1 Introduction
Dispersal is a frequent process engaged by almost all species, animals and plants alike. It has strong evolutionary and ecological implications on the spatial structure of meta-populations [1,2], particularly via gene flow in a context of habitat fragmentation [3–7]. The effects of dispersal are thus important for fauna and flora for a variety of reasons, preventing population homogenization, and reducing inbreeding, intraspecific competition and predation. Dispersal has also been defined as a “bet-hedging” strategy, or risk spreading, allowing individuals to move out of unstable environments [8–16] or habitats of marginal quality.
In addition to ground dispersal, some spider species use aerial dispersal strategies commonly called “ballooning” [17–19]. In addition to araneomorpha spiders, other Arachnids, trap-door spiders (Mygalomorpha) and acarid juveniles (e.g., Ixodidae and Tetranychidae) disperse by flight [14,20]. Ballooning has been defined as a passive dispersal enabled by the emission of a silk thread and subsequent transport in air currents [14,21,22] during which individuals lack control of direction, speed, and distance [21,23,24], contributing to the so-called “aerial plankton” [15,24,25]. Ballooning is divided into three phases: the ascent to a high point of the vegetation, the adoption of a specific body posture (“tiptoe” behaviour) and rising into the air [14,23,24].
Previous ballooning studies have focused on species initiation of pre-dispersal behaviour and on the effect of meteorological factors, but this research was primarily conducted under laboratory conditions. Juveniles are supposed to display this behaviour more frequently, mainly because of physiological conditions undergone during early development [26,27]. The weight of the individuals also plays an essential role [21,24], as climbing-up and dispersing in the air become more difficult concomitant to weight increase [18,28]. Moreover, stenotopic species, i.e. narrowly dependent on a habitat type, are less inclined to perform ballooning, in contrast to those who settle a variety of habitats (eurytopic species) [21]. Costs of dispersal are indeed higher for stenotopic species, because of reduced chance for dispersal success. Ballooning is especially important for survival in fragmented and/or disturbed habitats [21,30], such as agroecosystems [8,11,13,29,31–33]. Ballooning also plays a key role in limiting within-populations inbreeding, and promotes gene flow by dispersal to genetically different populations. Furthermore, dispersal allows spiders to prevent intraspecific competition rising from over-population [11,13,19,27,34].
Besides the endogenous parameters and those related to habitat characteristics, meteorological factors, particularly wind speed and air temperature [23,35–38], are believed to play an important role in the initiation of ballooning. A wind speed threshold beyond 3 m·s−1, or even 6 m·s−1, is expected to compromise dispersal, as showed under laboratory conditions [15,21,23,35,36,38]. High temperatures and precipitation are also likely to negatively affect dispersal by modification of air currents; the important influence of these factors increases as conditions become more extreme [15,19,21–23,39]. Here, we aim at increasing the knowledge on the determinants of spider ballooning in an agricultural landscape by conducting field experiments utilizing a 12-m high suction trap [24] and testing several hypotheses related to the timing of ballooning events. First, we expect the existence of several ballooning peaks during the course of a year, including a main one in the summer [33]. Considering the life cycle of most spider species [40], we also predict that dispersal phenology will differ between adults and immature individuals [33]. The predominant disperser is expected to be Linyphiidae because it is the most diversified and abundant family in temperate regions of the Northern Hemisphere [11,19] and because linyphiids are particularly abundant in meadows and agroecosystems [8,11,13,14,26,27,29]. Finally, we expect a difference between adults, juveniles and sex ratio abundances among families [22], because of differences in mean weight between life history stages and sex.
2 Methods
2.1 Study site and sampling design
A Rothamsted Insect Survey suction trap was employed to study spider dispersal in Brittany, western France (48°6′44.82″N 1°46′51.16″O) in an agricultural landscape consisting of crops, hedgerows, woody areas and human infrastructures (e.g., buildings, roads). The 30-cm-diameter trap, with a maximum power of inhalation of 40 m3·h−1, was placed at a height of 12.2 m. Samples were collected daily over the course of three years (from 1st January 2000 to the 31 December 2002). Three days (29 January and 9 May 2000, and 19 January 2001) were excluded because the trap failed to properly operate on the previous day.
2.2 Identification of spiders
Spiders were identified at the species level with a binocular magnifying glass (magnification up to 40×) using several complementary books [41,42] and Internet websites [43,44]. Adults were identified at the species level, whereas juveniles were identified at the family, genus or species level, using nomenclature from the 2016 World Spider Catalogue [45]. Some individuals (n = 141) were damaged and could not be properly identified (“doubtful” status).
