1 Introduction
Physiology is a science with two identities. Its most well-known identity nowadays is also the most recent one: molecular physiology. What this type of physiology deals with is the function, in one particular cell type, of one protein or group of proteins whether they are transcription factors, receptors or ion channels. However, predating molecular physiology and relegated by it for the longest time to the attic of biology, there has been another physiology. This is the whole-organism physiology, the one described so well, but only described by three giants in this field: Claude Bernard, followed by Walter Cannon who formulated the concept of homeostasis, and L.J. Henderson who postulated that there is a mutual dependence between organs and functions (Bernard [1]; Henderson [2]; Cannon [3,4]). These two concepts, especially the last one were forged at a time when none of the molecular and genetic tools necessary to test their validity and to explain them were available. Yet, almost everything that Claude Bernard described has proven to be right. Likewise, what would physiology be without the notion of homeostasis? Below, we will come back on the far-reaching implication of the concept of mutual dependence. Ironically, it is the most sophisticated advance of molecular biology, the ability to inactivate one gene at a time in one cell type at a time and in an inducible manner that has rejuvenated whole-organism physiology. The premises on which whole-organism physiology is built, namely that all or most organs interact together, requires it to be tested in a living animal in which one molecule only is removed in only one cell type and after embryonic development has been completed. This can be achieved nowadays through the technological arsenal of mouse genetic tools.
At the same time that developmental biology was revolutionized through the ability of mouse genetics to study the function of a single gene in vivo, there has been, through the same means, spectacular advances in our knowledge of the genetic and molecular control of physiological functions in vertebrates. Among these many conceptual and experimental advances, one can cite the ever-growing in complexity, elucidation of the control of appetite (Friedman and Halas [5], Spiegelman and Flier [6]); the identification of a molecular clock (Albrecht et al. [7]; Yagita et al. [8]); of its components and of their respective functions; the identification of genes controlling longevity such as Sir1 and Insulin (Bell et al. [9]; Pillus and Rine [10]); and the realization that nuclear receptors regulate in a major way in heart function, energy metabolism and skin integrity (Kliewer et al. [11]; Huss et al. [12]; Schreiber et al. [13]; Li et al. [14]). In the field of skeleton biology, this mouse genetics-based approach has been very fertile, probably because skeleton is a late acquisition during evolution and therefore, it is more likely that gene functions are conserved between mice and humans (Karsenty and Wagner [15]). Among the many conceptual and biologic advances elicited in the field of the skeletal physiology by the use of mouse genetics, one can cite the identification of all the components the RankL-dependent pathway and the demonstration of their function in the control of osteoclast biology (Lacey et al. [16]; Kong et al. [17]), the identification of TSH and FSH (Abe et al. [18]; Sun et al. [19]), two pituitary hormones as regulators of bone mass; and the realization that bone formation and bone resorption are regulated by the sympathetic nervous system (Takeda et al. [20]; Elefteriou et al. [21]). All these discoveries that were made possible through mouse genetics have contributed to establish that previously unsuspected biological links exist between various organs, i.e. between fat and bone, between pituitary gland and bone, and between hypothalamus and bone. As a result, it becomes increasingly evident that if we want to make even more progress, skeletal physiology has to be studied in an integrative manner in an intact animal.
2 Common endocrine regulation of bone and energy metabolism
In the last fifteen years, my laboratory has become increasingly engaged in an intensive and systematic effort to define, and to understand molecularly, the role of bone as an endocrine regulator of energy metabolism, reproduction and now of other functions (Karsenty [22]). This investigation was initiated because of a spectrum of clinical observations linking bone modeling and remodeling to the control of energy metabolism and fertility. That gonadal failure favors appearance of osteoporosis while obesity seems to protect from osteoporosis and its deleterious consequences, was an argument to propose the following working hypothesis: bone mass, energy metabolism or at least some aspects of it, and reproduction may be regulated by the same hormones. As will be presented later, this hypothesis was not only based on an interpretation of the clinical literature, but also tested experimentally through genetic and molecular tools previously described, generating specific mutant mouse strains.
