1 Introduction
Plant development and performance is strongly influenced by environment. Light is one of the most important factors affecting plants because it is involving in the regulation of seed germination, establishment of seedlings, determination of growth habit, and the transition to flowering [1]. Plants have a collection of photoreceptors perceiving information (light quality and quantity, and duration) about their light environment [2,3]. Red (R) and far-red (FR) light are important environmental signals in the regulation of plant development, with major roles in seedling de-etiolation, neighbor detection, and photoperiodism [4,5]. Plant R and FR photoreceptors, the phytochromes, are soluble chromoproteins consisting of a 120–130 kDa apoprotein and a linear tetrapyrrole chromophore. A small gene family phy, encoding the phytochrome apoproteins (chromoproteins), functions in the absorption of R and FR light and the transduction of intracellular signals during light-regulated plant development [6].
Plants contain multiple, distinct phytochrome species that are the products of a divergent gene family [7]. Physiological and biochemical experiments in earlier studies indicated that plants contain two different forms of phytochromes [8–10]. The first form is frequently referred to as type-I (or light labile) phytochrome, predominating in etiolated tissue, while the second form is referred to as type-II (or light-stable) phytochrome, prevalent in light-grown tissue [11–13].
The phy gene family was first identified in Arabidopsis and subsequently in many other plant species [1,7,14–16]. In Arabidopsis, there are five phytochrome genes, phyABCDE [17]. The product of the phyA gene, phyA, is light labile and predominates in etiolated seedlings where it accumulates to relatively high levels. phyB and phyC proteins are more light-stable, with phyB are common in light-grown tissues [18]. Arabidopsis may not be representative of flowering plants because additional phy loci related to phyA and phyB evolved independently several times in eudicots. Additionally, monocots may lack orthologs of Arabidopsis phyD and phyE [19]. Etiolated seedlings of phyB mutants are deficient in several responses to red light [20,21]. Light-grown seedlings of phyB mutants have an elongated, early flowering phenotype of the shade-avoidance syndrome of wild-type seedlings grown under low R/FR. The phyB mutant seedlings display attenuated responses to the low R/FR or to the end of day (EOD) far-red light, leading to the proposal that phyB may play a key role in the shade-avoidance response [22,23]. In addition, Hirschfeld et al. [13] indicated that the level of phyC apoprotein is strongly reduced in Arabidopsis phyB mutants. This observation suggests that phenotypes associated with phyB mutant genes result from the attenuation of phyC signaling. Some shade-avoidance responses of the phyB mutant to the low R/FR or to EOD far-red light are severely attenuated [22], although other responses, such as reduction in leaf area and acceleration of flowering, are clearly retained [24,25]. Devlin et al. [26] demonstrated that phyA–phyB double mutants respond to EOD far-red light by the acceleration of flowering and by the promotion of elongated internodes between rosette leaves. These responses are reversible by subsequent treatment with red light, indicating that one or more of phyC, phyD, or phyE control flowering time and internode elongation [26,27]. Unexpectedly, Hu et al. [28] revealed both light-dependent and -independent roles for phytochromes to regulate the Arabidopsis circadian clock, indicating dual roles for phytochromes to arrest and/or promote the progression of plant development in response to the prevailing light environment. Phytochrome was also shown to function in circadian clock adjustment to plant iron status through a new retrograde pathway that involves a plastid-encoded protein [29].
Rhazya stricta Decne is a robust plant with erect stems and upright thick and smooth leaves placed close together on the stem [30]. It is common in the Arabian Peninsula and the Indian subcontinent. Leaves are used to make a tonic with a peculiar bitter taste. Rhazya stricta is used traditionally in Asia for the treatment of different types of diseases, such as skin diseases, stomach diseases, and hypertension [31]. The leaves, flowers and fruits are also used in joint infections and for cancer [32]. Phytochemical analyses have identified more than 100 alkaloids [33] with several pharmacological properties. There is considerable evidence that light can affect the production of alkaloids. For example, Catharanthus roseus L. is affected by light in the production of the dimeric indole alkaloids, vinblastine and vincristine. The biosynthesis and accumulation of vindoline in the intact plant is controlled by tissue-specific, development regulated, and light-dependent factors [34]. Several lines of evidence showed that phytochromes are involved in the light-regulated biosynthesis of these dimeric indole alkaloids. Also, Höft et al. [35] demonstrated that the alkaloid content in the leaves of Tabernaemontana pachysiphon Stapf. (Apocynaceae) is influenced by light intensity.
In this study, phytochrome-like genes of phyA, phyC and phyE were characterized from de novo assembled genome contigs from next generation sequencing in the wild plant species Rhazya stricta. Bioinformatic analyses of these data confirmed the recovery of three full-length phytochrome-like genes.
