn (mg/kg) |
W1 | 3.4 ± 0.1 | 0.5 ± 0.03∗ | 1.3 ± 0.02∗ | ||
W2 | 3.1 ± 0.02∗ | 3.6 ± 0.09∗ | 0.4 ± 0.06 | 1.3 ± 0.04∗ | 52.3 ± 0.7∗ |
W3 | 2.9 ± 0.03 | 0.4 ± 0.02 | 1.2 ± 0.09 | 45.2 ± 1.5 |
3.3 Lipid contents
Although lipids are minor components of whole wheat grain they play important role in nutritional value, processing and storability of grain. Lipid content and composition of wheat are affected by variety and growth conditions [22]. In wheat grain lipids are present in free and bound forms [23].
Lipid contents of the wheat samples examined in the experiment are reported as weight percent of petroleum ether extracts (free lipids) based on whole grain weight. Fig. 1 indicated that SWC (ranged from 45% to 85%) can have a significant effect on oil contents (0.88–1.21%) of the grain samples at post-anthesis stage, that is to say, water deficit during grain filling can result in a decrease in lipid contents in wheat grains. The above results also indicated that wheat grains in this study had significantly lower protein and higher lipid contents from wheat exposed to high SWC at post-anthesis stage (Table 1 and Fig. 1). A similar trend was also observed in soy bean. Protein and lipid contents in soybean had a negative correlation [24]. A reduction in lipids content on exposure to water stress conditions has also been reported earlier in lupin seeds [25] and chickpea genotypes [26].
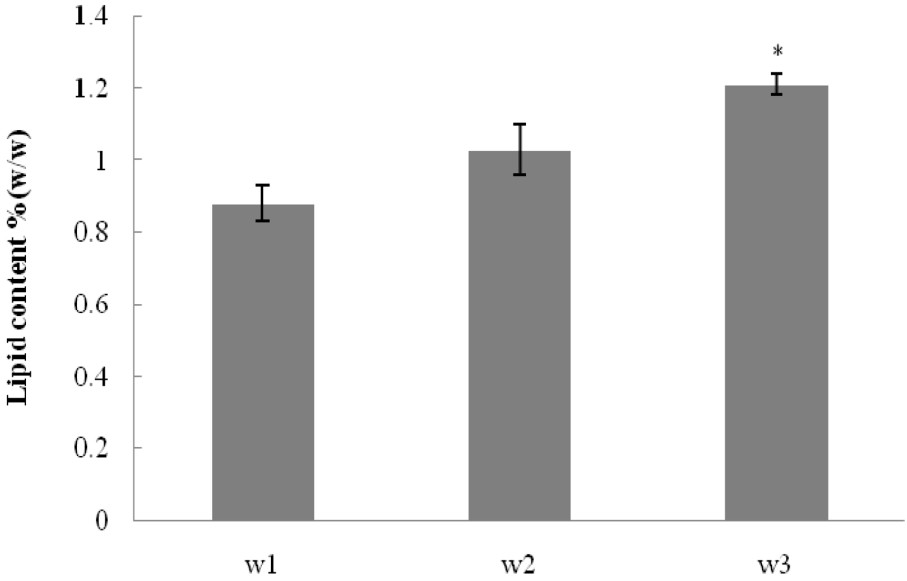
Effects of soil water content at post-anthesis stage on lipid contents (%(w/w)) in strong-gluten wheat grain.
3.4 Starch characteristics
Starch in the endosperm of wheat is the major form of carbon reserves and comprises 60% to 75% of the final dry weight of the grain [27]. Therefore, biosynthesis and accumulation of starch in grain is also a key process determining grain yield and quality in wheat. Our results in Table 3 clearly demonstrate that drought (w1) and more water supply can decrease starch contents. 65.5% of the final starch weight in wheat grains was accumulated during the later growth period of wheat imposed to w2 treatment (65% SWC), and only 61.1% (treatment w1) to 63.2% (treatment w3) for grains in the same period. As shown in Table 3, it also illustrates a mild water deficit (w2: 65% SWC) during grain filling would be beneficial to the grain filling by enhancing remobilization of stored reserves from the stems to grains for these cultivars. However, water stress imposed during the grain-filling period of wheat, especially at the early filling stage, usually results in a reduction in grain weight and leads to reduced grain yield [21,28,29]. In addition, water stress caused premature grain desiccation and resulted in a marked decline in grain sucrose and reduced grain weight [30].
Effects of soil water content at post-anthesis stage on starch and component content in strong-gluten wheat grain.
Treatment | Starch (%) | Amylose (%) | Amylopectin (%) | Amylopectin to amylose ratio |
W1 | 61.1 | 20.1 | 41.0 | 2.04 |
W2 | 65.5∗ | 21.2 | 44.3 | 2.09 |
W3 | 63.2 | 20.5 | 42.7 | 2.08 |
Starch molecules have one of two molecular structures: a linear structure, known as amylose; and a branched structure, known as amylopectin. The amylose: amylopectin ratio in wheat starch is known to affect its chemical characteristics. The results (Table 3) in this study was to determine the influence of soil water environment on amylose and amylopectin content, and amylose:amylopectin ratio in wheat grain at post-anthesis stage. Under terrible soil moisture deficit (treatment w1) at post-anthesis stage, amylopectin to amylose ratio, amylopectin and amylase content in the grains declined significantly, compared to treatment w2 and w3. Whereas good water environment (treatment w2) was benefit not only to biosynthesis and accumulation of starch but also to the regulation of starch composition (such as amylopectin to amylose ratio) during grain formation. So, we can say that if water availability is limited, drought stress can be most easily afforded during anthesis and grain development.
4 Conclusions
Wheat grain is an excellent staple food containing numerous nutritional and health beneficial compounds. This study focused on protein, mineral, lipid and starch contents in strong-gluten wheat grain exposed to different soil water content at post-anthesis stage. It is also important to examine variations in concentrations and compositions of the bioactive compounds and nutritions.
- (1) Protein contents of the samples varied from 14.8% to 16.7%. Effects of soil water environment on protein content and composition were significant.
- (2) Five minerals (P, K, Ca, Mg, and Zn) were identified in ash samples from the experiments, which were similar to the data published in the literature. In addition, P, K, Ca and Mg were the major minerals found in all the samples.
- (3) Water supply to soil (ranged from 45% to 85%) can have a significant effect on lipid contents (0.88%–1.21%) of the grain samples at post-anthesis stage, and a similar trend was also observed in other cereals.
- (4) 65.5% of the final starch weight in wheat grains was accumulated during post-anthesis period of wheat imposed to 65% SWC, and only 61.1% (under 45% SWC) to 63.2% (under 85% SWC) for grains in the same period.
It is evident from our results that as indicated above on SWC effects, proper water conditions at the later growth and development stage of wheat grain can be favorable to more mineral concentrations, better nutritions, higher quality and yield [21] in wheat grain. Meanwhile, the line of research will lead to elucidation of biological pathways that are involved in synthesis and accumulation of bioactive components in wheat and ultimately help to engineer good water management that can be used as agronomic practices to produce these valuable compounds in large quantities.
Acknowledgements
This research was supported by the Major State Basic Research Development Program of China (2009CB118602), the Science and Technology Planning Project of Qingdao (08-1-3-21-jch), the Open Foundation of State Key Laboratory of Soil Erosion and Dryland Farming on the Loess Plateau (10501-194), the National Natural Science Foundation of China (30871484) and China Postdoctoral Science Foundation (No. 20080430567).