In our first attempt to test this hypothesis, we asked whether a hormone, such as leptin, made by adipocytes and regulating appetite, energy expenditure and reproduction influenced bone mass. Over the course of 10 years we have shown: (1) that leptin is a very powerful inhibitor of bone mass accrual in both mice and humans that acts by reducing bone formation and increasing bone resorption; (2) that this action occurs exclusively through a neuronal relay; (3) that this relay occurs via hypothalamic neurons; (4) that the sympathetic tone and CART are two mediators linking hypothalamic neurons to osteoblasts; and (5) that these two pathways, the sympathetic tone and CART act in osteoblasts to affect bone formation and resorption (Ducy et al. [23]; Takeda et al. [20]; Elefteriou et al. [21]; Fu et al. [24]; Shi et al. [25]).
Following the logic of most endocrine regulations, which always include a feedback loop, and based on the fact that activity of bone cells is controlled by so many hormones, we hypothesized that bone cells must, in turn, regulate some aspects of energy metabolism. In full support of this hypothesis, we have shown that an osteoblast-specific secreted molecule, osteocalcin, that in its undercarboxylated form, acts as a hormone to regulate energy metabolism through several synergistic functions: favoring pancreatic β-cell proliferation, increasing insulin expression and secretion, enhancing insulin sensitivity and promoting energy expenditure (Lee et al. [26]; Ferron et al. [27]; Fulzele et al. [28]) (Fig. 1). Remarkably, the metabolic activity of osteocalcin is modulated, indirectly, by leptin and possibly more extracellular cues (Hinoi et al. [29]; Yoshizawa et al. [30]). Hence, this body of work has verified the first aspect of our working hypothesis, namely that there is a reciprocal regulation of bone remodeling and energy metabolism. These results provided the first evidence that the skeleton is an endocrine organ favoring whole-body glucose homeostasis and energy expenditure.
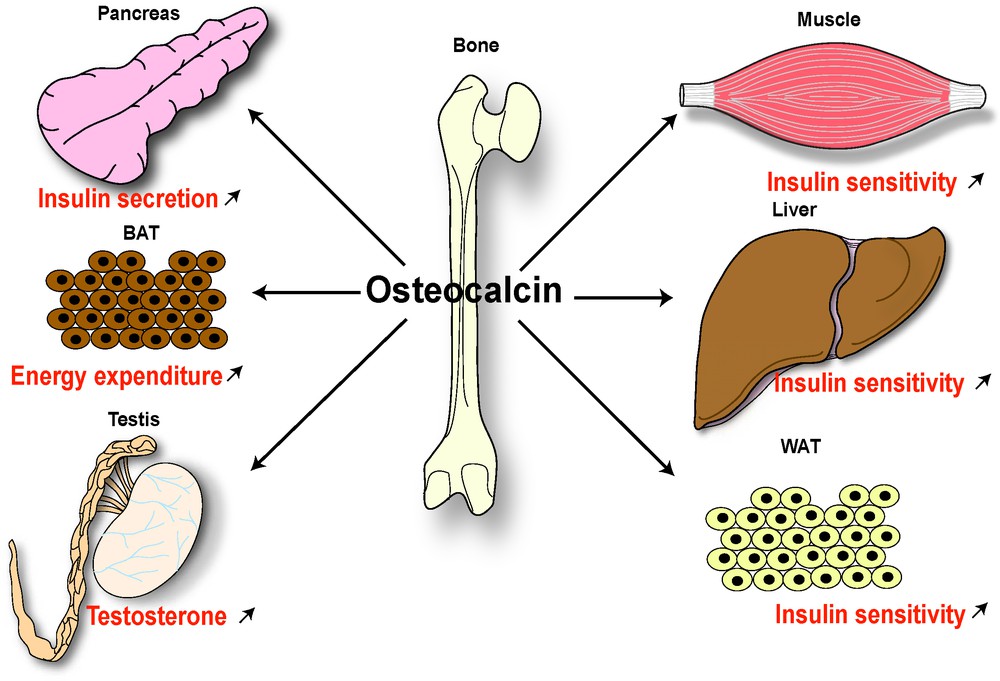
Endocrine regulation of energy metabolism and male fertility by bone. The endocrine regulation of energy metabolism and male reproduction by the bone is mediated by an osteoblast-specific secreted molecule, osteocalcin, that when undercarboxylated acts as a hormone favoring β-cell proliferation and insulin secretion in pancreas, insulin sensitivity in peripheral tissues (such as liver, muscle, white adipose tissue [WAT]), energy expenditure by brown adipose tissue (BAT) and testosterone production by Leydig cells in testis.