2.3 Statistical analyses
To test the influence of meteorological factors on the aerial dispersal of spiders, daily meteorological parameters were obtained from data recorded by the meteorological station of Saint-Jacques-de-la-Lande (48°07″N 1°74″O), approximately 10 km away from the suction trap. The mean daily temperature (°C) corresponds to the mean between the lowest and the highest temperatures of each day. The daily mean wind speed (m·s−1) at 10 m high and the daily precipitation (mm) were also measured.
The daily abundance data were used to calculate: the total abundance (number of individuals per day and per ballooning peak), species richness, numbers of adults and immature individuals, and numbers of adult males and females. Dispersal peaks were graphed separately for adults and immature individuals. We defined the beginning and the end of a seasonal peak as the point at which the number of trapped individuals respectively soared and collapsed very highly between two successive days, regardless the year. Seasonal peaks were confirmed if, in designated as the time-period, over which at least 5% of the individuals trapped every year were caught within a period of 15 successive days.
The abundance and phenology of adults and immature individuals were compared using a Chi2 test. Unidentified individuals have not been taken into consideration (n = 212; 1.95%). They comprise spiders whom life history stage could not be precisely ascertained for some spiders (n = 183; 1.68%) and others which were in a too poorly conserved state to allow their identification (n = 141; 1.30%).
The predominance of one or several spider families was assessed using a Chi2 test. A second Chi2 test was conducted to analyse the distribution of the proportions of each life history stage and sex among families. Spider gender and species richness were only considered for adults, with unsexed adults excluded from analyses (n = 35; 0.79% of the adults). Families with less than five individuals per modality (adult or immature and male or female) were included in an “Other” category.
Finally, we studied the influence of meteorological factors on the total abundance of spiders, abundances of adults, immature individuals, males and females, and species richness. A generalized linear model (GLM) was created for each one of these response variables using the family tests “Quasi-Poisson”, since the data are relative to censuses [46], and also because data were over-dispersed (residual variance > degree of freedom). Each model included the three meteorological variables and their potential interactions. To test for the effects of these interactions, we used Anova and F-test. The most parsimonious model was determined by calculating Akaike Information Criterions (QAIC). The dredge() function was used from this model created on the “Poisson” family, informing the dispersion parameter “chat” relative to the “Quasi-Poisson” model. Linear regressions were created to demonstrate the variation of the responses in the final model. To show these interactions, we determined classes of low and high temperatures based on the exploratory analysis of the data variation along the temperature parameter, using 19 °C as a threshold cut-off for “low” and “high” temperature categories, respectively. All statistical analyses were conducted in R software (3.0.1, [47]), using the “MuMIn” package to calculate the QAIC. A threshold significance of α = 0.05 was applied for all tests.
3 Results
3.1 Phenology of dispersal
In total, 10,879 spiders were identified, including 4419 adults and 6248 immature individuals. The abundance per life history stage category strongly varied over the years (Figs. 1 and 2). Regardless of the life history stage, dispersal was more frequent over January and November, and was especially prevalent during four periods: from 6 to 21 January (peak 1), from 8 to 21 February (peak 2), from 13 May to 31 July (peak 3) and from 9 October to 3 December (peak 4). These peaks were characterized by a strong increase in the number of individuals caught, and persisted for several days or weeks. All years considered, ¾ of the adults and 2/3 of the immature individuals were caught during these four peaks.
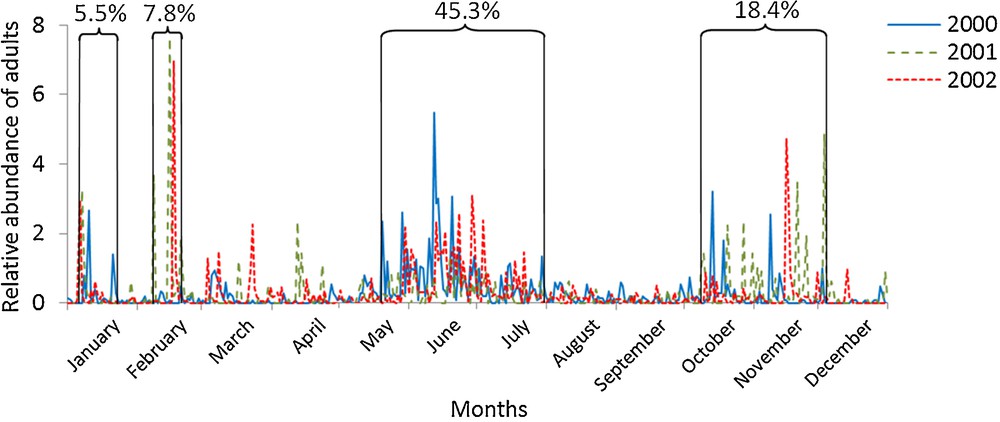
Daily variations in annual relative abundance of adult spiders (n = 4419) between 2000 and 2002.