2 Materials and methods
2.1 Isolation of nuclear DNA
Extraction of total DNA was performed using the modified procedure of Gawel and Jarret [36]. Three samples of discs collected from the upper leaves of R. stricta were frozen in liquid nitrogen (approximately 500 mg of tissue each). To remove RNA contaminants, RNase A (10 mg/mL, Sigma, USA) was added to the DNA samples and incubated at 37 °C for 30 min. Estimation of the DNA concentration in different samples was done by measuring optical density at 260 nm according to the equation:
Purified DNA samples were sent to Beijing Genomics Institute (BGI), Shenzhen, China for sequencing.
2.2 Sequence filtering and bioinformatic analysis
The raw sequence data were obtained using the Illumina python pipeline v. 1.3. For obtained libraries, only high quality reads (quality > 20) were retained. Then, de novo assembly of the short single-end read dataset was performed using assembler Velvet, which has been developed for the assembly of short reads using the de Bruijn graph algorithm [37].
2.3 Basic local alignment search tool (BLAST)
BLAST finds regions of local similarity between sequences. The program compares nucleotide or protein sequences to sequence databases, and calculates the statistical significance of matches based on pair-wise alignment method. It can be used to infer functional and evolutionary relationships between sequences as well as help identify members of gene families (http://www.ncbi.nlm.nih.gov/BLAST).
2.4 Eukaryotic GeneMark.hmm program
GeneMark.hmm E-3.9 (http://exon.gatech.edu/eukhmm.cgi, A. Lukashin and M. Borodovsky, unpublished) was used for gene finding and annotation of R. stricta phytochrome-like genes. The statistical model of genomic sequence organization employed in the GeneMark.hmm algorithm is a HMM with duration [38] or a hidden semi-Markov model (HSMM).
2.5 Sequence alignment and estimation of phylogenetic relationships
MUSCLE [39] was used for multiple-sequence alignment. GenBank accession numbers for phytochrome protein sequences data aligned and analyzed in this study are displayed in Table 1. Maximum likelihood was used to build a tree where the evolutionary rates are free to differ in different lineages. ML trees were constructed using amino acid sequences and the Jones–Taylor–Thornton (JTT) substitution model. To evaluate the reliability of the inferred trees, bootstrap analysis using 1000 replicates was carried out. All the phylogenetic analyses were performed using MEGA 5.2 [40].
Accession number, description of the genes and organism (Latin name), for phytochrome genes used in this study.
Accession No. | Description | Latin Name |
XM_637404 | Dictyostelium discoideum AX4 prolyl 4-hydroxylase (phyA) mRNA, complete cds | Dictyostelium discoideum AX4 |
XM_004362186 | Dictyostelium fasciculatum prolyl 4-hydroxylase (phyA) mRNA, complete cds | Dictyostelium fasciculatum |
AF547224 | Synthetic construct phytase (phyA) mRNA, complete cds | synthetic construct |
EU786166 | Aspergillus japonicus strain BCC18313 PhyA (phyA) mRNA, complete cds | Aspergillus japonicas |
EU786167 | Aspergillus niger strain BCC18081 PhyA (phyA) mRNA, complete cds | Aspergillus niger |
XM_002561048 | Penicillium chrysogenum Wisconsin 54-1255 phytase phyA from patent WO2003038111-A2-Penicillium chrysogenum (phyA) mRNA, complete cds | Penicillium chrysogenum Wisconsin 54-1255 |
AJ310697 | Agrocybe pediades mRNA for Phytase (phyA gene) | Agrocybe pediades |
AJ310700 | Trametes pubescens mRNA for Phytase (phyA gene) | Trametes pubescens |
AB042805 | Aspergillus oryzae phyA mRNA for phytase, complete cds | Aspergillus oryzae |