3 Osteocalcin-dependent regulation of male fertility
The discovery of hormonal functions of osteocalcin raised multiple questions with great biological and medical importance. Chief among them is to elucidate the signaling events triggered by this hormone in target cells. A prerequisite to address this question was the identification of a specific receptor for osteocalcin. A second question, with even broader implications, was to determine whether osteocalcin, like many hormones, has functions in addition to those exerted on energy metabolism. The well-known regulation of bone remodeling by gonads provides an ideal setting to address the aforementioned question. In light of this hypothesis, the proposal that bone influences fertility became of great conceptual importance.
That menopause favors bone loss is a well-established fact (Riggs et al. [31]; Khosla et al. [32]; Manolagas et al. [33]; Nakamura et al. [34]; Khosla [35]). What this medical observation means biologically is that gonads, mostly through sex steroids hormones, affect, in one way or another, the functions of bone cells, an aspect of bone physiology that will not be discussed here. What the regulation of bone mass accrual by gonads suggested is that bone in turn, in its endocrine capacity, may affect the reproductive functions in one or both genders. Verifying this hypothesis was of course of great conceptual importance as it would further enhance the emerging importance of bone as an endocrine organ.
Testing this hypothesis in vivo was admittedly greatly helped by a striking feature of mutant mice lacking osteocalcin. Indeed, while female Osteocalcin-deficient mice were normally fertile, the male mutant mice were rather poor breeders, whether their partners were WT or Osteocalcin-deficient. As it was the case for energy metabolism, the demonstration that this phenotype portrays a true biological function of osteocalcin was made more complete and convincing because of the availability of gain (Esp−/−) and loss-of-function (Osteocalcin−/−) mutations for Osteocalcin (Lee et al. [26]). Osteocalcin-deficient mice showed a decrease in testes, epididymitis and seminal vesicles weights whereas the weight of these organs was increased in Esp-deficient mice. The spermogram of male osteocalcin-deficient showed a 50% decrease in sperm count, whereas the one of male Esp-deficient mice showed a 30% increase in this parameter, and Leydig cell maturation appeared to be halted in absence of Osteocalcin (Oury et al. [36]). These features suggested that osteocalcin might favor testosterone synthesis. Again, this was verified by a simple but always powerful co-culture assay (Oury et al. [36]). The supernatants of WT osteoblasts in culture increased testosterone production by Leydig cells of the testes, a feat no other mesenchymal cells could achieve to the same extent (Oury et al. [36]). Accordingly, circulating testosterone levels are low in Osteocalcin−/− and high in Esp−/− mice (Oury et al. [36]).
To formally establish that osteocalcin is regulating testosterone production as a bone-derived hormone, and not as a testis-secreted growth factor, mice lacking Osteocalcin only in osteoblasts were generated. Male Osteocalcinosb−/− mice had the same testosterone production defect as the classical Osteocalcin−/− mice, while deletion of Osteocalcin in Leydig cells did not affect male fertility (Oury et al. [36]). Taken together, these experiments established that osteocalcin is a bone-derived hormone favoring fertility in male mice by promoting Leydig cell maturation and testosterone production (Fig. 1). In other words, it verified that for at least one gender, there is an endocrine regulator of reproduction by the skeleton. It also reveals a novel notion in endocrinology of reproduction, that there are major differences between males and females in the regulation of this function.