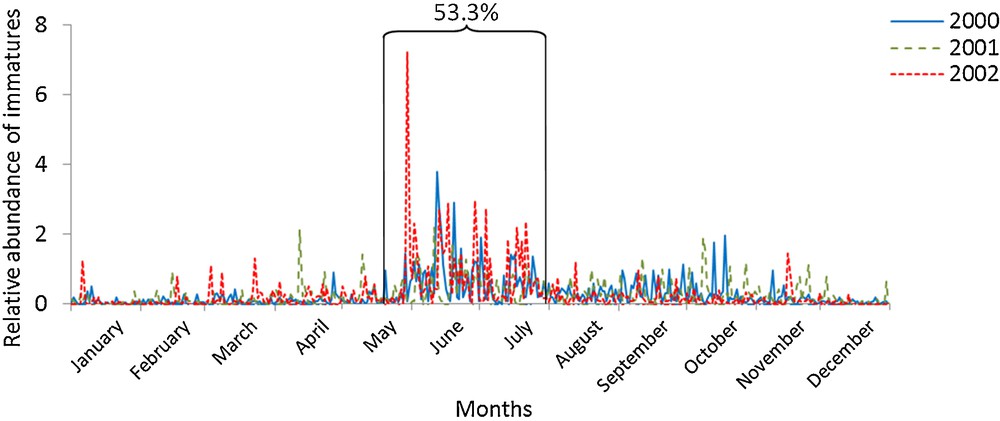
Daily variations in annual relative abundance of immature spiders (n = 6248) between 2000 and 2002.
Adults and immature individuals presented a distinct phenological pattern (Chi2 = 8.59, df = 3, P < 0.05). Regardless of the life history stage, summer represented the most important period of ballooning for both adults and immature individuals (45.3% and 53.3%, respectively) and persisted for the longest amount of time (2.5 months). The next most dominant peak was the autumn peak (18.4 and 10.9% respectively: ∼ 2 months), followed by two peaks at the beginning of the year (peak 1: 5.5 vs. 1.5%; peak 2: 7.8 vs. 1.4%). These last two peaks existed primarily for adults. Thus, immature individuals dispersed more than adults during non-peak times, and more gradually throughout the year (32.8% and 23.0% respectively), without presenting an obvious activity peak except during summer. The dispersal of adults was clearly spread throughout these four peaks and more equally among them throughout the seasons, in contrast to the juvenile peak that occurred during summer.
3.2 Taxa involved in ballooning
The 18 families we identified demonstrated statistical differences in abundance (Chi2 = 98,274.44, df = 17, P < 0.001). Linyphiidae was the predominant family (74.1% of the individuals), followed by Theridiidae and Araneidae, (8.8% and 7.2% respectively). Other families present in low numbers were: Philodromidae, Clubionidae, and Tetragnathidae (3.3; 2.0 and 2.0%). A few other families were also censused, but with less than 100 individuals (Appendix 1).
Concerning taxonomic diversity, 71 genera and 83 species were identified (Appendix 1). Linyphiidae was the most diversified family (44 taxa and 39 species). Among the 4419 classified adults, 3427, (77.55%), were represented by only seven species (Oedothorax fuscus, Tenuiphantes tenuis, Bathyphantes gracilis, Erigone atra, Oedothorax retusus, Erigone dentipalpis, and Robertus arundineti). Most of them are linyphids (71.55%), except R. arundineti (Theridiidae).
The relative abundance of each life history stage was clearly different between families (Chi2 = 285.43, df = 8, P < 0.001) (Fig. 3). Considering all families, the greatest difference between the proportions of adults to immature individuals was observed for the Tetragnathidae, which was comprised almost exclusively of adults of the genus Pachygnatha (P. degeeri and P. clercki). Linyphiidae and Dictynidae were also families having high proportions of adults relative to juveniles. At the opposite end of the spectrum, Araneidae, Clubionidae, Philodromidae, Salticidae, Theridiidae and “Other” families were mainly represented by immature individuals.