AJ310696 | Peniophora lycii mRNA for Phytase (phyA gene) | Peniophora lycii |
GU120223 | Aspergillus sp. A25 phytase (phyA) mRNA, complete cds | Aspergillus sp. A25 |
AJ543399 | Trichoderma harzianum phyA gene for phytase | Trichoderma harzianum |
JF412664 | Amphicarpaea edgeworthii phytochrome B (PhyB) mRNA, complete cds | Amphicarpaea edgeworthii |
JQ771614 | Arabidopsis thaliana ecotype Landsberg erecta (Ler-0) phytochrome C (PhyC) mRNA, complete cds | Arabidopsis thaliana |
DQ208423 | Solanum tuberosum phytochrome A (phyA) mRNA, complete cds | Solanum tuberosum |
AB743571 | Fragaria x ananassa phyA mRNA for phytochrome A, complete cds | Fragaria x ananassa |
XM_002271635 | PREDICTED: Vitis vinifera phytochrome E (PhyE), mRNA | Vitis vinifera |
GU994130 | Amblyopyrum muticum cultivar Ae45 phytochrome A (PhyA) mRNA, complete cds | Amblyopyrum muticum |
GU994114 | Triticum monococcum cultivar BO1 phytochrome A (PhyA) mRNA, complete cds | Triticum monococcum |
AB743572 | Fragaria x ananassa phyB mRNA for phytochrome B, complete cds | Fragaria x ananassa |
XM_003555718 | Glycine max phytochrome type A-like (LOC100790763), mRNA | Glycine max |
EU428746 | Glycine max phytochrome A-1 (phyA) mRNA, complete cds | Glycine max |
EU428748 | Glycine max phytochrome A-3 (phyA) mRNA, complete cd. | Glycine max |
EU428747 | Glycine max phytochrome A-2 (phyA) mRNA, complete cds | Glycine max |
NM_117721 | Arabidopsis thaliana phytochrome D (PhyD) mRNA, complete cds | Arabidopsis thaliana |
AY348568 | Orobanche minor phytochrome A (phyA) mRNA, complete cds | Orobanche minor |
AY348569 | Monotropastrum globosum phytochrome A (phyA) mRNA, complete cds | Monotropastrum globosum |
X75412 | P. crispum phyA mRNA for phytochrome A | Petroselinum crispum |
AF544028 AY078504 | Stellaria longipes ecotype prairie phytochrome B (PhyB) mRNA, complete cds | Stellaria longipes |
NM_001251357 | Glycine max phytochrome A (PhyA), mRNA | Glycine max |
L34844 | Soybean phytochrome A (phyA) mRNA, complete cds | Glycine max |
NM_117923 | Arabidopsis thaliana phytochrome E (PHYE) mRNA, complete cds | Arabidopsis thaliana |
U39787 | Ipomoea nil phytochrome E (PhyE) mRNA, complete cds | Ipomoea nil |
NM_122975 | Arabidopsis thaliana phytochrome C (PhyC) mRNA, complete cds | Arabidopsis thaliana |
EU428751 | Glycine max phytochrome B-3 (phyB) mRNA, complete cds | Glycine max |
XM_003533109 | Glycine max phytochrome B-like (LOC100799831), mRNA | Glycine max |
NM_001123784 | Arabidopsis thaliana phytochrome A (PhyA) mRNA, complete cds | Arabidopsis thaliana |
U56731 | Sorghum bicolor phytochrome C (PhyC) mRNA, complete cds | Sorghum bicolor |
XM_002991073 | Selaginella moellendorffii hypothetical protein (PhyB), mRNA | Selaginella moellendorffii |
AF544029 | Stellaria longipes ecotype prairie phytochrome C (PhyC) mRNA, complete cds | Stellaria longipes |
U56729 | Sorghum bicolor phytochrome A (PhyA) mRNA, complete cds | Sorghum bicolor |
XM_002278574 | PREDICTED: Vitis vinifera phytochrome A (PhyA), mRNA | Vitis vinifera |
NM_100828 | Arabidopsis thaliana phytochrome A (PhyA) mRNA, complete cds | Arabidopsis thaliana |
XM_002318877 | Populus trichocarpa phytochrome (phya), mRNA | Populus trichocarpa |
AJ001914 | Lycopersicon esculentum mRNA for phytochrome A, type 1 | Solanum lycopersicum |
AB109891 | Oryza sativa Japonica Group PHYA mRNA for phytochrome A, complete cds | Oryza sativa Japonica Group |
AJ001318 | Populus tremula x Populus tremuloides mRNA for phytochrome A | Populus tremula x Populus tremuloides |
AB036762 | Armoracia rusticana phyA mRNA for phytochrome A, complete cds | Armoracia rusticana |
