1. Introduction
Proteins largely determine the way cells work and perform their functions. The amount of mRNA and how efficiently it is translated into proteins are tightly regulated, ensuring that proteins are produced at the right time and place within the cell and between different cell types [1, 2, 3]. Research has shown that mRNA can be found in specific areas within a cell, where it may be directly translated into proteins [4, 5, 6]. This configuration enables cells to promptly and accurately modify their proteome without requiring modifications in transcription levels.
The recent advancement in live imaging of translation provides a dynamic perspective of protein synthesis in real-time within living cells, contrasting with fixed methods that offer only a static snapshot at a single time point. Translation imaging can regroup several different approaches (reviewed in [7]). In this perspective, we focus on the antibody-based detection of nascent peptides for visualizing single molecule translation in vivo. We discuss how spatio-temporal imaging advances our understanding of biological processes in developing organisms. We discuss technological advancements that could deepen our grasp of translation mechanisms and review some contexts in which this method has led to scientific discoveries and its potential to drive future breakthroughs.
Imaging translation through antibody recognition of nascent peptides involves a two-component system: a tag, which is typically multimerized (from 5 to 56 times [8, 9] but often 24 or 32 times), and inserted into the gene of interest; and a genetically encoded detector made of either a single-chain antibody (scFv) or a nanobody fused to a fluorescent protein (FP) [10, 11, 12]. Upon translation, the repeats of the peptide tag will be bound by the detector, resulting in bright signal spots that stand out against the background. This is because a single mRNA molecule will bear FPs bound to the multiple copies of the tag, but also because there will be numerous nascent peptides for each mRNA molecule, as it is translated by multiple ribosomes (Figure 1). The translation dots, or polysomes, may then be tracked and their fluorescence measured to extract dynamics parameters such as diffusion, translation speed, etc [9, 13, 14, 15, 16]. This method is the only one that enables to capture parameters mentioned above while bearing in mind that it requires the insertion of an array of tags in frame with the gene of interest. These tags could significantly increase the size of the reporter gene, and the values measured should not be considered absolute values but an approximation of the translation dynamics of the gene under study.
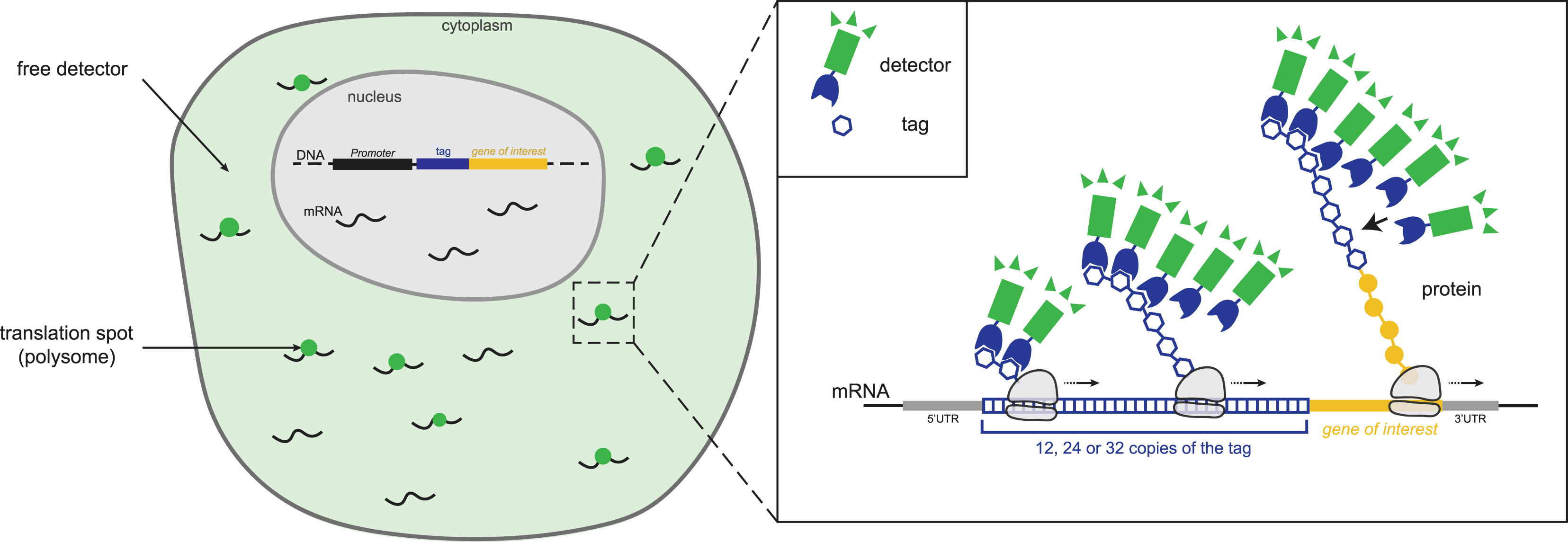
Nascent peptide labeling for live imaging of translation. On the left, schematic of a cell expressing a reporter construct with multiple tags, a gene of interest and the fluorescent detector freely diffusing in the cell. Once translated, the tag peptides are bound by the fluorescent detector (right panel) and create a bright signal in the cell.