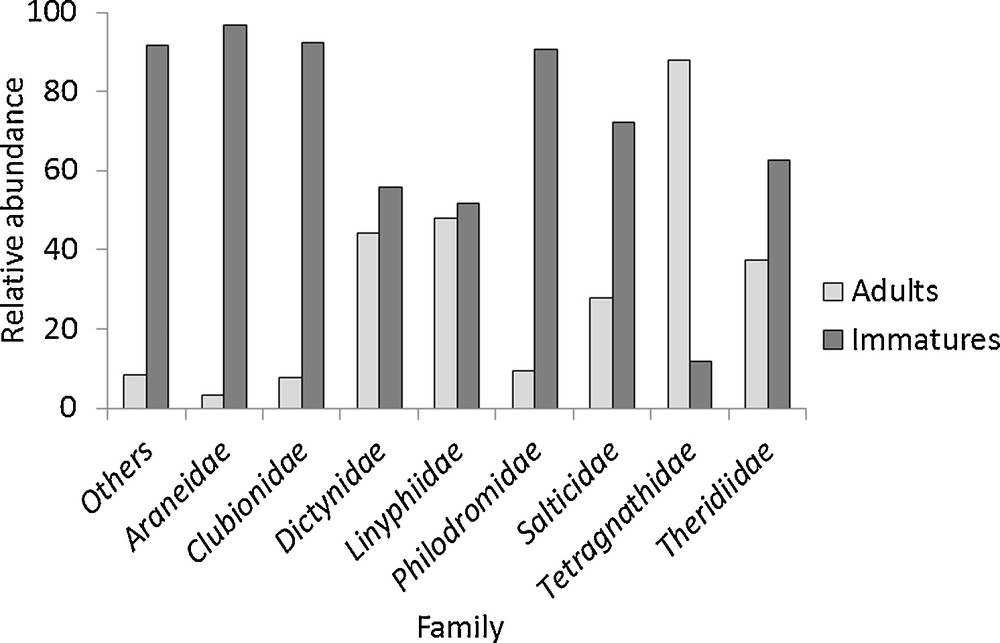
Relative abundance of adults and immature individuals in each family (n = 10,667) between 2000 and 2002.
Among the adults identified, 2010 males and 2374 females were counted. The proportion of each sex differed among families (Chi2 = 112.54, df = 7, P < 0.001) (Fig. 4). The highest proportion of males was observed in Araneidae, Clubionidae and Philodromidae, whereas proportionally more females were observed in Tetragnathidae and the “Other” families. The sex ratio was close to 1 for Linyphiidae, Dictynidae and Theridiidae.
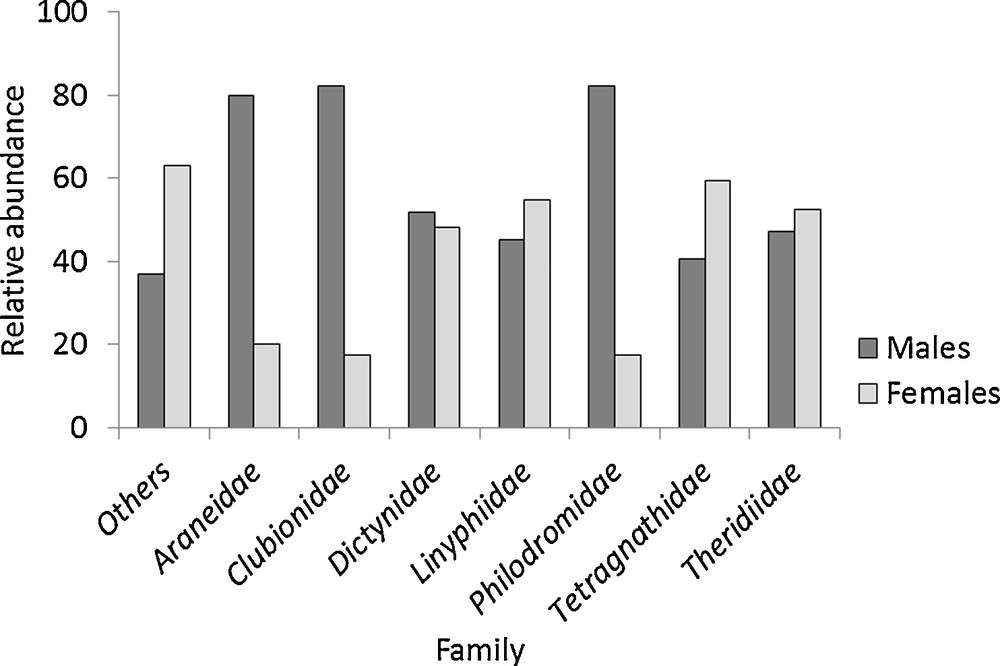
Relative abundance of adult males and females in each family (n = 4384) between 2000 and 2002.
3.3 Meteorological determinants of dispersal
The GLM on all response variables revealed an influence of the mean daily wind velocity and the mean daily temperature, as well as their interaction terms. Other models were significant, but less biologically relevant, and QAIC values were similar to those of the model we retained (Appendix 2).
The abundance of spiders was negatively influenced by the wind speed, regardless of the mean daily temperature (Fig. 5) [low temperatures (Tlow): P < 0.001, R2 = 0.129; high temperatures (Thigh): P < 0.010, R2 = 0.0658]. This effect is also noticeable on the abundance of adults (Tlow: P < 0.001, R2 = 0.114; Thigh: P < 0.010, R2 = 0.043), immature individuals (Tlow: P < 0.001, R2 = 0.098; Thigh: P < 0.010, R2 = 0.065), females (Tlow: P < 0.001, R2 = 0.092; Thigh: P < 0.001, R2 = 0.075) and males (Tlow: P < 0.001, R2 = 0.110; Thigh: P < 0.050, R2 = 0.036). Indeed, beyond a wind speed of 4 m·s−1, spider abundances dropped to 0. Nevertheless, temperatures greater than 19 °C seem to be slightly more suitable for ballooning, but this effect is weakened by increases in wind speed and disappears with wind speeds beyond 5.5 m·s−1. The interaction between wind speed and temperature was weakest in adults, and especially in females.