AJ001915 | Lycopersicon esculentum mRNA for phytochrome A, type 2 | Solanum lycopersicum |
NM_001247561 | Solanum lycopersicum alternative transcript type 3 (phyA), mRNA | Solanum lycopersicum |
AJ001916 | Lycopersicon esculentum mRNA for phytochrome A type 3 | Solanum lycopersicum |
AY348567 | Cuscuta pentagona phytochrome A (phyA) mRNA, complete cds | Cuscuta pentagona |
EU428752 | Glycine max phytochrome B-4 (phyB) mRNA, complete cds | Glycine max |
AB018442 | Oryza sativa Japonica Group mRNA for phytochrome C, complete cds | Oryza sativa Japonica Group |
NM_127435 | Arabidopsis thaliana phytochrome B (PhyB) mRNA, complete cds | Arabidopsis thaliana |
AY345120 | Cyrtosia septentrionalis phytochrome A (phyA) mRNA, complete cds | Cyrtosia septentrionalis |
AY888046 | Triticum aestivum putative phytochrome B (PhyB) mRNA, complete cds | Triticum aestivum |
AB264087 | Lotus japonicus phyb mRNA for phytochrome b, complete cds | Lotus japonicas |
3 Results
3.1 Next generation sequencing and BLAST searches for phytochrome-like gene family
A total of 73,841,902 single-end short DNA sequence reads was generated for R. stricta using the HiSeq 2000 Illumina platform (Illumina, San Diego, CA). The raw sequencing reads have been deposited at EMBL (accession number PRJEB4739). De novo assembly using Velvet generated 714,083 contigs and the 45,641 contigs greater than 1000 bp were used in constructing a local database. Five sequences belonging to Arabidopsis thaliana phytochrome gene family (i.e. AtphyABCDE, accession nos. NM_100828.3, NM_127345.3, NM_122975.2, NM_117721.1 and NM_117923.7, respectively) were obtained from GenBank and used for searching the local database to identify contigs with phytochrome-like sequences using BLAST. The BLAST searches resulted in the identification of three phytochrome-like contigs in R. stricta. The first contig (number 152,341) was 4955 bp long and had an average depth of coverage of 15.32 and corresponds to AtphyA-like sequence. The second contig (number 6,028) was 7216 bp long with an average coverage of 13.25 and represents an AtphyC-like. The third contig (number 66,070) was 7000 bp long with an average coverage of 12.61 and corresponds to an AtphyE-like sequence (Table 2).
Description of the three detected Atphy-like sequences in Rhazya stricta genome contigs greater than 1000 bp.
Subject | Query | Score | Identities | E-value |
PhyA (NM_100828.3) | Contig no. 52,341 | 1266 | 1593/2037 (78%) | 0.0 |
phyC (NM_122975.2) | Contig no. 6,028 | 893 | 1243/1737 (72%) | 0.0 |
phyE (NM_117923.7) | Contig no. 6,070 | 850 | 1186/1654 (72%) | 0.0 |
3.2 Annotation of the three phytochrome gene sequences
GeneMark.hmm was used to identify exon-, intron- and protein-like structures in three phytochrome-like sequences of the R. stricta contigs. Each contig consisted of one phytochrome-like gene comprised of three exons and two introns (Fig. 1, Table 3). Contigs 152,341, 6,028 and 66,070 were submitted to GenBank (accession numbers: phyA–HG380749; phyC–HG380750; phyE–HG380751). BLASTn and BLASTp of the GenBank nucleotide and protein databases were performed using the predicted mRNA and protein sequence of each contig to search for homologous sequences.
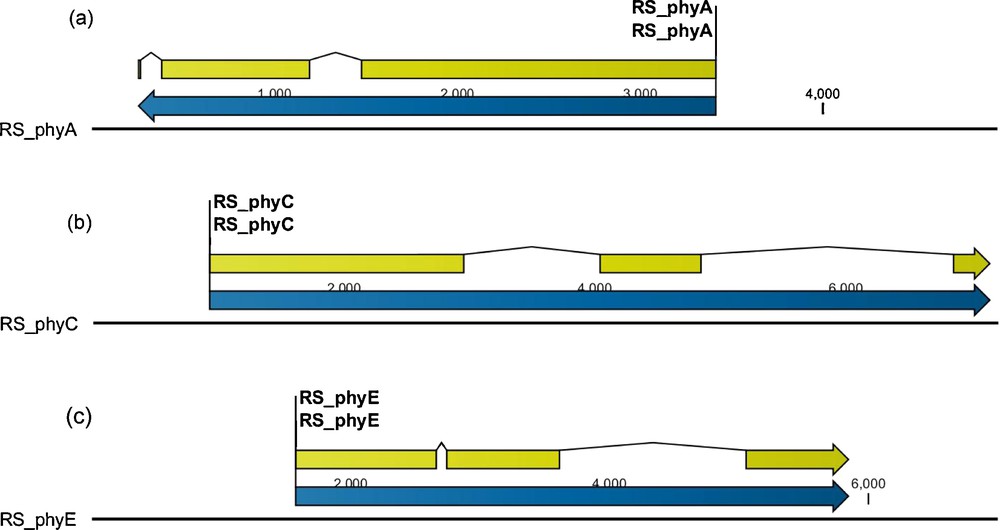
The relative position for the three phytochrome-like genes (blue), i.e. RsphyA-like (a) RsphyC-like (b) and RsphyE-like (c), and exons (yellow) within the three contigs. Color online.
Annotation results of the three contigs with Rsphy-like sequences showing number, type, range and length of exons (bp).