In the molecular era, the identification of a novel hormone immediately begs the question of its mechanism of action. A prerequisite to answering this question is to characterize a receptor to which this hormone would bind specifically to its target cells. In the case of osteocalcin, this was achieved through a two-step strategy taking advantage of the fact that osteocalcin regulates fertility in males but not in females (Oury et al. [36]).
In the first step, we asked was what is the signal transduction pathway affected by osteocalcin in two target cells, the β-cell of the pancreas and the Leydig cell of the testis (Lee et al. [26]; Oury et al. [36]; Pi et al. [37]). This approach identified the production of cAMP as the only intracellular signaling event reproducibly triggered by osteocalcin in these two cell types. We interpreted this result as suggesting that the, or an osteocalcin receptor, is probably a G protein coupled receptor (GPCR) linked to adenylate cyclase. Therefore, in the second step of this experimental strategy, taking advantage of the dichotomy of function of osteocalcin between males and females, we asked whether there were orphan GPCRs expressed at a higher level (5-fold higher) in testes than in ovaries. Out of more than a hundred orphan GPCRs submitted to this test, twenty-two of them were more expressed in testes than in ovaries and only four were expressed predominantly or only in Leydig cells (Oury et al. [36]). One of these four orphan GPCRs, GPRC6A, was a particularly good candidate to be an osteocalcin receptor, since its inactivation in mice results in metabolic and reproduction phenotypes similar to those seen in Osteocalcin−/− mice (Pi et al. [38]). Furthermore, and although it was never tested through any binding assays, it had been proposed that GPRc6A was a calcium serving receptor working better in the presence of osteocalcin (Oury et al. [36]). Although the aforementioned result could not be reproduced, several criteria formally identified Gprc6A as an osteocalcin receptor present in Leydig cells (Pi et al. [38]; Oury et al. [36]). First, there is direct binding of osteocalcin to WT but not to Gprc6a-deficient Leydig cells; second, osteocalcin increased cAMP production in WT but not in GPRc6a-deficient Leydig cells; third, and more to the point, a Leydig cell-specific deletion of GPRc6a revealed a reproduction phenotype caused by low testosterone production similar, if not identical, to the one seen in the case of osteocalcin inactivation; fourth, in an even more convincing experiment compound heterozygous mice lacking one copy of Osteocalcin and one copy of Gprc6a had a reproduction phenotype identical in all aspects to the one seen in Osteocalcin−/− or Gprc6a−/− mice. The identification of GPRc6A as an osteocalcin receptor led subsequently to the realization that CREB is a transcriptional effector of osteocalcin regulation of testosterone biosynthesis, by favoring the expression of key enzymes of this biosynthetic pathway in Leydig cells [36] (Fig. 1). The identification of Gprc6a now allows us to address many more questions, chief among them is to identify others functions of osteocalcin. It allows us also to perform a more sophisticated dissection of the osteocalcin molecular mode of action in known, and yet to be identified, target cells.
4 Conclusion
This work demonstrates how powerful the concept of functional dependence in physiology is, and how intimately connected are all the organs and genetic direction in model organisms, provided they are vertebrates and provides an ideal tool to link multiple physiologies, and therefore, multiple medical disciplines. As of today and for the foreseeable future, mouse genetics is the most powerful tool to map out and study all the inter-organ connections that exists in vertebrates.
The functions of the skeleton are not all known yet but the ones we know indicate that skeleton physiology affects many more organs and functions than skeleton itself, as it already affects glucose homeostasis, energy expenditure and fertility. Lastly, and this is a major outcome of this work, if the skeleton affects two major functions so significantly and possibly others, then it is likely that vertebrate physiology is best studied in animal models containing a bony skeleton.
Disclosure of interest
The authors declare that they have no conflicts of interest concerning this article.