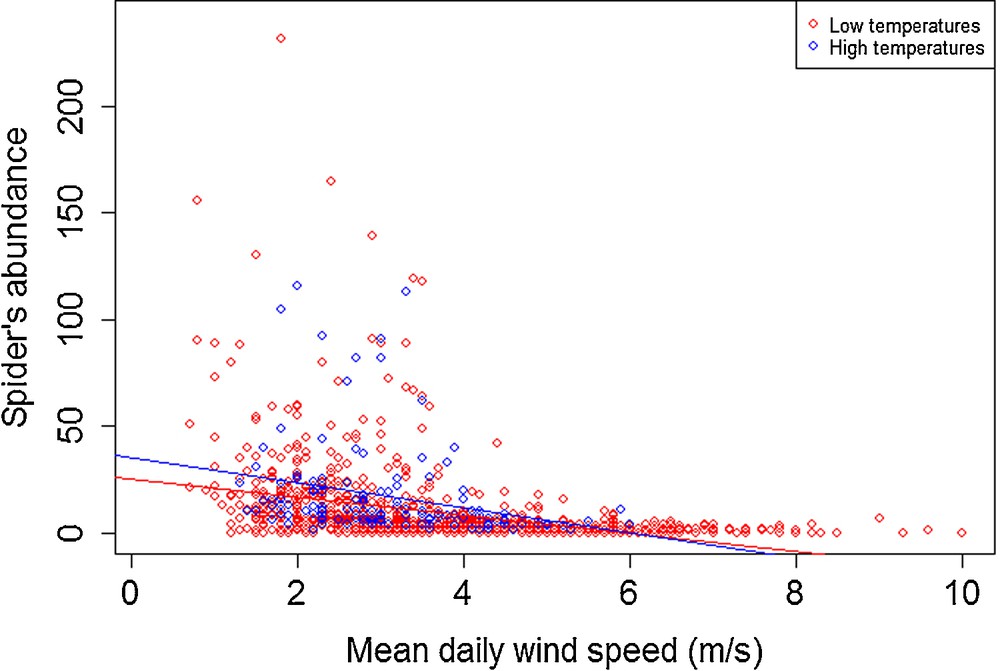
Modelled effect of an increased wind speed on total abundance of ballooners, under both low (< 19 °C) and high temperatures (> 19 °C).
Concerning species richness, wind had a negative influence, regardless of temperature (Fig. 6) (Tlow: P < 0.001, R2 = 0.227; Thigh: P < 0.001, R2 = 0.134). Similar to patterns observed for abundance, the species richness was greatest when temperatures exceeded 19 °C. The trend was reversed beyond 5.5 m·s−1, approximately. Indeed, no individual was trapped on days when the wind speed and the temperature exceeded 6 m·s−1 and 19 °C, respectively. Also similar to patterns observed for abundance, once the 4 m·s−1 wind speed threshold was reached, species richness consistently dropped to low values (less than 5).
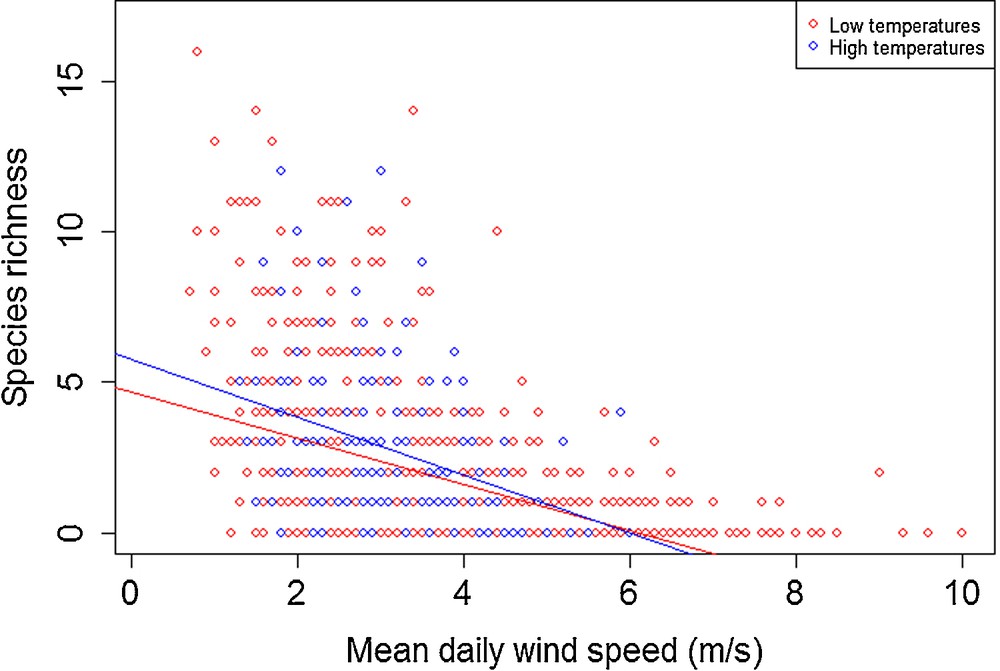
Modelled effect of an increased wind speed on spider species richness, under both low (< 19 °C) and high temperatures (> 19 °C).