Gene | Contig No. | Exon No. | Strand | Exon Type | Exon range (bp) | Exon length (bp) | |
Start | End | ||||||
phyA | 15,2341 | 3 | – | Terminal | 252 | 270 | 19 |
phyA | 15,2341 | 2 | – | Internal | 379 | 1195 | 817 |
phyA | 15,2341 | 1 | – | Initial | 1372 | 3418 | 2047 |
phyC | 6,028 | 1 | + | Initial | 923 | 2960 | 2038 |
phyC | 6,028 | 2 | + | Internal | 4035 | 4851 | 817 |
phyC | 6,028 | 3 | + | Terminal | 6854 | 7154 | 301 |
phyE | 66,070 | 1 | + | Initial | 1570 | 2667 | 1098 |
phyE | 66,070 | 2 | + | Internal | 2737 | 3619 | 883 |
phyE | 66,070 | 3 | + | Terminal | 5050 | 5852 | 803 |
Rhazya stricta phyA-like (or RsphyA-like) mRNA sequence (contig 152,341) had the highest homology to the phyA coding sequence of Wrightia coccinea with query coverage equal to 49% and an E-value of 0.0. Protein sequence searches indicated that RsphyA-like sequence is most similar to phyA of Solanum tuberosum with query coverage equal to 96% and an E-value of 0.0. Both results confirmed that contig 152,341 likely contains a full-length copy of the RsphyA-like gene (Table 3 and Fig. 1a). Searches of mRNA sequence predicted from the second contig (6,028) identified a Rhazya stricta phyC-like (or RsphyC-like) sequence that had the highest sequence identity to the phyC coding sequence of Vitis vinifera with query coverage equal to 98% and an E-value of 0.0. The protein sequence query identified the highest identity to phyC of Vitis riparia with query coverage equal to 99% and an E-value of 0.0. These results confirmed that contig 6,028 likely contains a full-length RsphyC-like gene (Table 3 and Fig. 1b). Finally, using the mRNA sequence predicted from the third contig (66,070), R. stricta phyE-like (or RsphyE-like) sequence was found to have high sequence identity to the phyE coding sequence of Ipomoea nil with query coverage equal to 96% and an E-value of 0.0. Protein sequence queries indicated that this contig had the highest identity with phyE of Ipomoea nil with query coverage equal to 97% and an E-value of 0.0. Both results confirmed that contig 66,070 likely contains a full-length copy of the RsphyE-like gene (Table 3 and Fig. 1c).
Protein sequences of the three identified R. stricta phy-like genes were analyzed against the pfam database (http://pfam.sanger.ac.uk/) to detect the protein family and conserved domains. The analysis revealed the presence of a phytochrome protein family (accession number PF00360). The domains summary of the predicted proteins is shown in Tables 4–6 and Fig. 2.
Pfam search results show relative position, bit score and E-value for the identified protein family and domains within R. stricta phyA-like identified protein.
Family | Description | Entry type | Clan | Envelope | Alignment | HMM | HMM length | Bit score | E-value | ||||
Start | End | Start | End | From | To | ||||||||
N-terminal domain | NTE | N-terminal extension | Region | N/A | 1 | 65 | 1 | 65 | N/A | N/A | 65 | 60.8 | 4.00E−09 |
PAS_2 | PAS fold | Domain | CL0183 | 66 | 182 | 66 | 182 | 2 | 110 | 110 | 135.8 | 7.30E−40 | |
GAF | GAF domain | Domain | CL0161 | 215 | 398 | 215 | 398 | 1 | 154 | 154 | 142.4 | 1.20E−41 | |
PHY | Phytochrome region | Family | CL0161 | 409 | 588 | 409 | 586 | 1 | 181 | 183 | 234.8 | 3.60E−70 | |
C-terminal domain | PAS | PAS fold | Domain | CL0183 | 616 | 731 | 617 | 731 | 2 | 113 | 113 | 78.8 | 2.50E−22 |
PAS | PAS fold | Domain | CL0183 | 746 | 871 | 747 | 869 | 2 | 111 | 113 | 82.0 | 2.40E−23 | |
HisK | Histidine kinase-related (phospho-acceptor) | Domain | CL0025 | 891 | 953 | 894 | 948 | 4 | 61 | 68 | 28.5 | 1.10E−06 |
Pfam search results shows relative position, bit score and E-value for the identified protein family and domains within R. stricta phyC-like identified protein.