Live imaging of translation using this approach has been possible since 2016 in cultured cells [9, 13, 14, 15, 16]. In these studies, the authors use two different systems, the SunTag and the Spaghetti Monster. The SunTag system consists of multiple copies of a small peptide, derived from the GCN4 protein, inserted at the N-terminus of the protein of interest and a genetically encoded anti-GCN4 scFv fused to a fluorescent protein [9, 13, 14, 15]. The Spaghetti Monster system is based on a repetition of several FLAG tags inserted after the start codon and recognized by a fluorescently labeled anti-FLAG Fab introduced into cells by bead loading [14]. In 2021, the SunTag system was implemented in a multicellular organism [17, 18, 19] and was followed by multiple studies that were more and more resolutive in time and space [8, 20, 21, 22, 23, 24, 25]. These studies went down to the single molecule level and have shown various examples where translation can be heterogeneous within the cell, adding another layer of regulation to that of mRNA localization and expression level.
Several studies have shown evidence for the localized dynamic translation of mRNA. In Dufourt et al. [17], the authors used the CRISPR/Cas9 system to tag the twist gene, encoding a key transcription factor involved in the epithelial-to-mesenchymal transition, at its endogenous locus in Drosophila embryos using the SunTag system. They found that the translation dynamics can be heterogeneous within cells. In another study, Chen and colleagues [26] showed in the Drosophila embryo that ribonucleoprotein (RNP) granules regulate localized protein synthesis through compartmentalized relief of translational repression.
This finding implies that during the development of an embryo, one needs to look at the precise regulation of translation locally, and during time. Further investigation will be required to identify in which exact biological processes this is relevant.
These in vivo studies, as well as many others in mammalian cells, have shed light on new spatial and temporal regulatory mechanisms that cells employ for post-transcriptional gene expression control, such as local translation [27, 28], IRES/Cap translation [25], bursting of translation [29], RNP granule [18, 26], polysome transport [30], translation kinetics [31], and coordination of translation and degradation [20, 32].
2. Technology improvements
In the past years, the range of tools used to image translation has advanced considerably [33]. Recently, Bellec et al. [11] developed and characterized new tools to image translation in a living Drosophila embryo. This study also revealed that imaging translation in vivo is not that trivial and that it has limitations and requires optimization. In the meantime, these new tools are powerful and will lead us to see what we couldn’t access before.