4 Discussion
4.1 Phenology
In this three-year study, 10,879 spiders were sampled, providing a much richer dataset than previous studies that assessed spider dispersal by ballooning in an agricultural landscape [22,48]. The effectiveness of a suction trap placed at a height of 12.2 m in intercepting ballooning spiders at all life history stages is evidenced by our finding that approximately half of all of the trapped individuals were immature ones, including some Linyphiidae sp., Araneidae sp., Theridiidae sp. and Philodromus sp., as well as the undetermined spiders.
Ballooning was found to be more frequent in immature individuals, especially during the summer, in agreement with previous studies [18,26,27,33]. This can be mainly attributed to the physiological conditions immature individuals experience during their development or immediately after hatching from the egg bag. Ballooning could be stimulated by the lack of food realized after emergence, or inferred from development based on maternal condition and resource allocation to eggs [37,49]. Density dependence would play an important role in the commitment of ballooning by the immature individuals, in particular as this relates to starvation [50–52]. Juveniles mostly disperse to limit intraspecific competition [11,13,19,27]. Relative to adults, immature individuals are also usually smaller and lighter, making it easier for them to rise in the air and to disperse. Nevertheless, all the studies do not converge to the same conclusion [14,27,53]. Indeed, according to Bell et al. [14], the greater abundance of immature individuals would reflect more the fact that their density is greater and that they can rise higher, favouring their trapping and probability of detection. The predation risk may also be a major factor in the decision to disperse [27]. There is also certain plasticity in ballooning, including at the intra-population scale, especially related to the temperature during early development [37]. Indeed, exposure to higher temperatures promotes the decision to balloon [4,37]. Thus, according to the laying site a female chooses, she can influence the future behaviour of her offspring to balloon.
Spiders are likely capable of ballooning throughout the year because of their relatively small size, but they appear to prefer periods between January and November (e.g., [26,33]). Spider activity increases at the beginning of the seasonally warm weather, in particular that which is favourable for reproduction, during which the adults disperse to find a reproduction site, a mate and/or to disperse their offspring [22,33]. Thus, the most precocious species disperse from February. The summer period corresponds to the reproduction period of most of spiders, hence the high proportion of adult ballooners likely seeking a mate or a favourable location to lay their egg bag [22]. The proportion of immature individuals in the summer period, with peaks in activity from June and continuing until November, suggests that ballooning is important to prevent starvation, avoid predation risk, and to reduce intraspecific competition [11,13,19,27,37,49–52]. The autumn peak corresponds to the search for a wintering site, both for adults and immature individuals [13,15,22,54]. This allows them to survive in winter conditions and to increase their chance of reproduction the following year [33]. Ballooning is thus more important in summer and autumn relative to other time-periods [33]. The intensity and the frequency of dispersal peaks vary according to the biology and phenology of the species. As an example, Erigone atra is active throughout the year, but its activity decreases between the end of May and the end of November [27]. Another linyphiid species, Agyneta rurestris, disperses mostly from the end of July through mid-October [26]. This could explain the existence of the two first peaks in adults that we observed, given the abundance of linyphiid specimens in our sample.
Thus, the immature individuals would have an activity more unimodal, whereas the adults would be more plurimodal [33]. Alternatively, because the suction trap is located in an agricultural landscape, the spiders’ experience perturbations associated with farming practices (e.g., pesticide application, fragmentation, rotation, crop harvest) known to have negative repercussions on spider populations [8,11,13,29,55]. Therefore, the adults’ plurimodal behaviour could be a response to the instability of the habitat [32,33] and seasonal disruptions related to agricultural practices. That could also explain more largely the dispersal out of the peaks for 23.0% of the adults and 32.8% of the immature individuals.