Family | Description | Entry type | Clan | Envelope | Alignment | HMM | HMM length | Bit score | E-value | ||||
Start | End | Start | End | From | To | ||||||||
N-terminal domain | NTE | N-terminal extension | Region | N/A | 1 | 67 | 1 | 67 | N/A | N/A | 67 | 137 | 1.00E−35 |
PAS_2 | PAS fold | Domain | CL0183 | 68 | 184 | 68 | 184 | 1 | 110 | 110 | 145 | 1.00E−42 | |
GAF | GAF domain | Domain | CL0161 | 218 | 396 | 221 | 395 | 5 | 153 | 154 | 133.7 | 5.70E−39 | |
PHY | Phytochrome region | Family | CL0161 | 407 | 586 | 407 | 584 | 1 | 181 | 183 | 234.9 | 3.30E−70 | |
C-terminal domain | PAS | PAS fold | Domain | CL0183 | 613 | 728 | 614 | 728 | 2 | 113 | 113 | 67.2 | 9.80E−19 |
PAS | PAS fold | Domain | CL0183 | 743 | 868 | 745 | 866 | 3 | 111 | 113 | 58.5 | 4.70E−16 | |
HisK | Histidine kinase-related (phospho-acceptor) | Domain | CL0025 | 892 | 952 | 893 | 950 | 6 | 66 | 68 | 27.7 | 1.90E−06 |
Pfam search results shows relative position, bit score and E-value for the identified protein family and domains within R. stricta phyE-like identified protein.
Family | Description | Entry type | Clan | Envelope | Alignment | HMM | HMM length | Bit score | E-value | ||||
Start | End | Start | End | From | To | ||||||||
N-terminal domain | NTE | N-terminal extension | Region | N/A | 1 | 67 | 1 | 67 | N/A | N/A | 67 | 138 | 3.00E−36 |
PAS_2 | PAS fold | Domain | CL0183 | 83 | 196 | 83 | 196 | 1 | 110 | 110 | 136.7 | 3.70E−40 | |
GAF | GAF domain | Domain | CL0161 | 229 | 371 | 231 | 368 | 3 | 126 | 154 | 104.8 | 4.30E−30 | |
PHY | Phytochrome region | Family | CL0161 | 387 | 566 | 387 | 563 | 1 | 180 | 183 | 237.3 | 6.10E−71 | |
C-terminal domain | PAS | PAS fold | Domain | CL0183 | 593 | 709 | 594 | 709 | 2 | 113 | 113 | 85.3 | 2.30E−24 |
PAS | PAS fold | Domain | CL0183 | 724 | 846 | 725 | 844 | 2 | 111 | 113 | 57 | 1.40E−15 | |
HisK | Histidine kinase-related (phospho-acceptor) | Domain | CL0025 | 865 | 924 | 869 | 921 | 5 | 59 | 68 | 27.9 | 1.70E−06 |
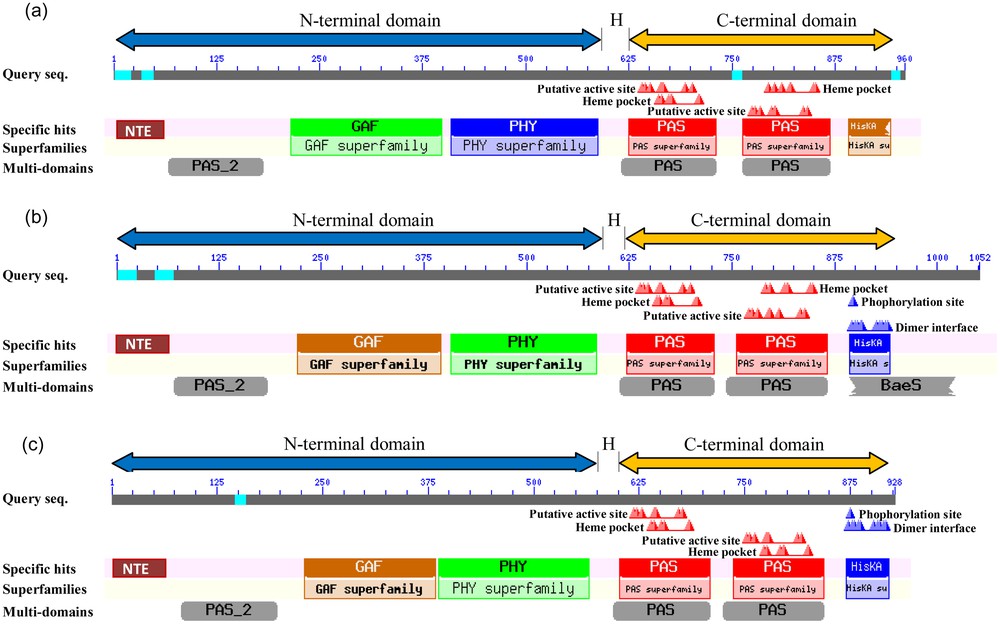
Domain structure of the three phytochrome-like proteins of R. stricta displayed as a Pfam diagram. The figure shows relative position for the identified protein family and N-terminal and C-terminal domains within the three identified proteins, i.e. RsphyA-like (a) RsphyC-like (b) and RsphyE-like (c). Domains are connected by a flexible hinge region (H). Color online.