Looking forward, there are several technical areas requiring further development. First, there is a need for advancements in mRNA tracking. Since translation of proteins can take several minutes, continuous tracking of mRNA for tens of minutes or even hours is essential to comprehend translation regulation at the single mRNA level. Bright and stable RNA and antibody labeling techniques are imperative for this purpose; the use of fluorogenic protein tags, such as SNAP-Tag, CLIP-Tag, or HaloTag, could provide some advantages over FP, such as high brightness and better photo-stability. However, this would require injection of the dyes or harsh treatment in organisms because of their lack of permeability (in contrast to cell lines). Moreover, in contrary to FPs which replenish the pool of antibodies constantly, during time-lapse experiments, fluorogenic protein tags require constant feeding of dyes potentially leading to toxicity/lethality. One of the potential future advances would be the development of genetically encoded Quenchbodies which would fluoresce only when bound to their target [34], combined with ultra-bright and stable FP such as monomeric StayGold [35]. Second, it is essential to improve in vivo microscopy techniques. The visualization of freely diffusing mRNAs in cells and tissues continues to pose challenges, especially in a living organism. Improvements in imaging technologies, such as lattice light sheet microscopy or adaptive optics, hold the potential to enable single mRNA imaging in live animals thanks to their fast acquisition, large field of view, and good resolution [36]. However, the rapid increase in the volume of microscopy data is outpacing computer capabilities, and this issue needs to be addressed in order to extract all the information encoded in the heavy imaging data.
Third, it is crucial to establish robust quantitative mathematical models. The first modeling used to fit translation imaging data derived from the theory implemented for transcription by RNA polymerase II [37, 38], using many assumptions. Now, modeling of translation dynamics should be improved to distinguish between different scenarios (such as initiation rate bursting, non-homogeneous ribosome coverage, ribosome collisions, ribosome slowdown, ribosome release delay, etc.), as it is starting to be implemented for transcription [39, 40, 41, 42, 43]. Recently, the laboratory of B. Wu improved these measurements for the analysis of translation bursting [29]. Furthermore, the accuracy of these mathematical models depends on the measurement methods used, making it crucial to employ multiple approaches for a more precise assessment of translation kinetics (reviewed in [31, 44]). Kinetic parameters of translation can be measured using Fluorescence Recovery After Photobleaching (FRAP) [9, 15], but this method faces challenges in developing organisms due to the rapid polysome movements, especially when studying translation in its native context (except for mRNA naturally associated to immobile organelles [26]). Other approaches exist, such as Ribosome Run-Off Assay, in which the decrease in fluorescence is measured, corresponding to the time needed for the ribosome to translate the entire mRNA, using naturally occurring translation bursts or drug treatments such as harringtonine, an inhibitor of translation initiation [13]. This method also faces challenges in developing organisms. First, like with FRAP experiments, it requires a long tracking of polysomes, and second, it faces the lack of permeability of developing organisms compared to cells in culture.
One of the most convenient ways to measure translation dynamics in living organisms is by analyzing the fluorescence fluctuation of the polysomes. This technique faces numerous challenges and is highly sensitive to experimental noise (e.g., photobleaching, imaging focusing issues). One of the next steps would be to generate a common theoretical framework to improve the analysis of biological measurements and to generate robust and reproducible results, with variations reflecting only the biological context but not the way it is analyzed. This improvement is even more important for developing organisms, where it is challenging to apply specific tricks used in cell culture to improve translation dynamics measurements as developed in M. Tanenbaum’s laboratory [45, 46].
Lastly, the development of tools to perturb the translation of a specific gene will be key to understanding the role of fine-tuning translation, without affecting the overall translation. Optogenetic techniques would allow the spatial and temporal control of translation using, for example, light-induced aggregation of mRNA [47] or recruitment of light-sensitive RNA binding protein (RBP) to block translation [48] (Figure 2). These tools will enable us to determine when and where the translation of a specific gene is required, and to observe the resulting phenotypes that could potentially be linked to some diseases. Moreover, the integration of single-molecule translation measurements with ensemble protein output, such as ribosome profiling and proteomics experiments, will surely enhance our understanding of the protein life cycle.