4.2 Taxa involved in ballooning
Many families and species recorded by Blandenier [22] were detected in this study. Nevertheless, six families (Mimetidae, Oxyopidae, Pholcidae, Pisauridae, Segestridae, and Zoridae) were not censused by Blandenier [22]. Few Pholcids have been reported to disperse by ballooning, except for one tropical genus [14]. There are four species of Pholcids spiders in western France: Holocnemus pulchei, Pholcus phalangioides, Psilochorus simony, and Spermophora senoculata. All of them are species associated with human buildings in the area and none of them are known to disperse by ballooning. Pholcus phalangioides, the most common of them in our region, therefore the most likely species to be trapped, seems to disperse by passive transport rather than by ballooning [56]. So, our probable first documented occurrence of an immature of this species in a suction trap does not confirm any aerial dispersal behaviour; however, future studies are needed to assess this life history strategy for dispersal. Globally, 58 new genera and species are recorded here for the first time as having balloon strategies of dispersal (Appendix 1). For instance, Araniella inconspicua, Lathys sexpustulata, and Clubiona leucaspis were encountered in the suction trap. Moreover, the suction trap yielded new locational records for Trematocephalus cristatus and A. inconspicua, the sixth and fifth observations in the Armorican Massif and the first and second in the Ille-et-Vilaine department [7,57]. These are little known species because of their tree-living habitats (respectively in the canopy and under barks) [58]. This is also only the ninth observation for C. leucaspis [57], a tree-dwelling species. The classic trapping methods (Pitfall trap, etc.) are not well suited for detecting these species, whereas the suction trap provides more information about area occupancy. We also noticed the dominance of some species, already known to balloon (e.g., Oedothorax fuscus, Tenuiphantes tenuis, Erigone atra, Pachygnatha degeeri) [4,15,22,27]. Indeed, these species are common in the study site examined here, and they are widespread in the Armorican Massif [57].
The frequency of ballooning strongly varies among families [37,59]. Linyphiids are the dominant species, in accordance with the fact that they are the most species rich family in temperate regions of the Northern Hemisphere [11,13,19]. Their success can be attributed to their ability to store sperm, which allows the females to lay several eggs bags, and maximizes their reproductive rate [9]. This process is one that defines the “bet-hedging” strategy, or risk spreading [8–16]. On the other hand, linyphiids are very abundant in meadows and crop fields, habitats favourable to ballooning because of perturbations associated with agriculture [6,14,22,26]. Linyphiids are also natural predators of insects pests like aphids [8,11,60], which brings a particular interest to their conservation in the context of agricultural practices and for ecosystem services. Moreover, global climate change could have profound consequences given our finding of spider's reliance on favourable winds to promote dispersal.
The relative abundance of each stage was also different among families. Among linyphiids, dispersal of adults and immature individuals is facilitated by their small size [22,26]. Indeed, the ballooners we observed did not weigh more than 2.0 mg in average, a mass not reached by adult linyphiids [18,28]. This threshold can be explained by three factors: the loss of energy during climbing-up, the important risk of predation while in the air, and the decrease of the number of available sites to perform ballooning [27]. Accordingly, the ballooners’ weight is typically between 0.2 and 1.0 mg, and rarely exceeds 2.0 mg [18,28]. The adults of Dictynidae are also quite small, which explains, as previously, the similar proportions of ballooning adults and immature individuals. Within the other families (Araneidae, Clubionidae, Philodromidae, Salticidae, Theridiidae and “Other”), immature individuals represented the majority of trapped individuals, likely because the ability of adults to successfully balloon was constrained by size [42]. Contrary to other Tetragnathids species, Pachygnatha spp. are small and therefore can disperse at the adult stage, similar to linyphiids and theriidids, hence a high proportion of adults was observed among samples represented by this family [42].
Gender-specific differences in size and reproductive strategies have important consequences for dispersal. In this study, weight constraints were evident from observed differences in sex ratios among families known for dimorphisms [27]. The highest proportions of males was observed for Araneidae, Clubionidae and Philodromidae, where the sexual dimorphism is particularly high and favours the dispersal of males, the smaller and lighter sex [42]. This dimorphism is explained particularly by the dependence of each sex on food resources and microclimate, mostly during the reproduction and egg production periods [11,49,61]. Females, with larger body sizes than males and greater need for nutrition to maximize reproductive output, may be willing to take greater risks than males. Thus, ballooning is strongly related to the reproductive state of an individual. The fertilized females are expected to disperse from low-quality habitat patches in search of better quality habitat to meet their higher nutritional needs [13]. The females would be searching for laying sites to disperse their offspring and to limit intraspecific competition and inbreeding [11,13,19,27]. Therefore, females are predicted to take risks to increase the chances of their breeding success [14,33], while males require less food than females and disperse mostly to mate [11,13,19,21].