3.3 Multi-sequence alignment (MSA) and phylogenetic analysis
The best BLASTp search hits were used to perform multi-sequence alignment. This resulted in 30 sequences from 23 different species. Alignment of the 30 sequences was obtained by gap open penalty of 10 and gap extension penalty of one. Alignment of R. stricta phytochromes-like proteins and the 30 amino acid sequences from the BLASTp search showed high sequence identity (Table 7). Phylogenetic relationships among these sequences showed that each phytochrome-like gene of the R. stricta grouped with the same type of subunit in the other plant species (Fig. 3).
Information for 30 plant phytochrome proteins used for multi-sequence alignment.
No. | Accession | Description | Latin name | Length (aa) |
1 | AAF66603 | phytochrome C | Oryza sativa Indica Group | 1137 |
2 | AAP06790 | phytochrome C1 apoprotein | Zea mays | 1135 |
3 | AAR33026 | phytochrome C | Sorghum bicolor subsp. Verticilliflorum | 1135 |
4 | AAU06208 | phytochrome C | Triticum aestivum | 1139 |
5 | ABA46868 | phytochrome A | Solanum tuberosum | 1123 |
6 | ABB13327 | phytochrome C | Hordeum vulgare subsp. vulgare | 1139 |
7 | ACC60969 | phytochrome A | Vitis riparia | 1124 |
8 | ACC60971 | phytochrome C | Vitis riparia | 1123 |
9 | ACC60972 | phytochrome E | Vitis riparia | 1124 |
10 | AEA50880 | phytochrome A | Populus tremula | 958 |
11 | AEK26583 | phytochrome A | Populus tremula | 1109 |
12 | BAA99410 | phytochrome A | Armoracia rusticana | 1122 |
13 | BAH79238 | phytochrome A | Cardamine nipponica | 1122 |
14 | BAH79243 | phytochrome A | Cardamine resedifolia | 1122 |
15 | BAM67032 | phytochrome A | Chrysanthemum seticuspe f. boreale | 1121 |
16 | BAN14712 | phytochrome E | Lotus japonicas | 943 |
17 | P33530 | Phytochrome A1 | Nicotiana tabacum | 1124 |
18 | P55004 | Phytochrome E | Ipomoea nil | 1115 |
19 | XP_002271671 | PREDICTED: phytochrome E | Vitis vinifera | 1124 |
20 | XP_002512596 | phytochrome A | Ricinus communis | 1124 |
21 | XP_002519749 | phytochrome B | Ricinus communis | 1131 |
22 | XP_003535030 | PREDICTED: phytochrome E-like | Glycine max | 1120 |
23 | XP_003559446 | PREDICTED: phytochrome C-like | Brachypodium distachyon | 1140 |
24 | XP_003595571 | Phytochrome E | Medicago truncatula | 1122 |
25 | XP_004144620 | PREDICTED: phytochrome C-like | Cucumis sativus | 1119 |
26 | XP_004147430 | PREDICTED: phytochrome E-like | Cucumis sativus | 1134 |
27 | XP_004232975 | PREDICTED: phytochrome E-like | Solanum lycopersicum | 1137 |
28 | XP_004243570 | PREDICTED: phytochrome C-like | Solanum lycopersicum | 1118 |
29 | XP_004295419 | PREDICTED: phytochrome E-like | Fragaria vesca subsp. vesca | 1162 |
30 | XP_004303565 | PREDICTED: phytochrome C-like | Fragaria vesca subsp. vesca | 1122 |
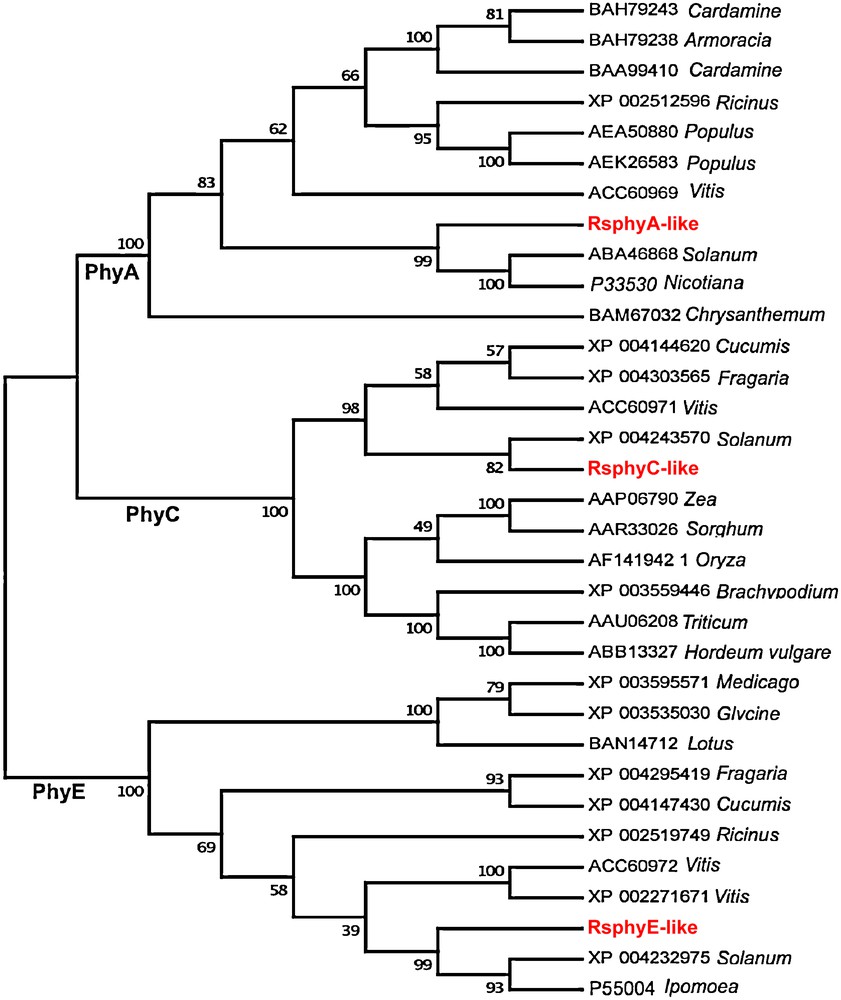
Phylogenetic analysis of 33 phytochromes including those of R. stricta phytochrome proteins. Color online.
4 Discussion