Optogenetic tools to control translation. On the left, a schematic representing mRNA in translation (mRNA in black and ribosomes in grey) bound by a light sensitive protein (orange). Upon light exposure, RNA-protein complexes will multimerize and trigger clusterization of the mRNAs bound to it, which will result in eviction of ribosome and inhibition of translation. On the right, a second system with mRNAs 5′ tagged with an aptamer (loop). Upon light exposure, an RBP (yellow) will change its conformation and bind the loop, resulting in inhibition of de novo translation of target mRNAs. Masquer
Optogenetic tools to control translation. On the left, a schematic representing mRNA in translation (mRNA in black and ribosomes in grey) bound by a light sensitive protein (orange). Upon light exposure, RNA-protein complexes will multimerize and trigger clusterization of the ... Lire la suite
3. Future outlooks
Over the past decade, considerable progress have been made to image translation in vivo and at the single molecule level. Currently, we possess a toolkit to observe the translation of endogenously tagged mRNA at the single mRNA level, in living cells and developing organisms.
Investigating the contribution of translation regulation in developmental regulation would represent a significant advance, given that we are beginning to understand that translation is not a simple protein synthesis mechanism, as it has also emerged previously for transcription thanks to imaging using the MS2/MCP system [49]. Yet, many key biological questions remain unsolved, such as: how do cells selectively translate their transcriptome to drive their fate? How dynamic is translation during development and cell differentiation? Are there different “modes” of translation for the same mRNA depending on the context? This level of understanding of biological processes could lead to breakthroughs in developmental biology, enabling us to reinvestigate and better characterize previously described biological concepts. As a first step, it would be interesting to revisit the translation activation of localized mRNA using these tools. Several studies suggest that mRNA localization is one of the main drivers of localized translation, for example in morphogens gradient formation [50, 51, 52, 53], mRNA polarization in intestinal epithelium [54], as well as in neuronal signaling [55] and development [56, 57]. These tools will also enable researchers to better understand how translation regulation is precisely defined in the absence of transcription, such as before the maternal-to-zygotic transition when the development relies only on deposited mRNA and proteins [58], and during erythropoiesis [59, 60]. Indeed, mature erythroid cells lack nuclei and therefore rely on a balanced translation of the globin chain proteins. Red blood cells can rapidly adapt to environmental changes and organismal needs, using various mechanisms that rely on translation regulation [60].
Another interesting perspective proposed by Chen et al. [61] and Boersma et al. [62] is the use of the SunTag system to study the translation dynamics of viruses in cell culture. These studies open the door to a completely new area of investigation into the replication cycle dynamics of viruses during infection, at the single molecule level, and it has yet to be implemented in living organisms to understand viral replication and translation within an organismal context and across different cell types. Last but not least, the role of translation dynamics in the robustness of developmental processes is poorly understood. For instance, gene expression compensation at the translation level has been reported in the context of aneuploidy [63], but we still lack understanding of how, when, and where this translation compensation is triggered. Another interesting context is the oocyte and early embryonic development, where dynamic and oscillatory patterns of translation have been described genome-wide, particularly during the cell cycle [64, 65]. However, how this is regulated at the single cell level and how it can control patterning and cell fate decisions remains to be elucidated. Finally, this approach would be beneficial for understanding diseases such as ribosomopathies, a group of disorders that are not fully understood, caused by disrupted ribosome biogenesis and/or diminished function [66]. It could also be useful for neurodegenerative diseases, including amyotrophic lateral sclerosis [67, 68]. Imaging translation in this context would help us to better understand the disease (e.g., which mutation affects which aspect of translation dynamics?) in order to improve the treatment of patients.
Declaration of interests
The authors do not work for, advise, own shares in, or receive funds from any organization that could benefit from this article, and have declared no affiliations other than their research organizations.
Author contributions
JD and MB wrote the manuscript.
Funding
This work was supported by the HFSP long-term fellowship to MB, JD is sponsored by CNRS.
Acknowledgments
We acknowledge D. Stainier and D. Muriaux for their support during the manuscript preparation. We thank the Académie des sciences for awarding MB with the Madeleine-Lecoq Prize.