4.3 Meteorological factors
The important role of meteorological conditions on dispersal is highlighted by our finding that both wind, and to a lesser extent temperature, have strong influence on dispersal. Indeed, there is a wind speed threshold beyond which the dispersal seems to be compromised (∼ 4 m·s−1) and a second threshold beyond which it seems to be impossible (∼ 5.5 m·s−1), regardless of temperature. Below wind speeds of ∼ 4 m·s−1, dispersal is favoured, as measured in this study by both abundance and species richness. In a previous laboratory experiment [58], a threshold of 3 m·s−1 was found and later confirmed by other authors [21,23,35,36,38]. Another threshold of 6 m·s−1 was demonstrated at a height of 10 m [15], which is closer to the value that we found. Thus, wind speed is a key parameter for spiders to initiate ballooning: the individuals’ climb-up is compromised beyond 4 m·s−1, and wind speeds above 5.5 m·s−1 makes ballooning nearly impossible.
With regards to temperature, our findings are consistent with previous research that indicate litter temperature are key determinants of dispersal intensity [15,19,23,39,54,56,62,63]. We found that low temperatures (< 19 °C) decreases dispersal probability, while higher temperatures equate to higher dispersal probabilities and explain the almost systematic dispersal during “hot” days (> 19 °C). On the contrary, ballooning did not systematically occur on “cold” days, although dispersal was still possible at 0 °C and even lower, which is consistent with previous studies [39] and informs others [27,59]. Some authors also showed that beyond extreme temperature values, as those known during the 2003 heat wave in Western Europe, dispersal is no longer possible, likely because air currents are modified [39]. In our case, the mean temperature did not exceed 26.5 °C, therefore the temperature threshold observed by [39] was not reached. Ballooning is obviously less frequent in winter because of the reduction of the climbing-up air currents required for spiders to rise in the airs, but it is still possible (this study, [22]). High temperatures in late summer can explain the late peaks of ballooning, particularly observed for immature individuals in September [19,21].
This study informs phenological characterization, increases our understanding of the effects of the meteorological factors on ballooning, and fills in gaps for which principal species and families disperse by ballooning. Yet, the influence of other parameters, such as air pressure, wind direction, mean temperature 20 cm underground, relative humidity, solar exposure, but also the difference of temperature between two successive days would be worthwhile to consider [14,19,23,24,35,37,38,48,59]. Indeed, from a spider standpoint, hot and windless days following a cool night would create optimal conditions for the formation of air currents suitable for ballooning [14,19]. A sharp change in temperatures in the morning could also initiate spider dispersal [19,23,35,37,38,59]. The phenology and the response to climatic factors would certainly be different between the open (e.g., agroecosystem) and closed habitats (e.g., forest) [33]. Thus, in a closed habitat, humidity may play an important role [33]. Additionally, this study was based on daily mean meteorological conditions, which mask daily variations. Measures taken at a different step of time (hours or day vs. night for instance) might reveal other effects, and particularly that of precipitation [15]. Another consideration for our findings is that wind velocity was not measured close to the trap, but at a distance of 10 km. Important fluctuations in meteorology across space and over time, and between the trap and meteorological station, may bias our results. We can also expect the suction trap to operate suboptimally during days with extreme wind speeds. Thus, the number of trapped individuals during high wind speed conditions may be negatively biased. High wind velocity may be the reason why the suction trap did not properly operate for three days.
We measured parameters at a height of 10 m, which corresponds to the initiation of the dispersal, whereas the effect of wind speed may be different according to where it is measured, on the ground (initiation of ballooning) or at height (pursuing of ballooning) [23,59]. Given that, spiders are able to balloon for tens of kilometres [14,27]; it is possible that individuals trapped during this study do not originate from the Le Rheu agroecosystem site, which could reduce the predictive power of locally measured explanatory variables.
To summarize, we found that peak timing of ballooning by adult and immature spiders is a consequence of several biotic and abiotic factors, including meteorological conditions. Here, a low wind speed associated with high temperature was shown to favour the dispersal of spiders, but these conditions are not necessary [21,23]. A spider's response to an increase in wind speed and to a decrease in temperature would be a behavioural reduction in dispersal attempts [14,23]. In case of strong winds, they tend to avoid displaying any pre-dispersal behaviour [23,64,65], hence decreasing the abundance and the species richness of ballooning spiders. This study also enabled us to better understand how dispersal is shaped by local factors over time in an agricultural landscape, providing a solid base for further research on agro-ecology and biocontrol using spiders [39,66].
Disclosure of interest
The authors declare that they have no competing interest.
Acknowledgments
We would like to thank Maurice Hullé and Christelle Buchard of French National Institute for Agricultural Research (INRA) for providing and sorting the samples, Maxime Hervé for help in R package, and M. Renee Bellinger and one anonymous referee for useful comments on earlier drafts. We also acknowledge the GRETIA (Armorican Entomological Society) and Aphiweb for providing the funding necessary to run this study and Météo-France for providing access to the meteorological data.