Using local BLASTn searches, three phy-like genes (i.e., RsphyA-, RsphyC- and RsphyD-like) were identified in Rhazya stricta. By applying more sensitive protein search tools, such as GeneMark.hmm, it was possible to identify the start and stop codons as well as the exon and intron boundaries of phy-like homologues in R. stricta (Fig. 1). Domain searches confirmed that both the C-terminal and N-terminal domains are conserved for all three Rhazya phy-like genes (Fig. 2). phyA and phyB proteins have been shown to be the primary mediators of the phytochrome-mediated development (reviewed in Mathews [41]).
The fact that phyB gene was not detected in R. stricta could be the result of the protein sequence being too divergent from Arabidopsis to be detected by BLAST. This explanation seems unlikely because of the high amino acid sequence identity between the three detected phytochrome genes of Rhazya and other angiosperms (96–99%). Alternatively, phyB protein may be absent from Rhazya and one of the other subunits has taken over its function. Rhazya stricta grows in extremely high light intensities in the deserts of western Asia and these conditions may have affected the evolutionary history of phytochrome genes in this plant species. Earlier studies of single and double mutants for phyA and phyB genes have demonstrated redundancy of function between both genes [42], supporting the hypothesis that that phyA may have taken over the function of phyB in Rhazya. Other studies on transcriptional profiling of etiolated phyB mutants subjected to red (R) wavelengths showed similar response to that of the wild-type controls [43,44]. These results suggest that other phytochrome family member(s) may be predominantly responsible for perception and transduction of R light in the absence of phyB protein. Recently, phyA protein was shown to play a dominant role in regulating rapid gene expression responses to R light [45]. These and other more recent studies indicate a shuttle role for phyA protein in response to R light, which is normally masked in the presence of phyB [46]. Thus, we propose that absence of phyB gene in R. stricta has resulted in RsphyA taking over the role of phyB.
Phytochromes play both unique and overlapping roles across the life stages of plants to regulate a range of developmental processes especially at seed germination and seedling stages. The latter stage is known for accumulating the higher levels of alkaloids useful for different pharmaceutical purposes in another medicinally important plant Pinellia ternata (Araceae) [47]. The present study provides valuable information for future manipulations of phytochrome gene expression at the seedling stage to enhance resources for better understanding alkaloids and their potential medicinal/pharmaceutical use in Rhazya stricta.
Disclosure of interest
The authors declare that they have no conflicts of interest concerning this article.
Acknowledgments
The authors gratefully acknowledge the financial support from King Abdulaziz University (KAU) Vice President for Educational Affairs Prof. Dr. Abdulrahman O. Alyoubi and the KAU Deanship of Scientific Research, Jeddah, Saudi Arabia, represented by the Unit of Strategic Technologies Research through the Project number 431/008-D for the Project entitled: “Environmental Meta-Genomics and Biotechnology of Rhazya stricta and its Associated Microbiota”.